Abstract
Hyaluronic acid (HA), is a polyanionic polysaccharide that consists of N-acetyl-D-glucosamine and β-glucoronic acid. It is most frequently referred to as hyaluronan because it exists in vivo as a polyanion and not in the protonated acid form. HA is distributed widely in vertebrates and presents as a component of the cell coat of many strains of bacteria. Initially the main functions of HA were believed to be mechanical as it has a protective, structure stabilizing and shock-absorbing role in the body. However, more recently the role of HA in the mediation of physiological functions via interaction with binding proteins and cell surface receptors including morphogenesis, regeneration, wound healing, and tumor invasion, as well as in the dynamic regulation of such interactions on cell signaling and behavior has been documented. The unique viscoelastic nature of hyaluronan along with its biocompatibility and nonimmunogenicity has led to its use in a number of cosmetic, medical, and pharmaceutical applications. More recently, HA has been investigated as a drug delivery agent for ophthalmic, nasal, pulmonary, parenteral, and dermal routes. The purpose of our review is to describe the physical, chemical, and biological properties of native HA together with how it can be produced and assayed along with a detailed analysis of its medical and pharmaceutical applications.
Hyaluronic acid (HA), discovered in bovine vitreous humor by Meyer and Palmer in Citation1934, is most frequently referred to as hyaluronan due to the fact that it exists in vivo as a polyanion and not in the protonated acid form. HA is ubiquitous in that it is distributed widely in vertebrates and present as component of the cell coat of many strains of bacteria (e.g., A. Streptococcus) (Laurent Citation1970). Commercially produced HA is isolated either from animal sources within the synovial fluid, umbilical cord, skin, and rooster comb, or from bacteria through fermentation or direct isolation. The molecular weight of HA is heavily dependent on its source; however, refinement of these isolation processes has resulted in the commercial availability of numerous molecular weight grades extending up to a maximum of 5,000,000 daltons (Milas et al. Citation2001). Extensive studies on the chemical and physicochemical properties of HA and its physiological role in humans, together with its versatile properties, such as its biocompatibility, nonimmunogenicity, biodegradability, and viscoelasticity, have proved that it is an ideal biomaterial for cosmetic medical and pharmaceutical applications.
Several of HA's important physicochemical properties include molecular weight dependence. Therefore discrete differences in function over the wide range of commercially available molecular weights enables HA to used in a diverse set of applications. In addition, chemical modification of hyaluronan can confer more extensive applications. While retaining biocompatibility and biodegradability, chemical modification can produce a more mechanically and chemically robust material. Methods detailing the synthetic alteration of HA and the resulting applications in cosmetic, medical, and pharmaceutical applications have been reviewed previously (Larsen and Balazs Citation1991; Denlinger Citation1998; Band Citation1998; Lapcik et al. Citation1998; Vercruysse and Prestwich Citation1998; Adams et al. Citation2000; Moreland Citation2003; Campoccia et al. Citation1998) and are not included in this article. The purpose of our review is to describe the physical, chemical, and biological properties of native HA together with details of its medical and pharmaceutical uses.
PHYSICAL AND CHEMICAL PROPERTIES OF HA
Chemical Structure and Stability
The precise chemical structure of HA, which comprises repeating units of D-glucoronic acid and N-acetyl-D-glucosamine, was first determined by Weissman and Meyer (1954). Further studies (Laurent Citation1970; Laurent and Fraser Citation1992) have shown the primary structures of the polysaccharide to be an unbranched linear chain with the monosaccharides linked together through alternating β1,3 and β1,4 glycosidic bonds (Scott and Heatley Citation1999).
Data obtained from viscosity measurements, light scattering, and ultracentrifugation suggest that HA molecules assume an expanded random coil structure in solution (Lapcik et al. Citation1998). However, these techniques only provide a measure of the bulk properties and thus are indicative of the mean contribution of a large number of molecules within a polydispersive distribution and do not necessarily provide molecular information. However, nuclear magnetic resonance spectroscopy (NMR), X-ray diffraction, and molecular modeling all provide evidence of the existence of ordered structure in HA molecules (Lapcik et al. Citation1998; Scott Citation1984; Scott and Heatley Citation1999; Day and Sheehan Citation2001). The data obtained from these techniques suggest that up to 5 hydrogen bonds exist between two neighboring disaccharide units and the secondary structures are formed as tape-like 2-fold helices by twisting each disaccharide unit through 180°. In addition, secondary hydrophobic faces exist within the structure, formed by the axial hydrogen atoms of about 8 CH groups on the alternating sides of the secondary structure. Such hydrophobic patches, in turn, energetically favor the formation of meshwork-like β -sheet tertiary structure as a result of molecular aggregation (). The tertiary structure is stabilized further by the presence of intermolecular hydrogen bonding (Scott and Heatley Citation1999). The hydrophobic and hydrogen bonding interactions, countering electrostatic repulsion, enable large numbers of molecules to aggregate leading to the formation of molecular networks (matrices) of HA ().
FIG. 1 The primary, secondary, and tertiary structures of HA in solutions. (A) The primary structure of HA consists of repeating dissacharide units of D-glucoronic acid and N-acetyl-D-glucosamine with up to 5 hydrogen bonds existing between each two neighboring disaccharides. The secondary structures are formed as tape-like 2-fold helices by twisting each disacchride unit through 180° compared with those ahead and behind it in the chain. (B) The β -sheet tertiary structure tertiary structure is energetically stabilized by interactions between hydrophobic patches hatched and intermolecular hydrogen bonding between the acetamido ▪and □ and carboxylate groups (• and ○). (C) Schematic networks of HA molecules as a results of intermolecular aggregation (Reprinted with permission from Scott and Heatley Citation1999).
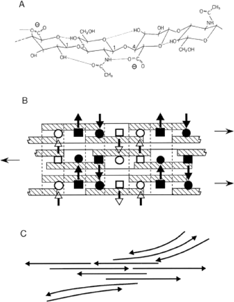
Such an ordered and rigid structure is more stable than any random state that may exist in solution although it does possess varying degrees of flexibility and may undergo interconversion between different conformation states. For example, Scott and Heatley (Citation2002) reported that the stability of the tertiary structure of HA appeared to be marginal in physiological solutions. In this study, the mesh-like HA molecules were susceptible to reversible de-aggregation, with changes to an unordered structure (denaturation) occurring in response to cooling, warming, or pH. Such denaturation was irreversible upon enzymatic degradation and chemical modification. The delicate equilibrium between ordered and unordered structure is assumed to be a consequence of steric, hydrophobic, and particularly hydrogen bonding interactions, which occur between HA chains and water, although detailed dynamics in molecular terms have yet to be elucidated.
