Abstract
A polysaccharide hydrogel was isolated from the seeds of Tamarindus indica (tamarind) and was used as release modifier for the preparation of diclofenac sodium spheroids, using extrusion-spheronization technique. The process was studied for the effect of variables to arrive at spheroids with satisfactory particle shape, size and size-distribution. The prepared spheroids were characterized for surface morphology, qualitative surface porosity, friability, bulk density, and flow properties. The in vitro release studies exhibited a zero-order release kinetics that was confirmed by Higuchi's and Peppas' models. A credible correlation was obtained among swelling index, viscosity, surface roughness of the polysaccharide, and in vitro dissolution profile of the spheroids. In the comparative bioavailability study, we found that the developed spheroids were able to sustain the drug release over 8 hr and could improve the extent of absorption and bioavailability of the drug.
Spheroids are agglomerates of fine powders or granules of bulk drugs and excipients. They consist of small, free-flowing, spherical or semispherical solid units, typically from ∼0.5 to 1.5 mm and are intended usually for oral administration (Gajdos Citation1984; Kristensen and Schaefer Citation1987; Ghebre-sellassie Citation1989). The spheroids offer certain specific advantages over conventional solid forms, which include free flow and ease of packing, which resulting in reproducible and uniform fill weight of capsules (Vuppala, Parikh, and Bhagat Citation1997; Conine and Hadley Citation1970). Film coating can be applied on spheroids easily due to their ideal spherical shape. Spheroids of different drugs can be blended and formulated in a single dosage form. Even spheroids of different release rates of the same drug can be supplied in a single unit dosage form. The spheroids can freely disperse in the gastrointestinal tract as subunit, which maximizes drug absorption and minimizes peak plasma fluctuations. Local irritation derived from high local concentration of drug from a single unit dosage form can be avoided (Ghebre-sellassie Citation1989). It has been reported that spheroids smaller than 2.4 mm diameter are free from digestive function of the stomach and the closing system of pyloric sphincter to be emptied from the stomach (Davies, Wilson, and Washington Citation1987; Feely, Khosla, and Davis Citation1987).
Many methods have been reported for the preparation of spheroids: compaction, drug layering, melt spheronization, globulation, balling, compression, and extrusion-spheronization. Among these, extrusion-spheronization is the most popular method (CitationGhali et al. 1990; Reynolds Citation1970). Although the main thirst of spheronization processes is to manufacture spherical drug cores that will be subsequently coated to modify the drug release, it also is possible to prepare spheroid cores that inherently possess specific release profiles. This is achieved by the incorporation of substances that modify the drug release. These substances are called release modifiers. To retard the drug release, polymers such as shellac and waxes are used (Harns and Ghebre-sellassie Citation1989).
Gums and mucilages are polysaccharide complexes formed from sugar and uronic acid units. They can absorb large quantity of water and swell. They find a wide range of pharmaceutical applications that include their use as binders and disintegrants in tablets, emusifiers, suspending agents, and gelling agents. They also are used as sustaining agents in tablets. Many gums and mucilages have been reported to sustain the drug release from matrix tablets (Kulkarni et al. Citation2002; Baveja, Rangarao, and Arora Citation1988). Thus, in the present study, polysaccharide isolated from the seeds of Tamarindus indica (tamarind) has been used as a release modifier to formulate controlled release spheroids using diclofenac sodium as model drug.
MATERIALS AND METHODS
The seeds of Tamarindus indica were purchased from local market. Acetone (AR grade) was obtained from Ranbaxy Fine Chemicals, (Mumbai, India). Microcrystalline cellulose (MCC) was obtained from Loba Chemie (Mumbai, India). Acetonitrile and triethylamine (HPLC grade) were obtained from Qualigens (Mumbai, India). Diclofenac sodium was gift sample from Cipla Ltd. (Pune, India). All other chemicals and reagents used in the present study were of AR grade.
Methods
Isolation of Polysaccharide
The isolation of polysaccharide was done by following a reported method with modification (Baveja et al. Citation1988; Kulkarni et al. Citation2002). Seeds of Tamarindus indica were crushed and the outer brown seed coat was removed. Then, 250 g of seeds were soaked in water (7.5 L) for 24 hr, heated at 80°C for 2 hr, and kept aside for 2 hr, for the release of polysaccharide into water. The soaked seeds were taken and squeezed in a muslin bag to separate marc from the filtrate. The filtrate was concentrated to half of its original volume under vacuum at 40°C, and an equal quantity of acetone was added to the filtrate to precipitate the polysaccharide. The precipitated polysaccharide was separated by filtration, dried at 40°C under vacuum, powdered, and stored in airtight container at room temperature.
