Abstract
The interaction of polymeric nanoparticles formulated from the biodegradable polymer poly(DL-lactide-co-glycolide) loaded with bacteriochlorophyll-a was studied in homogeneous solution and in vitro in the presence of a macrophage cell line (P388-D1-ATCC). Photodynamic therapy (PDT) activity after different laser doses also was investigated. Scanning electron microscopy analysis of cell phagocyte nanoparticles showed that after 30 min of incubation most of the nanoparticles are in a clear adhesion process to the cell surface. The majority of nanoparticles became phagocytic after 2 hr of incubation time. After laser irradiation of the dye-containing system a total photodamage by nanoparticle phagocyte cells was observed and the cell survival was quantified by the 3-(4,5-dimethylthiazol-2-yl)-2,5-diphenyl tetrazolium bromide test. Our results indicate that polymeric nanoparticles work as an efficient drug delivery system for PDT drugs. This approach can be widely used for many other hydrophobic photosensitizers with higher aggregation tendency in neoplastic cell treatment.
During the past three decades photodynamic therapy (PDT) has become an established cancer treatment with the combination of two therapeutic agents. A photosensitizing drug and a visible light that are relatively harmless by themselves but when combined (in the presence of oxygen) lead to tumor destruction (Ochsner Citation1997; Van Tenten et al. Citation2000; Demoiseau et al. Citation2001). This is due to a sequence of biological events, including direct cytotoxicity, vascular damage, and immune modulation response (Grahn et al. Citation1997; Chen and Lu Citation1999).
Bacteriochlorophyll-a (BChl-a) is the main pigment in the light harvesting antenna process and the reaction center of photosynthetic purple bacteria (Peter Martinsson 2000). It consists of a tetrapyrrole ring with two of its pyrrole groups saturated. Among the various photosensitizers that have been under consideration, BChl-a was found to be highly promising for PDT. The absorption spectrum of monomeric BChl-a in a dilute organic medium (toluene) showed two absorption bands in the visible region at 560 and 770 nm (Q bands) and an intense band in the near-ultraviolet region at 360 nm (B or Soret band). Indeed, particular attention has been devoted to this sensitizer presenting a tumor cell preferential retention and absorbing above 700 nm, with a high molar extinction coefficient of about 90,000 M-1cm-1 at 780 nm.
These properties make it an alternative option to other molecules routinely used in PDT (e.g., porphyrin and phthalocyanine), already in clinical trial since the past decade, due to BChl-a absorption properties located in the middle of the “photodynamic window” (Borland, McGarvey, and Truscott Citation1987; Henderson et al. Citation1991). The “photodynamic window” is defined by the absorption region situated between 600 nm and 800 nm (Moser Citation1998). In this spectral region, endogenous tissular components are transparent to the incident radiation, minimizing the risk of photodamage at the level of cells or tissues not containing the photosensitizers. Moreover, under visible light irradiation, these dyes must produce oxygen reactive species able to react with the surrounding biological components inducing lethal damage by a complex biological pathway (Maryse Hoebeke 1999).
One of the main problems with BChl-a in PDT arises from its high tendency toward aggregation in physiological medium, once organic medium was not allowed to be used in biological system. This intermolecular association process promotes the nonradiative internal conversion, leading to a decrease of triplet excited state lifetime and reducing the overall photosensitizing efficiency (Fu et al. Citation2002). The strategy to reduce BChl-a aggregation in aqueous media involves the use of a delivery system, that can target malignant tissues, keeping the dye in a hydrophobic environment until it reaches as the intracellular medium.
Poly(DL-lactide-co-glycolide) (PLGA) nanoparticles are able to deliver many different compounds to the intracellular medium at a slow rate, which results in a sustained therapeutic effect of a variety of agents. These include macromolecules such as DNA, protein, drugs, vaccines, hormones, growth factors, and cytokines to treat various diseases (Crotts and Park Citation1998; Jiao et al. Citation2002; Cui and Mumper Citation2003). PLGA has shown a number of advantages over other biodegradable polymers used routinely for drug delivery. Special attention has to be paid to its biodegradability and biocompatibility approval for more than two decades for human use by the U.S. Food and Drug Administration (Panyam et al. Citation2002). According to Panyam et al. (Citation2002), the mechanism of action of nanoparticles is an important advantage in the use of PLGA nanoparticles as a cytoplasmatic delivery vehicle due to its characteristics.