Apart from the inherent instability of highly ordered secondary and tiertary structures, the primary structure of HA also is susceptible to degradation under various conditions, including mechanical, ultrasonic, pH, thermal, free radical, and enzymatic stresses (Lapcik et al. Citation1998). The dependence of HA's stability on pH has been reported by Tokita and Okamoto (Citation1995) who showed that HA possesses the highest stability at neutral pH, whereas they found it to be more labile in acidic solution than basic and to be less stable at higher temperatures. In general, the degradation of a HA solution under pH, thermal, and free radical stresses has been reported to occur randomly (Reed and Reed Citation1989; Tokita and Okamoto Citation1995; Vercruysse et al. Citation1995; Lowry and Beavers Citation1994). In contrast, the depolymerization that occurs following ultrasonic treatment has been reported to occur in a nonrandom manner (Hawkins and Davies Citation1996) and to be mechanical in origin (Basedow and Ebert Citation1977; Miyazaki et al. Citation2001).
Mechanical depolymerization of HA also has been demonstrated to occur using a rotational viscometer, where the molecular weight of HA in a diluted solution was found to decrease with increasing shear stress (Miyazaki et al. Citation1998). In this study, the authors found that the position of the cleavage of the HA chain depended upon the shear rate loaded and ionic strength. The same authors utilized ultrasonication in specific ionic conditions to depolymerize HA to form controlled molecular weights (Miyazaki et al. Citation2001). The degradation of HA solution induced by stresses is of pharmaceutical relevance. For example, upon freeze-drying, HA has been reported to be susceptible to depolymerization (Pigman et al. Citation1961; Wedlock et al. Citation1983; Doherty et al. Citation1994; Tokita et al. Citation1997). During the freeze-drying process, the stability of HA depends upon the form of HA employed, with sodium HA being more stable than sodium-free HA. The instability of HA has been attributed to the production of free radicals during this process and can be minimized by the presence of free radical scavengers (Wedlock et al. Citation1983; Tokita et al. Citation1997).
Physicochemical Properties
HA and its associated networks have many physiological roles that include tissue and matrix water regulation, structural and space-filling properties, lubrication, and a number of macromolecular functions (Balazs Citation1998). Changes in the ordered structures of HA can lead to major alterations in its physicochemical (e.g., viscoelastic and mechanical) properties since they are largely dominated by the nature of the secondary and tertiary structures. In the solid state when prepared at a low pH, HA has been found by X-ray diffraction to possess double helical structures (Arnott et al. Citation1983) that are markedly different from the structures of HA formed in aqueous solution. The effect of water on the structure and dynamics of HA is a consequence of the strong hydrogen-bonding that exists between the two molecular species. These interactions are illustrated by the highly inhibitory effect on the freezing of water conferred by 0.5 and 3.0% w/w HA in aqueous solution, as determined by modulated differential scanning calorimetry. Results have shown that 1 g of HA could lead to the presence of about 3.65 g of nonfreezing water during the freezing process (Liu and Cowman Citation2000).
The number of hydrogen bonds between water molecules and the hydrogen bond accepting atoms of HA has been calculated using molecular dynamic simulations to be between 10 and 15 per disaccharide unit of HA (Kaufmann et al. Citation1998). As such, when compared with such other polysaccharides as alginate, carrageenan, guar gum, locust bean gum, and tragacanth gum, HA has a much higher capacity to bind water (Budiaman and Fennema Citation1987; Cowman et al. Citation1998).
HA in aqueous solution has been reported to undergo a transition from Newtonian to non-Newtonian characteristics with increasing molecular weight, concentration, or shear rate (Gribbon et al. Citation2000). In addition, the higher the molecular weight and concentration of HA, the higher the viscoelasticity the solutions possess (Gibbs et al. Citation1968; Gribbon et al. Citation2000; Karim et al. Citation1996; Kobayashi et al. Citation1994; Fujii et al. Citation1996). The networks of gelling HA are initially formed by weak interactions between HA chains and these can be readily disrupted upon increasing shear rate leading to shear-thinning (Gribbon et al. Citation2000). The viscoelasticity of HA in aqueous solution also is affected by the inclusion of a small molecular agent (Kobayashi et al. Citation1994; PasqualiRonchetti et al. Citation1997; Mo et al. Citation1999; Ghosh et al. Citation1994). For example, the presence of phospholipids, guanidine, and sodium chloride leads to decreases in both elastic and viscous moduli while the addition of sugars appears to promote structure.
The effect of these compounds on the viscoelasticity is due to the disruption of intermolecular interactions and reduction in the entanglement between HA molecules. Guanidine and sodium chloride act by shielding electrostatic repulsion between HA molecules (Mo et al. Citation1999) whereas phospholipids provide competition for the hydrophobic binding between HA molecules (Ghosh et al. Citation1994). When sugars such as sucrose or glucose were added to HA solutions, both the radius of gyration and hydrodynamic radius of HA were reduced such that the interactions between HA molecules were enhanced; this resulted in an increase in the storage and loss of moduli (Kobayashi et al. Citation1994; Mo et al. Citation1999).
The viscoelastic properties of HA in aqueous solution are pH-dependent. HA has a pKa value of about 3.0 and therefore a change in pH will affect the extent of ionization of the HA chains. A shift in ionization affects the intermolecular interactions between the HA molecules which changes the rheological properties of the compound. Gibbs et al. (Citation1968) found that HA in solution possesses entirely different viscoelastic properties at pH 2.5 compared with those at either pH 1.5 or 7.0 with the elasticity be higher at pH 2.5 than at either 1.5 or 7.0 (Gibbs et al. Citation1968). In physiological solution, the carboxylic groups within D-glucuronic acid of HA are completely ionized with the charges being sited about 1 nm from each other. However, the charges on HA chains are sensitive to specific ionic conditions, which may cause alterations in the bulk solvents, and the inter and intramolecular interactions. For example, with the addition of different ions (Ca2 +, Mn2 +, Na+, K+, and Mg2 +), the visocoelasticity of HA solutions was different because of the different effects conferred by the ions on the HA chain flexibility (Sheehan et al. Citation1983; Gribbon et al. Citation1999; Gribbon et al. Citation2000).
HA in combination with other glycosaminoglycans (GAGs) such as dermatan sulphate (Koshiishi et al. Citation1999), chondroitin sulphate, and keratin sulphate (Poggi et al. Citation1999) are prominent in tissues such as the skin. HA is found to exist together with protein cores (aggrecans) to which the other members of the GAG family are attached. As its name implies, aggrecan is composed of very large proteoglycan aggregates. The most important property of these molecules is their ability to bind to water and this induces the proteoglycans to become hydrated to such an extent that a gel-like system is formed. The networks of proteoglycan-HA aggregates shift the Newtonian region to lower shear rates and these gels have an increased dynamic viscoelasticity relative to HA-HA networks.