Swelling Index, Viscosity, and Surface Characteristics of Polysaccharide
Swelling index of polysaccharide was determined by a reported procedure (Anonymous Citation1998). The viscosity of 1% solution of the polysaccharide was determined using a Brookfield RVDV II+ viscometer (Brookfield Engineering, USA) with a small sample adapter (spindle # S28, at 50 rpm). To study the surface characteristics of the polysaccharide, scanning electron micrograph (SEM) of the powder which was (previously passed through British Standard Sieve 80) was used. The powder was evaporated with carbon and then sputtered with gold to make the samples electrically connected. Carbon was layered to a thickness of ∼10 nm and gold was layered to ∼25 nm. The SEM was taken in S-2400 equipment (Hitachi, Tokyo, Japan).
Extrusion-Spheronization: Process Optimization
For the preparation of spheroids, Extruder (EXT-65/037, R.R. Enterprises, Thane, India) and Spheronizer (SPH-150/010, R.R. Enterprises) were used. In the formula of spheroids, MCC was used as spheronization enhancer. For the optimization of formula for spheroids, different ratios of MCC-drug-polysaccharide, such as 65:30:5, 60:30:10, 55:30:15, 50:30:20, and 45:30:25, were selected and subjected to extrusion-spheronization. For the optimization of spheronization speed, extrudes from all the selected ratios of MCC-drug-polysaccharide were subjected to spheronization at different speeds such as 300, 400, 500, 1000, and 2000 rpm. For optimization of spheronization time, the ideal batch of spheroids was subjected to spheronization at 400 rpm for different duration of time such as 10, 15, 20, 25, and 30 min.
Physical Characterization of Spheroids
The prepared spheroids were characterized for different physical properties. The particle size and size distribution were determined by sieve analysis (Martin, Bustamante, and Chun Citation1994). The surface morphology and qualitative surface porosity of the spheroids were analyzed using SEM (Bajaj and Savarkar Citation1999; Mehta Citation1989). The friability, bulk density, and flow properties of the spheroids were determined using standard methods (Martin et al. Citation1994; Banker and Neil Citation1987).
In Vitro Dissolution
To study the in vitro dissolution profile, spheroids equivalent to 100 mg of diclofenac sodium were filled in hard gelatin capsules manually. Dissolution studies were carried out using USP-XXIII dissolution apparatus (Electrolab, Mumbai, India) attached with paddle. Freshly prepared buffer of pH 7.2 (900 ml) was placed in the dissolution flask and allowed to attain a temperature of 37 ± 10°C. The capsules were placed at the bottom of the dissolution flask. The paddle was rotated at 50 rpm. The 5 ml of samples were withdrawn on different time intervals and after each withdrawal, an equal quantity of fresh buffer of pH 7.2 was added to the dissolution medium. The samples were diluted suitably with same buffer and analyzed spectrophotometrically at 276 nm using an ultraviolet spectrophotometer (Shimadzu, 160A, Japan) (United States Pharmacopoeia Citation2000).
Bioavailability Studies
Bioavailability studies were carried out using 12 healthy human volunteers of 22–25 years age, weighing between 50–70 kg. Their liver and kidney functions in them were assessed by clinical and biochemical tests and were found to be normal. The subjects selected did not use alcohol or tobacco and had not taken any medication for 1 week prior to the study. All the volunteers gave written consent and the study protocol was approved by the ethical committee of the institution.
The bioavailability study was carried out in two phases. The volunteers were divided into two groups of 6 members each. In the first phase of the study, the first group received the capsules containing ideal batch of spheroids equivalent to 100 mg of diclofenac sodium, orally, after a overnight fasting. The second group received marketed sustained release tablets containing 100 mg of diclofenac sodium. In the second phase, the first group received marketed formulation and the second group received capsules containing spheroids. Blood samples (4 ml) were collected in heparinized centrifuge tubes at 0, 0.5, 1, 2, 4, 6, and 8 hr after dosing. The collected samples were centrifuged at 3000 rpm to separate plasma. Reverse phase HPLC method was used for the estimation of diclofenac sodium in plasma (Shoog Citation1996).