Unlike other drug delivery systems, as cationic lipids or polymers structures, these nanoparticles of PLGA are cationic only in the endosomal compartment and do not destabilize the lysosomes by itself. This behavior reduces the chances of toxicity commonly associated with cationic lipids and cationic polymers allowing their efficient intracellular delivery (Panyam et al. Citation2002). At the same time macrophages and leukocytes are two of the most representative phagocyte cells acting in a biological system as a first defensive barrier to protect their host from invaders, by engulfing and digesting them very fast and efficiently (Okawa et al. Citation1989; Ashsan et al. Citation2002).
In this work, polymeric nanoparticles were formulated from biodegradable polymer PLGA that were evaluated as a potential controlled-release drug (BChl-a) delivery system to P388-D1 macrophage cells. The P388-D1 cells were selected for this study because they have been used as an in vitro model for studying the antiproliferative efficacy of different therapeutic agents (Moghimi, Hunter, and Murray Citation2001; Ashsan et al. Citation2002; Panyam et al. Citation2002). We demonstrated through scanning electron microscopy that particles loaded with BChl-a were absorbed efficiently by macrophages. We also observed that the laser light activated the photosensitizer resulting in the photodynamic damage of the cell.
MATERIAL AND METHODS
BChl-a was obtained from Porphyrin Products (Logan, UT, USA), PLGA (50:50, Mw 17 kDa) and 3-(4,5-dimethylthiazol-2-yl)-2,5-diphenyl tetrazolium bromide (MTT) were obtained from Sigma Chemical (St. Louis, MO, USA); poly(vinyl alcohol) (PVA) (13–23 kDa, 87–89% hydrolyzed) was supplied by Aldrich (Milwaukee, WI, USA). Dichloromethane and dimethylsulfoxide (DMSO) of analytical grade were supplied by Merck (Darmstadt, Germany). All other chemicals were of analytical grade and used without further purification.
BChl-a Solution and Nanoparticle Preparation
Fresh BChl-a solution was prepared by dissolving the dye in DMSO. The final concentration was calculated from the absorption measured at 780 nm, using a U-3000 spectrophotometer Hitachi (ε780 = 90,000 M-1 cm-1). Nanoparticles were produced by the solvent evaporation procedure described by Garcia et al. (Citation1999). Typically, the organic phase consisted of 0.1 g of PLGA 50:50 polymer and 50 μ M BChl-a dissolved in 10 mL of CH2Cl2. The dispersed phase was dropped into the aqueous homogeneous phase (100 mL of an aqueous phase containing 3% [w/v] of 88% hydrolyzed PVA as dispersing agent), under ice cooling, with continuous stirring at 13,500 rpm for 3 min using IKA Ultraturrax T25 equipped with S25N dispersing tool. Afterward, solvent evaporation was carried out by gentle magnetic stirring, at room temperature, usually occurring in 3–5 h. Nanoparticles were recovered by centrifugation for 5 min at 10,000 rpm and 4°C, washed (three times) with distilled water (10°C) and lyophilized. The final concentration of BChl-a loaded nanoparticle was analyzed using the molar extinction coefficient. Nanoparticles without dye were prepared by the same procedure to evaluate damage promoted by the nanoparticle itself.
Particle Size Analysis and Cell Culture
The particle mean size and the polydispersity index were assessed by Horiba CAPA-700 particle sizing system (Marven Instruments, Worcesterhire, UK). The index is an indication of the size distribution with values ranging from 0 to 1.0. Size measurements were carried out at different steps of the nanoparticle production. Each nanoparticle preparation was analyzed twice with 30 readings per sample suspended in distilled water. Mean particle size and polydispersivity index were calculated for each sample.
P388-D1 cells, a mouse macrophage monocyte line that grows in semisuspension, was obtained from the American Type Culture Collection (ATCC N° CCL46). Cells were grown in 75 cm2 flasks and maintained in RPMI-1640 medium, complemented with 10% fetal bovine serum, 1% of L-glutamine, and 1% of penicillin-streptomycin (Gibco BRL, Grand Island, USA). The cells were used in the logarithmic phase of growth and cultured in a humidified incubator at 37°C with 5% CO2. The day before the experiment, cells were seeded at 0.5× 106 cell/mL.