Apart from the unique rheological properties, HA solutions exhibit mucoadhesive properties (Saettone et al. Citation1989; Durrani et al. Citation1995; Pritchard et al. Citation1996; Lim et al. Citation2000). Saettone et al. (Citation1989) and Durrani et al. (Citation1995) found that the degree of mucoadhesion exhibited by HA was both pH- and molecular-weight dependent. Increasing the molecular weight of HA from 134,000 to 4 million Da or decreasing pH from neutral to acidic was found to promote adhesion.
Biological Properties
HA is found in almost all vertebrate organs, but most abundantly in the extracellular matrix of soft connective tissues. HA is particularly abundant in mammalian skin where it constitutes a high fraction of the extracellular matrix of the dermis (Juhlin Citation1997; Tammi et al. Citation1994). The estimated total amount of HA in human skin has been reported to be 5 g (Banks et al. Citation1976), about one-third of the total amount of HA believed to be present within the entire human body (Laurent Citation1995). HA is present in both the dermis (∼ 0.5 mg/g wet tissue) and the epidermis (∼ 0.1 mg/g wet tissue). Interestingly, while the dermis consists primarily of extracellular matrix with a sparse population of cells, the epidermis is the reverse; the keratinocytes fill all but a small percentage of the tissue. The actual concentration of HA in the matrix around the cells in the epidermis (estimated to be 2-4 mg/ml) is an order of magnitude higher than in the dermis (estimated to be ∼ 0.5 mg/ ml). Thus, the matrix around keratinocytes may have a HA concentration as high as that in umbilical cord (∼ 4 mg/ml). In addition, Sakai et al. (Citation2000) have shown that HA is derived from keratinocytes beneath the stratum corneum and is present in the normal stratum corneum (Sakai et al. Citation2000).
HA biosynthesis occurs at the plasma membrane under control of HA synthase (Has) that has 3 isoforms: Has1, Has2, and Has3 (Itano and Kimata Citation2002). In addition, apart from the polymerization of HA chains on the inner face of the cell membrane, the proteins also are responsible for the extrusion or translocation of the polymer through the membrane to the extracellular face of the cell. Although any of the Has proteins are sufficient for HA production, the three isoforms possess different enzymatic properties including kinetic characteristics and the size of the HA produced. Has1 and Has2 proteins are responsible for the synthesis of high molecular weight HA (∼ 2 × 106 Da) with the latter more active catalytically than the former, whereas Has3 protein possesses the highest activity but polymerizes low molecular weight HA (< 3 × 105 Da) (Spicer and Nguyen Citation1999). The different molecular weight of HA chains can lead to different effects on cell behavior.
The turnover of HA has been found to be as important as its synthesis since the balanced metabolism of HA contributes to its physiological functions (Lee and Spicer Citation2000). In humans, the half-life of HA in tissues ranges from about 1 day in the epidermis (Tammi et al. Citation1991) up to 70 days in the vitreous body of the eye (Fraser et al. Citation1997). HA turnover within a tissue occurs either by local degradation or release from the tissue into the lymphatic/vascular systems. Generally, the removal of HA from the tissues takes place within the lymph nodes after entering the lymphatic system, where about 10% of the HA is transported to the circulation and cleared in the liver or the kidney (Fraser et al. Citation1998). The degradation of HA within the lymph nodes uses receptor-mediated endocytosis and at least two receptors, hyaluronan receptor for endocytosis (HARE) (Zhou et al. Citation2000) and LYVE (lymphatic vessel endothelial HA receptor [Banerji et al. Citation1999; Prevo et al. Citation2001]), have been identified as being involved.
The main clearance of circulating HA, either intravenously injected or transported from the lymphatic system, takes place rapidly in the liver also via receptor-mediated endocytosis resulting in a HA half-life of less than 10 min in humans (Lebel Citation1991). In contrast, the degradation of HA in the kidney is achieved via cellular uptake and lysosomal degradation by specific enzymes in an acid cellular compartment (Wuthrich Citation1999; Knudson et al. Citation2002). Local turnover of matrix HA within the tissues generally occurs again through receptor-mediated endocytosis via the action of a specific binding protein, CD44 (Menzel and Farr Citation1998). However, some may depolymerize to short HA chains through the action of hyaluronidase or chemical free radicals (Ng et al. Citation1992). Once internalized via CD44 binding, HA is degraded mainly within lysosomes to small fragments.
Many physiological functions of HA are thought to relate to its molecular characteristics, including its physiochemical properties, its specific interactions with hyaladhereins, such as CD44 and RHAMM (receptor for HA-mediated motility), and its mediating effect on cell signaling and behavior (Tool Citation2001). HA in physiological solution can act as an osmotic buffer, space filler, lubricant, and molecular sieve and play a role in maintaining the water content (Fraser et al. Citation1997). Small molecules such as water, electrolytes, and nutrients can freely diffuse through the pores formed within a solution/gel network while macromolecules such as large proteins and pathogens are partially excluded from the domain. However, networks of HA chains are highly dynamic in nature and constantly move in solution, leading to continuous changes in pore size. Thus, in certain situations even large macromolecules such as proteins have the potential to pass through a HA network. Water homeostasis is attributable to the strong water-HA interaction, which may be responsible for the regulation of moisture content in the epidermis/stratum corneum (Sakai et al. Citation2000).
Recently, an understanding of the role of HA in the mediation of physiological functions via interaction with binding proteins and cell surface receptors has emerged. The involvement of HA in many biological processes, including morphogenesis, regeneration, the regulation of cell signaling and behavior, wound healing, and tumor invasion have been described in numerous recent reviews (Cichy and Pure Citation2003; Ponta et al. Citation2003; Tammi et al. Citation2002; Day and Prestwich Citation2002; Turley et al. Citation2002; Toole et al. Citation2002). For example, HA binding to CD44 has been reported to lead not only to the removal of the polymer from the tissues, but also the initiation of the signaling cascade that contributes to cell motility and proliferation. However, the exact mechanisms by which different signals are activated have not yet been defined completely (Goodison et al. Citation1999; Tammi et al. Citation2002). In addition, the interactions of HA with RHAMM have been reported to mediate cell migration and proliferation (in both normal and tumor cells) along with being involved in the modulation of acute and chronic inflammation processes in man (Turley et al. Citation2002).