Chromatographic Conditions
A Waters® Breeze HPLC system was used for the analysis. The column used was Phenomenex Luna® C18 (5 μ m, 25 cm * 4.6 mm id). A mixture of acetonitrile-triethylamine (60:40 v/v, adjusted to pH 3.5 using orthophosphoric acid) was used as mobile phase at a flow rate of 1.0 ml/min with an operating pressure of 3000 psi. A Rheodyne-7125 injector with a 20-μ l loop was used for injection of samples. Detection was done at 276 nm with a sensitivity of 2.0000 AUFS. The mobile phase was filtered through 0.22 μ m membrane filter and degassed. The separation was carried out at temperature 20 ± 1°C (Anonymous Citation1996; Shoog Citation1996).
RESULTS AND DISCUSSION
After aqueous extraction and acetone treatment, the yield of the polysaccharide was found to be 78.07 ± 0.009% w/w. The swelling index of the polysaccharide was found to be 18.7 ± 1.021 ml, and the viscosity of 1% solution of the polysaccharide was found to be 74.69 ± 0.308 cP. depicts a SEM of the isolated polysaccharide. The particles exhibited rough surface with pores and crevices. From the SEM, it also is evident that the particle size distribution was not in a narrow range. A major portion of the powder consisted of fine particles, which might fill the voids between the larger ones and lead to compact000 packing.
TABLE 1 Physical characteristics of tamarind seed polysaccharide
Tables and show the results of optimization of extrusion-spheronization process. In the formula optimization study, we found that as the concentration of polysaccharide was increased, the granulating mass became elastic and extrudes were not formed. At very low concentrations of polysaccharide, spheroids were formed but a major fraction of the particles was irregular in shape. Also, the spheronized mass had a wide range of size distribution that was not ideal. Hence, MCC-drug-polysaccharide ratio of 60:30:10 was selected. In the optimization of spheronization speed, we found that at lower speeds, more numbers of rod- and dumbbell-shaped particles were obtained. At higher speeds, due to centrifugation, spheronization did not occur properly, again leading to the formation of rod- and dumbbell-shaped particles.
TABLE 2 Results of optimization of spheronization process
In the optimization of spheronization time, we found that at lower spheronization times (i.e., less than 15 min), a major portion of the granulating mass was not converted into spheroids and at longer residence times, due to evaporation of water, the particles were dried and thus, size reduction was observed. Under the ideal conditions, viz., MCC-drug-polysaccharide ratio of 60:30:10, spheronization time of 20 min at 400 rpm speed, maximum yield of spherical particles was obtained ().
TABLE 4 Characteristics of spheroids
For the characterization of spheroids, different physical properties were determined. In the size distribution analysis, we found that the spheroids had a narrow range of size distribution and 72.46% spheroids were within a size range of 0.655–0.855 mm and the size distribution was found to be normal. The average particle size of the spheroids was 0.699 mm. The SEM of spheroids () clearly exhibited spherical shape of the spheroids with smooth and non-porous surface.
TABLE 3 Results of optimization of spheronization time
The bulk density of the spheroids was found to be 0.88 ± 0.003 g/cc, which indicated that the spheroids were “heavy”0 in nature, which may be because of closet packing arrangement. The friability value for an ideal batch of spheroids was found to be 0.77 ± 0.003%. This shows that the spheroids were less friable, which might be due to the spherical shape and smooth surface of the spheroids, as indicated by SEM. This also can be correlated to the high viscosity of the polysaccharide, which imparts better cohesion in the powder mass, and results in less friable spheroids. The angle of repose value for the ideal batch of spheroids was found to be 23.76 ± 0.032, which is an indication of good flow. This also can be attributed to the spherical shape and smooth surface of the spheroids.
In the in vitro release study, the spheroids exhibited a linear release pattern, when the dissolution data were plotted as cumulative percentage of drug released against time (). The release profile indicated that the drug release from these spheroids followed zero-order and was diffusion controlled. For the confirmation of linearity, correlation coefficient (r) was calculated and it was found to be 0.978 indicating a perfect linearity.
FIG. 3 In vitro release profile of diclofenac sodium from spheroids of tamarind seed polysaccharide.