Scanning Electron Microscopy
For scanning electron microscopy (SEM) assays, macrophages were grown on glass pieces (20 × 20 mm) and placed in Petri dishes. Cells were exposed to dye-loaded nanoparticles (0 and 50 μ M) for 30 min and 2 hr. Preparation for scanning electron microscopy was carried out as follows: cells were fixed in 3% glutaraldehyde for 1 hr at room temperature and washed with 0.1 M phosphate buffer (pH 7.4). After washing, cells were postfixed in 0.1% of OsO4 for 30 min and washed with 0.1 M phosphate buffer (pH 7.4) at 40°C. Dehydration was carried out in ethanol 70% to 100% and acetone P.A. for 15 min each. A Blazers SCD-050 Coater sputter was used to coat the specimens with 5 nm of gold. Cells were examined and photographed by a Leica Stereoscan 440 scanning microscopy and Electronscan (Philips ESEM 2020). The microscope was operated at 5 kV. Similar procedure was used with cell-nanoparticles after laser irradiation. Unstimulated macrophages were used as controls.
MTT, Dark Toxicity, and Phototoxicity Assays
The photodestruction of cells was measured using a spectrophotometric 3-(4,5-dimethylthiazol-2-yl)-2,5-diphenyl tetrazolium bromide (MTT) assay that has been well characterized for use as classical procedure of cell viability evaluation after PDT effect (Grahn et al. Citation1997). MTT solution (150 μ L per well) containing 4 mg per mL of MTT-reagent was added to the cells in the 24-well plates followed by an incubation for 4 hr at 37°C. The samples were shaken for 2 hr in the dark. The absorbance at 570 nm was measured with U-3000 Hitachi spectrophotometer. The percentage of cell vitality was calculated with respect to control cells that were incubated without photosensitizer and nanoparticle.
The P388-D1 cells used were seeded at a concentration of 0.5 × 106 cell/mL in 2 mL per well of 24-well plates. They were incubated in the dark with a photosensitizer solution and dye-loaded nanoparticle at (0, 5, 10, 15, 25, and 50 μ M) in 37°C for 30 min and 2 hr with Hank's buffer. Following incubation, cells were washed to remove excess dye and unbound nanoparticle. Trypan blue exclusion method also was used to evaluate membrane integrity. Samples of these cell suspensions were taken in triplicate.
The P388-D1 cells were incubated with BChl-a and nanoparticle in RPMI media for 30 min and 2 hr. Immediately before irradiation, the culture medium was removed, and the cells were washed with Hank's buffer and placed within a 3-mL cuvette in a laminar flow cabinet thermostatically controlled at 37°C. Irradiations were performed using Nd-YAG laser system (355 nm). Irradiances of typically 30 to 35 mW per pulse were used to deliver total fluences of 30 J/cm2. The temperature during the experiment was maintained at 37°C using a thermostatically control chamber. Immediately after light exposure, cells were incubated in RPMI 1640 in the absence of phenol red medium for 24 hr at 37°C. At 24 hr after light treatment, the cells were incubated with 150 μ L MTT for 4 hr to quantify reduction by mitochondrial dehydrogenases. The reaction product, formazan, was quantified photometrically at 570 nm, and the data were normalized to a control set of cells where no light or photosensitizer was applied. Samples of these cell suspensions were taken in triplicate. A control with cell and light (30 mW) was performed to confirm that the light by itself is not toxic to this cell line.
Data Report
Each set of experiments described was repeated at least five times. Results were reported as mean ± standard deviation (SD) in text, and statistical analysis was performed using a two-tailed Student's t-test. A p value less than.05 was considered indicative of a significant difference between data.
RESULTS AND DISCUSSION
BChl-a was found to be a highly promising dye for the use in PDT due to its spectroscopic characteristics. It has an absorption band located in the middle of the “photodynamic window,” among others (Borland et al. Citation1987; Henderson et al. 1997) and a fluorescence emission at 790 nm that makes it useful as a tool for tumor diagnosis in early stages as shown in .
Figure 1 Absorption spectra of BChl-a (2 μ M) in toluene. Insert: fluorescence emission spectra excitated at 710 nm.