ANALYSIS OF HA
Quantification
The lack of an ultraviolet (UV) or fluorescent chromophore (Toida et al. Citation1997) within HA's native structure, its large molecular size, and wide molecular weight distribution make accurate and sensitive quantification of this molecule problematic. With the exception of Grimshaw et al. (Citation1994) who directly detected HA from the eye vitrious humor using low wavelength UV detection, the majority of reported chromatographic and colorimetric methods conjugate, react, or lyse HA to facilitate its analysis.
Conjugation
The European Pharmacopoeia (Citation2002) describes a simple colorimetric assay in which the glucuronic acid content of the polysaccharide is determined using a reaction with carbazole. This method was originally adapted from the work of Bitter and Muir (Citation1962). The carbazole modified HA absorbs UV light at 530 nm and produces a linear calibration in the range 6.5–65 μ g g− 1. A molar ratio calculation is subsequently used to determine the HA concentration.
Although colorimetric analysis is very simple and quick to perform, it can be affected by impurities and therefore chromatographic methods are more suitable for the analysis of complex mixtures containing HA (Asteriou et al. Citation2001). Platzer et al. (Citation1999) highlighted this problem when they compared capillary electrophoresis (CE) and the carbazole reaction to quantify HA within simple gel formulations. CE was capable of resolving the intact polysaccaride from the formulation excipients and produced a linear calibration curve (r2 > 0.999) over a 0.01–5 mg ml− 1 concentration range. Compared with the colorimetric assay, CE recovered significantly more of the polysaccaride from the gel formulations and furthermore was capable of quantifying the preservative in the formulations, p-hydroxybenzoic acid.
Gel filtration commonly is used to quantify HA in its native form. Chaidedgumjorn et al. (Citation2002) used suppressed conductivity detection to detect both HA polysaccarides and oligomers down to 6.78 ng (100 K Mw) on two coupled TSKgel G3000SWXL columns. However, this method was not capable of fully resolving a mixture of intact HA polysaccharides over a 70-min run time. Toida et al. (Citation1997) proposed combining HA with copper (II) to enhance its analysis. This group coupled GFC (HW40F, Toyo-Pear) with UV detection as the copper provided HA with optical properties. A highly resolved HA-copper(II) peak was eluted within 12 min, however, the effects of HA's molecular weight, polydispersity, or interfering analytes on the copper(II)-HA complex were never investigated in this study.
Digestion
Digesting HA into its constitutional disaccharide units prior to quantification offers analytical advantages in terms of detection sensitivity. Volpi (Citation2000) used chondroitin sulphate lyases to convert HA into unsaturated disaccharides that can be resolved and quantified using high performance liquid chromatography (HPLC). This method used flourimetric derivatization with dansylhydrazine originally described by Shinomiya et al (Citation1989). Volpi (Citation2000) completely separated the disaccarides as a result of the HA digestion and detected down to 50 picomol. Karamanos and Hjerpe (Citation1997) have since reported detection limits for unsaturated disaccharides using similar CE methodology within the attomole range.
Molecular Weight and Molecular Weight Distribution
HA is a homogenous polysaccharide generally with a very high molecular mass. However, in vivo its continual turnover can lead to the existence of oligosaccarides and even smaller molecular weight fragments. Therefore, HA most commonly exists as a population of polysaccharide chains with a wide range of molecular weights (105–107) (Kakehi Citation2003). Both the average molecular weight and the molecular weight distribution of HA can influence many of its physicochemical properties, e.g., its viscoelastic nature (discussed previously). This has lead to the classification of the commercially produced material into more defined molecular weight fractions compared with the wide distributions found in vivo, thus allowing grading the materials purity, physical, and chemical characteristics.
The importance of molecular weight to HA has led to several techniques developed to measure both the average molecular weight and distribution of HA. These include sedimentation, ultracentrifugation (Laurent and Gergely Citation1955; Ogston and Stainer Citation1950, Citation1951, Citation1952), light scattering (Reed Citation1989; Blumberg Citation1954; Laurent Citation1960), diffusion (Laurent Citation1960), osmometry, gel electrophoresis, viscometry, and chromatography. However, only the most commonly employed techniques, gel electrophoresis, chromatography, and viscometry are discussed in depth in this review.
Gel Eletrophoresis
Gel eletrophoresis can be used to determine both the molecular weight and molecular weight distribution of HA, although it is only possible to attain qualitative or semiquanitative data using this method. The earliest methods of gel electrophoresis used cellulose acetate membranes and Alcain blue staining (Seno et al. Citation1970). However, high porosity agarose gels are now more commonly used as illustrated by Lee and Cowman (Citation1994) This group separated HA from human knee joint synovial fluid using a nonspecific cationic dye Stains-All (3, 3′ -dimethyl-9-methyl-4, 5, 4′, 5′ -dibenzothiacarbocyanine) as a marker for HA detection. High molecular weight HA standards (characterized by light scattering) were used as molecular weight markers and the samples were run on a 0.5% agarose gel. In this method loading 4 μ g of HA (< 0.5 mg/ml) allowed separation of very high molecular weight HA samples up to 6 × 106 Da. The principles from Lee and Cowmans work have been further developed by several other groups (Armstrong and Bell Citation2002; Volpi and Maccari Citation2002). In addition, How and Long (Citation1969) characterized the polydispersity of HA from human synovial fluid using electrophoresis by fractionation of the 0.1% agarose gels. Additional details on the electrophoretic analysis of HA, chemically modified HA, and HA oligomers are discussed in a review by Kakehi (Citation2003).
Chromatography
Estimation of molecular weight of HA samples using gel filtration chromatography (GFC) or size exclusion chromatography requires the use of molecular weight standards such as polyethylene oxides (Beaty Citation1985), dextrans (Praest Citation1997), and pullulans (Iqbal Citation1997) because HA standards having well-defined molecular weights and narrow molecular weight distributions are not commonly available. Yeung and Marecak (Citation1999) question the use of polymer standards such as pullulans or dextrans to generate a relationship between retention volume and log of molecular weight because of the unique solution conformation of HA.
HA's polydisperse nature has precluded its direct molecular mass analysis using matrix-assisted laser desporption ionization mass spectroscopy (MALDI-MS) because the technique underestimates the molecular weight of such molecules (Jackson et al. Citation1996). However, Adam and Ghosh (Citation2001), have suggested that coupling gel filtration chromatography with MALDI-MS can resolve this problem. These researchers showed that the weight average molecular weight (Mw) and number average molecular weight (Mn) obtained for 6 of the 7 standards using preseparation with GFC were in good agreement with the reported values. Another novel molecular weight assessment assay for HA is anionic exchange chromatography. Karlsson and Bergman (Citation2003) reported the molecular weight distribution of HA samples in the range of 0.1 × 106 to 5 × 106 Da using this technique.