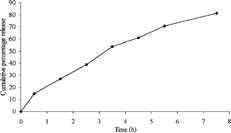
For the confirmation of order of release, the dissolution data were plotted as cumulative percentage of drug released against square root of time, according to Higuchi's classic equation (Higuchi Citation1963), which gives study state drug release. This plot also was a straight line (r = 0.995, ), indicating a diffusion-controlled release of drug from the spheroids.
FIG. 4 Higuchi's plot for the release data of diclofenac sodium from spheroids of tamarind seed polysaccharide.
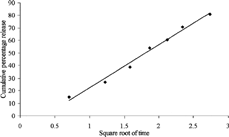
To confirm the mechanism of drug release and also to verify the fact that whether diffusion is Fickian or not, the dissolution data were also plotted according to Peppa's equation, in which log cumulative percentage of drug released is plotted against log time () (Peppas Citation1985). The log-log plot for tamarind spheroids also was a straight line (r = 0.997), with slope value of 1.238, indicating that the release of drug followed non-Fickian anomalous diffusion.
FIG. 5 Peppas' plot for the release data of diclofenac sodium from spheroids of tamarind seed polysaccharide.
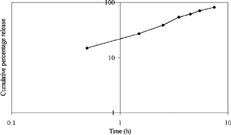
The zero-order kinetics of drug release from the spheroids could be attributed to the high viscosity and swelling index of the polysaccharide (Fell Citation1988; Rowe Citation1989). The high viscosity indicates the formation of a three-dimensional gel-like network that might retard the drug release. This could be supported by high swelling index of the polysaccharide, which is an indication of absorption of a large quantity of water and swelling of the polysaccharide. This can lead to the formation of three-dimensional network with aqueous channels in the matrix (Brunneton Citation1995). The drug diffusion might occur through these channels. The SEM of the polysaccharide powder exhibited a rough surface with pores and crevices. Earlier it had been reported that the excipients with rough surface can retard the drug release, due to entrapment of drug in the pores and crevices (Sallam et al. Citation1988).
In the bioavailability study, the data were plotted as plasma concentration of drug against time for ideal batch of spheroids as well as marketed sustained release tablets (). The plasma concentration of the drug was found to be higher with prepared spheroids than the marketed sustained release formulation. For further comparison, maximum plasma concentration (Cmax), time required for maximum plasma concentration (Tmax), and area under the plasma concentration time curve (AUC) were calculated ().
TABLE 5 Pharmacokinetic parameters for the spheroids and marketed formulation of diclofenac sodium
FIG. 6 Plasma concentration-time profile of diclofenac sodium from prepared spheroids and marketed sustained release tablet.
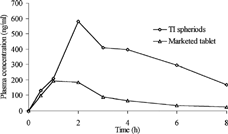
The Cmax for the prepared spheroids was found to be 580.90 ng/ml, that was attained in 2 hr (Tmax) post dosing. Following oral administration of a single dose of diclofenac sodium as sustained release tablet (marketed), the mean peak plasma concentration of 192.12 ng/ml was attained in 1 hr. Hence, we can state that the prepared spheroids were better than the marketed formulation in sustaining the drug release. Also, the extent of absorption of drug was found to be higher in the spheroids than the marketed formulation, which was confirmed by the AUC values. We found that spheroids exhibited higher AUC than the marketed formulation. The increase in AUC represents better absorption and bioavailability of drug from the formulated spheroids. These results may be because of higher surface area of the spheroids when compared with sustained release tablet, and also due to the spreading of spheroids in the gastrointestinal tract as separate units. The better absorption also can be attributed to the retarded release of drug form spheroids. During in vitro dissolution study, the spheroids were found to float on the surface of the medium. Such behaviour might increase the residence time and in turn bioavailability.
CONCLUSION
The isolated polysaccharide from the seeds of Tamarindus indica was found suitable to be used as a sustaining agent. The spheroids developed using this polysaccharide as release modifier, MCC as spheronization enhancer, and diclofenac sodium as model drug were found to sustain the drug release over a period of 7.5 hr. A credible correlation was obtained among swelling index, viscosity, surface roughness of the polysaccharide particles, and the in vitro dissolution profile of the spheroids. In the comparative bioavailability study, the developed spheroids were able to sustain the drug release and also were found to improve the extent of absorption and bioavailability of the drug.