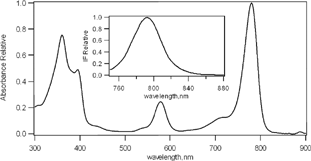
The tendency of aggregation of BChl-a in solution is one of the main problems of use of this drug in PDT in physiological medium, because this intermolecular association process promotes the nonradiative internal conversion, causing a decrease of excited triplet lifetime and reducing the overall photosensitizing efficiency (Fu et al. Citation2002). The strategy used in this work to reduce BChl-a aggregation in aqueous media involves a drug delivery system, that can target malignant tissues. In particular, nanoparticles could offer a number of advantages with respect to other drug delivery systems (liposomes, dendrimers, and erythrocytes): they maintain their physical-chemistry characteristics unaltered for long periods allowing long-term storage; depending on their composition they can be administered through different ways (oral, intramuscular, or subcutaneous); and they are suitable for scaling up and industrial production [E. Esposito 1999].
Nanoparticles Analyses
The choice of the encapsulation method was determined by the solubility characteristics of both BChl-a and the polymers. BChl-a was used as a hydrophobic photosensitizer. Therefore, we decided to use the classical solvent evaporation method to produce stable and sized control nanoparticles. For all nanoparticles formulations obtained, the polydispersivity index was lower than 0.3, which indicates a narrow particle size distribution, as expected for a drug and a delivery system to be used in systemic administration. This method led to the smallest nanoparticles with a mean diameter of 670 ± 100 nm as shown in . Its profile also was detected by ultrastructural analysis.
Ultrastructural Analysis
Phagocytosis of nanoparticles loaded with BChl-a used in our work in P388-D1 macrophage cell is a process controlled by the physical conditions of nanoparticles including the size, surface chemistry, and morphology. The above results support that PLGA nanoparticles are efficient delivery vehicles to stabilize and deliver drugs such as BChl-a in the treatment of cancer by PDT.
In our study the incubation process (30 min and 2 hr) of nanoparticles by P388-D1 cells was evaluated. The efficiency of phagocytosis were determined (adhesion or phagocytosis process) of nanoparticle in P388-D1 cells with the surface morphology of the cells analyzed by SEM presents a micrograph of P388-D1 cells incubated with nanoparticle for 30 min showing that after this incubation time, more that 70% of nanoparticles already were adhered to the cell surface.
B shows that P388-D1 cells incubated with nanoparticle for 2 hr presented more than 90% of nanoparticles completely phagocyted by the cell, appearing as an extension of the cell surface. This result from the incubation process provided the minimum time needed to reach the great majority of these nanoparticles phagocyted by cell. Simillar conclusions were observed by Konan et al. (Citation2003) with sub-130 nm nanoparticles of PLGA loaded with Meso-tetra(4-hydroxyphenyl)porphyrin (p-THPP) with incubating time of 15 or 30 min on EMT-6 mammary tumor cells evaluated subcellular localization and efflux of EMT-6 tumor mammary cells in comparison with the free dye. These results suggest that for each cell line the incubation time is an important parameter to be investigated to obtain the best results in PDT.
Cellular Photoinactivation on Phagocytic Cells
PDT involves administration of a tumor-localizing photosensitizing agent, followed by activation of the agent by a light wavelength matching the dye absorption spectrum. This triggers a sequence of photochemical and photobiological processes that cause irreversible photodamage to the tumor cell.
The PDT activity of BChl-a (50 μ M) solution and nanoparticle system after 2 hr of incubation was confirmed using the MTT test, following different light dose (0–30 Jcm-1) (). No toxicity was observed for the concentration of the dye studied (50 μ M) and for the light dose alone. The survival experiments showed that both BChl-a dye solution and loaded nanoparticle are able to mediate photodynamic killing of P388-D1 cells.
Figure 4 Effect of light dose on cell survival of P388-D1. □-Incubation with 50 μ M of BChl-a and ▪-BChl-a encapsulated in nanoparticles (MTT test). Each set of experiments described so far was repeated at least three times. Results were reported as mean ± standard deviation in text, and statistical analysis was performed using a two-tailed Student's t-test.
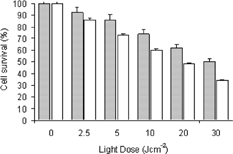
Our results showed that for P388-D1 cells the LD50 (light dose required of 50% cell inactivation) for BChl-a is 20 Jcm-2. When the photosensitizer is encapsulated in the nanoparticles, at the same laser dose, a decrease of cell death is observed. PLGA nanoparticles takes weeks to be completely biodegradable releasing the drug in the medium (Panyam et al. Citation2002). Our results also showed that this delivery system even in a short period of time (2 hr) in contact with the biological medium was an efficient drug delivery with cell death after light action. These results suggest that in a longer period of time the percentage of cell survival could diminished after PDT treatment. We also evaluated the ultrastructural surface morphology of macrophages, incubated with BChl-a after photodynamic action, showing considerable damage by rupture of the cell membrane with loss of cytoplasmic material.