Viscometry
Static off-line bulb tube viscometry does produce reliable dynamic viscosity measurements across a wide range of HA samples (Mendichi et al. Citation1998). However, bulb viscometry can be cumbersome and unsuitable for quality control. Therefore, the viscosity average molecular weight (Mv) is more frequently determined for HA, which is derived from the intrinsic viscosity [η] using the following Mark-Houwink equation
Both Karim, Citation1996 and Laurent, Citation1960 describe HA molecular weight characterization using viscometry. The measurement was carried out over a range of concentrations (for example, 0.15–5.6 × 10− 3 g/ml) and intrinsic viscosity was estimated using Huggins and Kraemer plots. Values of K and α have been determined for HA in both 0.2 M and 0.5 M NaCl by Cleland and Wang (Citation1970). Unsurprisingly, values of K and α depended on the ionic strength (Shimada and Matsumura Citation1975; Cleland and Wang Citation1970) and the pH (Balazs, Cowman, and Briller Citation1983) of the HA solutions. Gura et al. (Citation1998) also showed that the coefficients K and α are affected by the magnitude of shear applied to the material. This work postulated that large molecular weight HA samples require a low shear viscometer such as the Zimm-Crothers machine to attain accurate coefficient values, as the onset of shear-dependent viscosity shifts to a lower shear rate due to the re-enforced entanglement of the intermolecular domains in high weight HA (Gura et al. Citation1998).
MEDICAL APPLICATIONS OF HA
Treatment of Osteoarthritis
Under normal physiological conditions, HA is one of the principal components of synovial fluid present at concentrations between 1.4–4 mg/ml (Sundblad Citation1965). The viscoelasticity of the HA matrix is responsible for conferring lubrication and mechanical support to joints. However, the molecular weight of HA in osteoarthritic joints can decline to 0.5 million Daltons (from 4–5 million Daltons in the normal joints) as a result of the presence of proinflammatory cytokines, free radicals, and proteinases. In addition, the membrane permeability of the synovial vasculature increases in response to inflammation or injury, leading to increases in the infiltration of plasma fluids and in turn decreases in concentration of HA in the joints. The decrease in the concentration and/or the molecular weight of HA can result in changes in the viscoelasticity of the synovial fluid, leading to joint dysfunction. Therefore, the rationale for injecting purified high molecular weight HA into the joints is to restore the desirable rheological properties, alleviating some of the symptoms of osteoarthritis (Balazs and Denlinger Citation1993, Citation1989).
Intra-articular injection of HA is approved for the treatment of osteoarthritis in many countries including the USA, Canada, Japan, and some parts of the European Union. Commercially available products for this application contain HA with different molecular weight distributions. For example, the molecular weights of Hyalgan® (Fidia, Italy), Artz® (Seikagaku, Japan), and Orthovisc® (Anika, USA) are 0.5–0.73, 0.6–1.2, and 1.0–2.9 million Daltons, respectively. A summary of the commercial products that use HA can be found in .
TABLE 1 Summary of the medical applications of HA
The therapeutic effects of HA injection products on osteoarthritic joints have been extensively investigated in vitro, in vivo, and clinically (e.g., Hochberg Citation2000; Altman Citation2000; Dougados Citation2000; Guidolin et al. Citation2001; Maheu et al. Citation2002; Barrett and Siviero Citation2002; Miltner et al. Citation2002; Tascioglu and Oner Citation2003; Uthman et al. Citation2003; Kelly et al. Citation2003; Hamburger et al. Citation2003). Results from these studies indicated that HA confers pain relief and functional improvements. The analgesic effect of viscoelastic HA was found to be comparable or superior to either that obtained after the administration of either corticosteroids (Altman Citation2000; Kirwan Citation2001; Guidolin et al. Citation2001) or nonsteroidal anti-inflammatory drugs (NSAIDs) (Hochberg Citation2000). However, the mechanism by which HA confers therapeutic effects on osteoarthritic joints appears to be unclear (Ghosh and Guidolin Citation2002) despite the postulation of Balazs and Denlinger (Citation1993) that it acts as a viscosupplement, the reason it was first utilized in intra-articular injections. In the short term, HA can confer mechanical and lubricating effects, independent of the variation in the molecular weights of marketed HA preparations (Mabuchi et al. Citation1999; Balazs Citation2003) such that symptomatic relief can be elicited. The controversy about the role of viscoelasticity in the efficacy of HA for the treatment of osteoarthritis results from the rapid clearance of HA from the joint relative to its long duration of therapeutic effectiveness (Ghosh and Guidolin Citation2002).
The benefit from HA injections after a few weeks therapy has been reported to last up to 2 months (Altman Citation2000), which is inconsistent with the rapid local clearance of HA. In vivo studies found that the half-lives of injected HA into the normal joint knees were less than 1 day (Laurent and Fraser Citation1992), whereas those into the osteoarthritic joints were even shorter (Fraser et al. Citation1993). These results suggest that HA might confer its clinical benefits at least partly due to pharmacological effects. The pharmacological role of HA may be related to its anti-inflammatory and immunoregulatory properties, which are dependent upon its molecular weight, although detailed mechanisms by which the clinical benefits occur are yet to be established (Ghosh and Guidolin Citation2002; Zhu and Granick Citation2003).
Surgery and Wound Healing
HA is used routinely in eye surgery to facilitate the implantation of artificial intraocular lens, and commercially available products include Healon®, Opegan®, and Opelead®. HA also is used in surgical procedures as a viscoelastic gel (for example, Bionect®, Connettivina®, and Jossalind®) to aid healing and the regenerative processes of surgical wounds (reviewed in Balazs and Denlinger Citation1993; Lapcik et al. Citation1998; Ghosh and Jassal Citation2002). The applications of HA in eye surgery mainly is due to its viscoelasticity; its high viscosity at low shear enables the HA solution to be maintained in the operative space, while the shear thinning behavior assists both the protection of ocular cells from damage (acting as a lubricant) and ensures its ease of injection and removal (Balazs and Denlinger Citation1993; Risberg Citation1997).
Apart from acting solely as a viscoelastic substance, HA appears to possess a range of biological activity since it promotes corneal (Inoue and Katakami Citation1993; Miyazaki et al. Citation1996; Stiebel-Kalish et al. Citation1998; Tani et al. Citation2002), diabetic foot (Vazquez et al. Citation2003), nasal mucosal (Soldati et al. Citation1999), and venous leg ulcer (Ortonne Citation1996) wound healing. Miyazaki et al. (Citation1996) suggested that the corneal wound healing effect conferred by HA is partially due to its ability to promote the growth of corneal epithelial cells. In addition, HA also has been reported to decrease neutrophil elastase-induced alveolar injury after inhalation (Cantor et al. Citation1998). Aerosolization of 0.1% w/v HA solution to Syrian hamsters for 50 min significantly reduced neutrophil elastase-induced alveolar injury of the animals relative to that found in the animals exposed to aerosolized water alone. The authors suggested that the protective mechanism was due to specific binding between HA and lung elastic fibers (Cantor et al. Citation1998). However, the detailed molecular biological mechanism by which HA facilitates wound healing has yet to be identified (Turino and Cantor Citation2003).