REFERENCES
- Anonymous. Validation of Analytical Procedures: Methodology. ICH Harmonized Tripartite Guideline. 1996
- Anonymous. Quality Control Methods for Medicinal Plant Materials. World Health Organization, Geneva 1998
- Bajaj A. N., Savarkar S. P. Pelletization by extrusion-spheronization technique—process optimization. Ind. Drugs 1999; 36: 44–49
- Banker G. S., Neil R. A. The Theory and Practice of Industrial Pharmacy., L. Lachman, A. H. Liberman, J. L. Kanig. Varghese Publishers, Mumbai 1987; 297–321
- Baveja S. K., Rangarao K. V., Arora J. Examination of natural gums and mucilages as sustaining materials in tablet dosage forms. Ind. J. Pharm. Sci. 1988; 50: 89–92
- Brunneton J. Pharmacognosy, Phytochemistry Medicinal Plants. Lavoisier, New York 1995
- Conine J. W., Hadley H. R. Small solid pharmaceutical spheres. Drug Cosmet. Ind. 1970; 90: 38–41
- Davies S. S., Wilson C. G., Washington N. Gastrointestinal transit of a controlled-release pellet formulation of tiaprofenic acid and the effect of food. Int. J. Pharm. 1987; 35: 253–258, [CROSSREF]
- Feely L. C., Khosla R., Davis S. S. Investigating the gastric emptying of different sizes of non-disintegrating tablets in humans using gamma scintigraphy. J. Pharm. Pharmacol. 1987; 39: 31
- Fell J. T. Surface and interfacial phenomenon. Pharmaceutics—The Science of Dosage Form Design., M. E. Aulton. Churchill Livingstone, New York 1988; 50–61
- Gajdos B. Rotary granulators-evaluation of process technology for pellet production using factorial design. Drugs Made Ger. 1984; 27: 30–36
- Ghali E. S., Contractor A. M., Mankad A. D., O'Connor R. E., Schwartz. A high-speed mixer for continuous wet granulation. Pharm. Tech. April, 1990; 60–66
- Ghebre-sellassie I. Pharmaceutical Pelletization Technology. Marcel Dekker, New York 1989; pp. 1–13
- Harns M. R., Ghebre-sellassie I. Formulation variables. Pharmaceutical Pelletization Technology., I. Ghebre-sellassie. Marcel Dekker, New York 1989; 217–240
- Higuchi T. J. Pharm. Sci. 1963; 52: 1145–1149, [PUBMED], [INFOTRIEVE]
- Kristensen H. G., Schaefer T. Granulation. A review of pharmaceutical wet granulation. Drug Dev. Ind. Pharm. 1987; 13: 803–872
- Kulkarni G. T., Gowthamarajan K., Brahmajirao G., Suresh B. Evaluation of binding properties of selected natural mucilages. J. Sci. Ind. Res. 2002; 61: 529–532
- Martin A., Bustamante P., Chun A. H. C. Physical Pharmacy. B. I. Waverly, New Delhi 1994; pp. 426–446
- Mehta A. M. Evaluation and characterization of pellets. Pharmaceutical Pelletization Technology., I. Ghebre-Sellassie. Marcel Dekker, New York 1989; 241–265
- Peppas N. A. Analysis of Fickian and non-Fickian drug release from polymers. Pharm. Acta Helv. 1985; 60: 110–111, [PUBMED], [INFOTRIEVE]
- Reynolds A. D. A new technique for the production of spherical particles. Manuf. Chem. 1970; 41: 40–43
- Rowe R. C. Polar and non-polar interactions in the granulation of organic substances with polymer binding agents. Int. J. Pharm. 1989; 56: 117–124, [CROSSREF]
- Sallam E., Ibrahim M., Takieddin M., Shamat A., Baghal T. Drug Dev. Ind. Pharm. 1988; 14: 1277–1302
- Shoog D. A. Analytical Chemistry. W.B. Saunders, Philadelphia 1996
- United States Pharmacopoeia-24/National Formulary-19Asian edition. USP Conventional Inc., Rockville, MD 2000
- Vuppala M. K., Parikh D. M., Bhagat H. R. Application of powder-layering technology and film coating for manufacture of sustained-release pellets using a rotary fluid bed processor. Drug Dev. Ind. Pharm. 1997; 23: 687–694