The ultrastructural macrophages morphology when incubated with BChl-a solution is shown in A. A presents the P388-D1 cell without irradiation, whereas B shows P388-D1 cells irradiated with a fluency of 30 J/cm-2 (λ =355 nm). The cell membrane initial rupture due to lightphotosensitizer effect is easily observed after cell irradiation.
Figure 5 Scanning electron micrograph of macrophage (P388-D1 cells) exposed to BChl-a solution. (A) control (without irradiation) shows intact cell; (B) BChl-a (50 μM) after a 30 J/cm2 irradiation dose shows photodamage with cell morphology alteration. Scale barr 1 μ m.
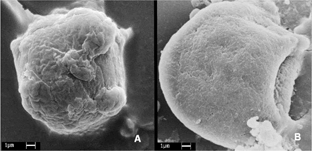
Cell uptake of nanoparticle (without irradiation) indicating no cellular damage was shown in A. On the contrary, B shows a micrograph presenting photodamage of P388 cell with nanoparticle after irradiation with a fluency of 30 J/cm-2 (355 nm), showing a burst of nanoparticle inside the cell with release of photosensitizer. Our cellular ultrastructural analysis of cell capture of nanoparticle indicates that this process might take place in two steps: (1) nanoparticle adhered to cell surface and (2) a burst of phagocyte nanoparticle with drug release followed by irradiation (Dune, Corrigan, and Ramtoola Citation2000) with a final effect of cell death.
CONCLUSION
BChl-a, a promising drug for PDT, showed that its photophysical characteristics match almost all requirements for a good candidate for clinic trials with absorption of the optical therapeutical window in solution or when associated with PLGA nanoparticle. In our study we demonstrated that nanoparticle of PLGA loaded with BChl-a is readily internalized by macrophages after 2 hr of incubation. Our results also show that phagocyte cells when incubated with nanoparticles loaded with BChl-a are susceptible to fast photodamage, after laser irradiation evaluated by MTT assay and SEM. These results reinforce the idea of using PLGA nanoparticles as a drug delivery systems for hydrophobic photosensitizers useful in PDT for neoplastic tissue.
The authors gratefully acknowledge the Säo Paulo State Foundation for Research Support (FAPESP) for financial support to A. J.Gomes grant No. 98/11712-0 and to C. N. Lunardi grant No. 00/00431-1. The authors thank Dr. Flávio Henrique Caetano from the Electron Microscopy Center of the Instituto de Biociência da UNESP-Rio Claro, and Dr. Julien F.C.Boodts from the Institute of Chemistry of UFU.
REFERENCES
- Ashsan F., Rivas I. P., Khan M. A., Suárez A. I. T. Targeting to macrophage: role of physicochemical properties of particulate carriers—liposomes and microsphere—on the phagocytosis by macrophage. J. Control. Rel. 2002; 79: 29–40
- Borland C. F., McGarvey D. J., Truscott T. G. Photophysical studies of bacteriochlorophyll-a and bacteriopheophytin-a—singlet oxygen generation. J. Photochem. Photobiol. B: Biol. 1987; 1: 93–101, [CROSSREF]
- Chen W., Lu D. R. Carboplatin-loaded PLGA microspheres for intracerebral injection: formulation and characterization. J. Microencapsul. 1999; 16: 551–563, [PUBMED], [INFOTRIEVE], [CROSSREF]
- Crotts G., Park T. G. Protein delivery from poly(lactic-co-glycolic acid) biodegradable microsphere: release kinetics and stability issues. J. Microencapsul. 1998; 15: 699–713, [PUBMED], [INFOTRIEVE]
- Cui Z., Mumper R. J. The effect of co-administration of an adjuvant with nanoparticle-based genetic vaccine delivery system on the resulting immune response. Eur. J. Pharm. Biopharm 2003; 55: 11–18, [PUBMED], [INFOTRIEVE], [CROSSREF]