Embryo Implantation
HA has been reported to be a suitable replacement for albumin in culture media for in vitro fertilization (Simon et al. Citation2003; Gardner et al. Citation1999). The utilization of albumin (human serum albumin for human use) in culture media during in vitro fertilization is not only due to its ideal physical properties such as viscosity, but also because it allows easy handling of the embryo, by preventing it adsorbing to the surface of the culture dish (Simon et al. Citation2003). However, blood-derived albumins may be contaminated with prions and viruses, leading to potential disease transmission. As a result, the utilization of a functional albumin substitute is now preferred (Vanos et al. Citation1991; Kemmann Citation1998). HA, as an endogenous component present in the human uterus at a high level (Suchanek et al. Citation1994), has been found to be compatible with animal and human embryos. The presence of HA as a substitute of albumin has not resulted in any detrimental effects on the development of frozen-thawed mouse and sheep embryos (Joly et al. Citation1992). In addition, the presence of HA in culture media could act as a cryopreservative to human embryos by maintaining their viability during thawing (Gardner et al. Citation2003).
More studies have investigated the effect of HA in murine (Gardner et al. Citation1999; Lane et al. Citation2003; Figueiredo et al. Citation2002), porcine (Miyano et al. Citation1994; Kano et al. Citation1998; Abeydeera Citation2002), and bovine (Jaakma et al. Citation1997; Furnus et al. Citation1998; Jang et al. Citation2003) embryo development and viability after culture in HA-based media. Such studies have demonstrated no differences between HA and albumin with regard to the efficiency of in vitro blastocyst production from in vitro-matured oocytes, and they have thus opened the possibility of including HA in culture media as a substitute of albumin. In a recent clinical study, Simon et al. (Citation2003) found that HA-based human embryo transfer medium leads to a comparable pregnancy and implantation rate when compared with albumin based medium (clinical pregnancy and implantation rates of 62.5% and 34% using the former versus 52% and 26.8% using the latter). summarizes both the medical applications and the commonly used commercial preparations containing HA used within this field.
HA IN DRUG DELIVERY
Dermal Drug Delivery
The physiological function of the stratum corneum, the outermost and nonviable layer of the skin, is to act as a protective barrier for the body and as such it is particularly effective at preventing the permeation of drug molecules into and across the skin for dermal (local) and transdermal (systemic) delivery. Although extensive studies are being performed into the latter, relatively few investigations have been conducted into the development of delivery systems for drug targeting to the skin, although HA is one such system that receiving increasing attention.
However, recently a formulation marketed as Solaraze was approved in the United States, Canada, and most of the European countries for the topical treatment of actinic keratoses (AK), the third most common skin complaint in the U.S. (Fieldman et al. Citation1998; Moy Citation2000; Del Rosso Citation2003; Jarvis and Figgitt Citation2003). AK, relatively common skin lesions that result from excessive sun exposure, are most common on the scalp, face, and/or dorsum of the hands in light-skinned persons aged ≥ 50 years, particularly those with a history of occupational exposure to the sun. AK now are considered to be synonymous with squamous cell carcinoma (SCC) in situ with growing evidence that AK and SCC lie on a clinical, histological, cytological, and molecular continuum (Schartz Citation1997).
Although the exact mechanism of action of diclofenac in the Solaraze® formulation remains unclear, it has long been established that some NSAIDs have an antitumorigenic effect (Panie Citation1981; Braun et al. Citation1989; Falk et al. Citation1992) that may be attributable to reduced PGE2 synthesis. PGE2 is known to attenuate cell-mediated immunity; in particular this prostaglandin inhibits natural killer cell cytotoxicity (Brunda et al. Citation1980), mitogen-induced lymphocyte proliferation (Tilden and Balch Citation1982), and macrophage proliferation and antitumor cytotoxicity (Elliott et al. Citation1988).
The beneficial effect of HA on the dermal delivery of diclofenac also has been rigorously investigated in vitro, in vivo, and in clinical studies (Brown et al. Citation2002, Citation1995a, Citation1995b, Citation1995c; Brown and Martin Citation2001; McEwan and Smith Citation1997; Wolf et al. Citation2001; Nazir et al. Citation2001; Brown and Moore Citation1996; Lin and Maibach Citation1996). Brown et al. Citation2002, Citation1995c and Brown and Martin (Citation2001) have shown in vitro that HA enhances significantly both the partitioning of diclofenac into human skin and its retention and localization in the epidermis when compared with an aqueous control, other glycoseaminoglycans, and other commonly used pharmaceutically acceptable gelling agents (). Such results have been confirmed in other studies in which HA was shown to minimize the percutaneous absorption of diclofenac (McEwan and Smith Citation1997; Wolf et al. Citation2001; Brown et al. Citation1995b) indicating the formation of a depot or reservoir of drug in the epidermis (Brown et al. Citation1995a; Lin and Maibach Citation1996). These data have been supported by preclinical studies where radiolabelled HA was found not only to penetrate the skin of nude mice and humans, but also to aid the transport of diclofenac to the epidermis (Brown et al. Citation1999). HA also has been reported to produce similar effects with ibuprofen (Brown et al. Citation2002; Brown and Martin Citation2001), clindamycin phosphate (Amr Citation2000), and cyclosporin (Brown and Moore Citation1996; Nazir et al. Citation2001).
Ophthalmic Drug Delivery
Topically applied drugs into the eye generally have low bioavailability with only a small fraction of the instilled dose remaining on the target tissue of the eye (Jarvinen et al. Citation1995). The precorneal clearance of the drugs is due to a rapid drainage, resulting in considerable loss of the drugs via the nasolachrymal duct, combined with conjunctiva absorption. After clearance, drugs can reach the circulation, resulting in systemic side effects (Sasaki et al. Citation1996). Therefore, it is desirable to prolong the ocular residence of drugs and consequently to enhance local bioavailability.
HA has been used as a vehicle for topical ophthalmic drugs due to its viscoelasticity and mucoadhesive capacity (Lapcik et al. Citation1998; Kaur and Smitha Citation2002). Numerous studies have reported that HA is capable of prolonging the precorneal residence and/or increasing the bioavailability of pilocarpine (Gurny et al. Citation1987; Camber and Edman Citation1989; Camber et al. Citation1987, 1994; Saettone et al. Citation1989, Citation1991, Citation1994; Bucolo and Mangiafico Citation1999; Bucolo et al. Citation1998), tropicamide (Saettone et al. Citation1989; Herrero-Vanrell et al. Citation2000), timolol (Bucolo et al. Citation1998), gentimycin (Moreira et al. Citation1991a, Citation1991b; Bernatchez et al. Citation1993), tobramycin (Gandolfi et al. Citation1992), arecaidine propargyl ester (Langer et al. Citation1997) and S-aceclidine (Langer et al. Citation1997) in solution form. The mucoadhesion together with the increased structure conferred by HA are thought to be primarily responsible for the prolonged precorneal residence of the drugs. HA binds to the layer of natural mucin on the corneal epithelium (Saettone et al. Citation1994, Citation1989) and its shear thinning behavior provides no resistance to blinking, and thus results in good patient compliance.
Nasal Delivery
HA has been used as a component of vehicles for the nasal delivery of small molecular drugs and peptides with a view to increasing bioavailability as a result of bioadhesion and/or penetration enhancement (Morimoto et al. Citation1991; Lim et al. Citation2000, Citation2002; Castellano and Mautone Citation2002). Castellano and Mautone (Citation2002) found that HA could be incorporated into a topical formulation of xylometazoline that provided effective relief of nasal congestion. In addition, Morimoto et al. (Citation1991) reported that the bioavailabilities of nasally administered vasopressin and 1-deamino-8-D-arginine vasopressin in rats were increased more than 2-fold and 1.6-fold, respectively, when HA was in the formulation relative to the bioavailability attained in the polymers absence. The increases conferred by HA were found to be molecular-weight dependent with the high molecular weight HA fractions (more than 300 kDaltons) promoting an increase in bioavailability while low molecular weight HA (55 kDaltons) had no effect. The authors attributed the increases in bioavailability to the penetration enhancement induced by HA since in vitro, the ciliary beat frequency of rabbit nasal mucosal membranes appeared not to be affected by the presence of HA in solution (Morimoto et al. Citation1991).
However, the incorporation of HA into microparticulate delivery systems confers mucoadhesive properties (Lim et al. Citation2000, Citation2002). HA-contained microspheres were found to be markedly more mucoadhesive than those of chitosan-based microparticulates. In addition, the adhesive forces appeared not to be affected by the presence of a model drug, gentamicin (Lim et al. Citation2000). The bioavailability of intranasally administered gentamycin using HA-based microspheres (23.3% relative to intravenous dosing), although significantly higher than that obtained using drug solution (1.1%) or drug particles (2.1%), was not as high as that using chitosan-based particles (31.4%). The highest bioavailability (42.9%) achieved via intranasally administered particles was obtained using HA/chitosan microspheres (Lim et al. Citation2002). Therefore, researchers assumed that HA mainly acted as a mucoadhesive agent whereas chitosan had a major penetrating enhancement function with the combination producing a synergistic effect on drug absorption.
Pulmonary Drug Delivery
Protein and peptide drugs generally are delivered parenterally. However, clinical use of these drugs requires repetitive injections, which makes noninvasive dosing more desirable. As a result, pulmonary delivery of proteins and peptides has been extensively investigated with a view to eliciting systemic therapeutic effects. HA has been included as a component of an inhaled formulation of insulin to enhance the absorption of the hormone and achieve a sustained release (Morimoto et al. Citation2001; Surendrakumar et al. Citation2003). Morimoto et al. (Citation2001) found that the inclusion of HA at a low concentration (0.1% and 0.2% w/v) to an insulin solution reasulted in an enhanced absorption, after intratracheal administration to rats. Such enhancement appeared to be independent of the pH of the solution.
In another study, insulin was spray-dried alone or in the presence of HA, HA/zinc ions, or HA/hydroxypropyl cellulose. Subsequently, the release profiles of the resultant insulin from particles were evaluated by monitoring the glucose levels of male Beagle dogs after inhalation (Surendrakumar et al. Citation2003). The administration of spray-dried insulin in the presence of HA to conscious dogs produced a sustained release and increased absorption of the hormone compared with spray-dried insulin alone. In addition, with the inclusion of Zn2 + or hydroxypropyl cellulose to HA-containing particles, the absorption and the mean residence time of insulin were found to increase to 2.5–5- and 7–9-fold, respectively, relative to that of pure insulin powders administered via the pulmonary route.
Parenteral Drug Delivery
The potential applicability of nonimmunogenic HA as drug carriers for parenteral delivery was recognized by Drobnik (Citation1991). Drug targeting to a specific site, such as the lymphatic system might be possible by conjugating a drug with HA or entrapping the drug in the HA matrix. Alternatively, such methods might be used to achieve sustained release or prolong the retention of drug within the body. For example, taxol, an anticancer drug, has been chemically linked to HA. The taxol-HA conjugate was found to selectively target human breast, colon, and ovarian cancer cell lines in vitro (Luo and Prestwich Citation1999; Luo et al. Citation2000). The linkage between HA and taxol was carried out by conjugating the taxol 2′-OH via a succinate ester to adipic dihydrazide-modified HA. After receptor-mediated cellular uptake, the free drug was released from the conjugate as a result of hydrolysis to achieve a concentration that resulted in cytotoxicity in cancer cell lines (Luo et al. Citation2000).
In addition, Sakurai et al. (Citation1997a) found that conjugation of manganese and superoxide dismutase (Sakurai et al. Citation1997b) to HA showed nonimmunogenicity and enhanced the antiinflammatory activity of the two proteins in mice. In another study, a sustained release of human recombinant insulin-like growth factor-I, from a HA matrix, was achieved (Prisell et al. Citation1992). In vitro data indicated that the diffusion rate of the peptide was markedly decreased as a function of HA concentration, possibly due to peptide-HA interactions and were confirmed in vivo.
Liposomal Drug Delivery
Liposomal drug delivery is reported to increase drug solubility, prolong the half-lives of entrapped drugs in the body, and provide protection of the drug within the lipid particle. When HA was linked to lipids, the resultant liposomes possessed improved function and stability, had target-specific cells, and acted as sustained-release carriers of drugs for therapy (Yerushalmi et al. Citation1994; Yerushalmi and Margalit Citation1998; Peer and Margalit Citation2000; Eliaz and Szoka Citation2001; Peer et al. Citation2003).