- Demoiseau X., Schuitmaker H. J., Lagerberg J. W. M., Hoebeke M. Increase of the photosensitizing efficiency of the bacteriochlorin-a by liposome-incorporation. J. Photochem. Photobiol. B: Biol. 2001; 60: 50–60, [CROSSREF]
- Dune M., Corrigan O. I., Ramtoola Z. Influence of particle size and dissolution conditions on the degradation properties of polylactice-Co-glycolide particles. Biomaterials 2000; 21: 1659–1668, [CROSSREF]
- Esposito E., Sebben S., Cortesi R., Menegatti E., Nastruzzi C. Preparation and characterization of cationic microspheres for gene delivery. International Journal of Pharmaceutics 1999; 189: 29–41, [PUBMED], [INFOTRIEVE], [CROSSREF]
- Fu F., Li X., Ng D. K. P., Wu C. Encapsulation of phthalocyanines in biodegradable poly(sebacic anhydride) nanoparticles. Langmuir 2002; 18: 3843–3847, [CROSSREF]
- Garcia J. T., Farina J. B., Munguia O., LLabrés M. Comparative degradation study of biodegradable microspheres of poly(dl-lactide-co-glycolide) with poly(ethyleneglycol) derivates. J. Microencapsul. 1999; 16: 83–94, [PUBMED], [INFOTRIEVE], [CROSSREF]
- Grahn M. F., McGuinness A., Benzie R., Boyle R., Jode M. L., Dilkes M. G., Abbas B., Willians N. S. Intracellular uptake, absorption spectrum and stability of the bacteriochlorin photosensitizer 5,10,15,20-tetrakis(m-hydroxyphenyl)bacteriochlorin (mTHPBC). Comparison with 5,10,15,20-tetrakis(m-hydroxyfenyl)chlorine (mTHPC). J. Photochem. Photobiol. B: Biol. 1997; 37: 261–266, [CROSSREF]
- Henderson B. W., Sumlin A. B., Owczarczak B. L., Dougherty T. J. Bacteriochlorophyll-a as photosensitizer for photodynamic treatment of transplantable murine tumors. J. Photochem. Photobiol. B: Biol. 1991; 10: 303–313, [CROSSREF]
- Jiao Y., Ubrich N., Marchand-Arvier M., Vigneron C., Hoffman M., Lecompte T., Maincent P. In vitro and in vivo evaluation of oral heparin–loaded polymeric nanoparticles in rabbits. Circulation 2002; 105: 230–235, [PUBMED], [INFOTRIEVE], [CROSSREF]
- Konan Y. N., Chevallier J., Gurny R., Allémann E. Encapsulation of p-THPP into nanoparticles: cellular uptake, subcellular localization and effect of serum on the photodynamic activity. Photochem. Photobiol. 2003; 77: 638–644, [PUBMED], [INFOTRIEVE], [CROSSREF]
- Moghimi S. M., Hunter A. C., Murray J. C. Long-circulation and target-specific nanoparticles: theory to pratice. Pharmacol. Rev. 2001; 53: 283–318, [PUBMED], [INFOTRIEVE]
- Moser J. G. Photodynamic tumor therapy: 2nd and 3rd generation photosensitizer. Harwood Academic Publishers, New DelhiIndia 1998; 3–6, 43-49
- Ochsner M. Photophysical and photobiological processes in the photodynamic therapy of tumors. J. Photochem. Photobiol. B: Biol. 1997; 39: 1–18, [CROSSREF]
- Okawa T., Ichimal H., Ishida T., Kawata M., Kaguba S., Mamba K., Makita T. Implication of activation of intraperitoneal macrophages with biodegradable microsphere. Cell Biol. Int. Rep. 1989; 13: 547–553, [PUBMED], [INFOTRIEVE], [CROSSREF]
- Panyam J., Zhou W. Z., Prabha S., Sahoo S. K., Labhasetwar V. Rapid endo-lysosomal escape of poly(dl-lactide-co-glycolide) nanoparticle: implications for drug and gene delivery. FASEB J. 2002; 16: 1217–1226, [PUBMED], [INFOTRIEVE], [CROSSREF]
- Van Tenten Y., Schuitmaker J. J., De Groot V., Willekens B., Vrensen G. F. J. M., Tassignón M. J. Cell biological mechanisms underlying posterior capsule opacification: search for a therapy. Bull. Soc. Belge Ophthalmol 2000; 278: 61–66