Peer and Margalit (Citation2000) have investigated the physicochemical properties of HA-modified liposomes and found that they provided comparable or better efficiency of encapsulation of some model chemicals, relative to unmodified liposomes. In addition, the drug release profiles of either modified or unmodified liposomes appeared to be similar and to follow a single rate constant (Peer and Margalit Citation2000). A further study also suggested that HA modification could confer cryoprotection to freeze-dried unilamellar liposomes, inhibiting the formation of larger multilamellar liposomes, which compromises the encapsulating efficiency of liposomes, upon reconstitution (Peer et al. Citation2003). In contrast, the encapsulation efficiency was found to decrease by 25–60% in the unmodified liposomes. The mechanism by which HA conferred cryoprotection of unilamellar liposomes was attributed to HA being able to replace the hydrogen bonds that stabilize the structures of liposomes during freeze-drying (Peer et al. Citation2003).
HA-modified liposome-encapsulated doxorubicin, an anticancer drug, was found to specifically target-cancer cells that express CD 44 (Eliaz and Szoka Citation2001). In addition, encapsulation of the drug in cell targeting liposomes was shown to increase the biological activity of doxorubicin against B16F10 murine melanoma cell line 4-8-fold relative to that of the free drug in terms of the IC50 values; the actual extent of the increase being dependent upon the duration of treatment (Eliaz and Szoka Citation2001). The targeting ability of HA-modified liposomes also was tested by Yerushalmi et al. (Citation1994) who found that more than 87% of the model drug, epidermal growth factor, could be encapsulated in the HA-modified liposomes. The authors found that the modified liposomes specifically adhered to the monolayers of the A431 cell line, the in vivo designated targets for the therapy of wounds and burns (Yerushalmi et al. Citation1994). A further study showed that such adherence was relatively robust and could be retained during cell migration, proliferation, and death, and under fluid flow (Yerushalmi and Margalit Citation1998).
Implantable Drug Delivery
HA has been utilized to develop an implantable delivery device with a view to conferring long-term delivery of protein drugs (Surini et al. Citation2003a, Citation2003b). HA has been reported to interact with chitosan and form a chitosan-HA complex, which in turn affects the drug release rate mainly due to the swelling effect that follows water penetration (Takayama et al. Citation1990). Surini et al. (Citation2003b) produced a chitosan and HA implant containing insulin that enabled programmed release profiles to be achieved. The release of insulin from the system appeared to be zero order in nature, and the release rate was dictated by the quantity of formulation applied and the formulation composition, i.e., the chitosan-to-HA-mixing ratios and the amount of insulin loaded. For example, the release rate constant from a 50-mg pellet containing a chitosan-to-HA-mass ratio of 3:7 was found to be three times faster compared with that from a 135-mg pellet with a chitosan-to-HA-mass ratio of 16:84.
Gene Delivery
Recently, HA has been used to prepare DNA-HA matrices and microspheres with a view to achieving sustained gene delivery (Kim et al. Citation2003; Yun et al. Citation2004). In the matrices and microspheres, DNA entrapped in HA networks was cross-linked with adipic dihydrazide during preparation. These researchers found that the release kinetics of plasmid DNA from the matrices and microspheres could be controlled by optimizing the amount of DNA loaded into the matrices and the process for cross-linking. The release of DNA was shown to be initially dominated by the burst effect, followed by the erosion of HA matrix that could be designed to occur over the longer term (over 2 months). The DNA entrapped in the HA matrix was stabilized against enzymatic degredation but once released was found to be capable of transfection in vitro. In addition, Yun et al. (Citation2004) found that DNA presented in HA microspheres transfected skeletal muscles in vivo. Moreover, after conjugation with monoclonal antibodies, it was found that DNA targetted specific cellular receptors, and the conjugates would distinguish between E- and P-selectin expressing cells. A summary of the drug delivery applications of HA is shown in .
TABLE 2 Summary of the drug delivery applications of HA
CONCLUSIONS
Since its first discovery in 1934, HA's unique viscoelasticity, hygroscopicity, biocompatibility, and nonimmunogenicity properties of hyaluronan have facilitated its use in a variety of medicinal and pharmaceutical applications. As more and more is understood about the macromolecule and how its physicochemical properties can be manipulated by chemical modification or simply controlling its molecular weight, these applications may become more diverse. However, such developments need to be accompanied by an increased knowledge of its cellular functions and how it can be produced, assayed, and controlled in a more cost-effective manner.
REFERENCES
- European Pharmacopoeia. European Pharmacopoeia Commission, Strasbourg 2002
- Abeydeera L. R. In vitro production of embryos in swine. Theriogenology 2002; 57: 257–273, [CSA]
- Adam N., Ghosh P. Hyaluronan molecular weight and polydispersity in some commercial intra-articular injectable preparation and in synovial fluid. Inflamm. Res. 2001; 50: 294–299, [PUBMED], [INFOTRIEVE], [CSA]
- Adams M. E., Lussier A. J., Peyron J. G. A risk-benefit assessment of injections of hyaluronan and its derivatives in the treatment of osteoarthritis of the knee. Drug Saf. 2000; 23: 115–130, [PUBMED], [INFOTRIEVE], [CSA]
- Altman R. D. Intra-articular sodium hyaluronate in osteoarthritis of the knee. Semin. Arthritis Rheum. 2000; 30: 11–18, [PUBMED], [INFOTRIEVE], [CSA], [CROSSREF]
- Amr S. K. The effect of homogenised skin on the activity of licosamde antibiotics. Proc. Millen. Cong. Pharma. Sci. 2000; A80, [CSA]
- Armstrong S. E., Bell D. R. Measurement of high-molecular-weight hyaluronan in solid tissue using agarose gel electrophoresis. Anal. Biochem. 2002; 308: 255–264, [PUBMED], [INFOTRIEVE], [CSA], [CROSSREF]
- Arnott S., Mitra A. K., Raghunathan S. Hyaluronic-acid double helix. J. Mol. Biol. 1983; 169: 861–872, [PUBMED], [INFOTRIEVE], [CSA]
- Asteriou T., Deschrevel B., Delpech B., Bertrand P., Bultelle F., Merai C., Vincent J. C. An improved assay for the n-acetyl—glucosamine reducing ends of polysaccharides in the presence of proteins. Anal. Biochem. 2001; 293: 53–59, [PUBMED], [INFOTRIEVE], [CSA], [CROSSREF]
- Balazs E. A., Cowman M. K., Briller S. O. On limiting viscosity number of hyaluronate in potassium phosphate buffers between pH 6.5 and 8. Biopolymers 1983; 22: 589, [CSA], [CROSSREF]
- Balazs E. A. The viscoelastic intracellular matrix and control of cel function by hyaluronanl. The chemistry, Biology and Medical Applications of Hyaluronan and Its Derivatives, T. C. Laurent. Portland Press, London 1998; 185–204
- Balazs E. A. Analgesic effect of elastoviscous hyaluronan solutions and the treatment of arthritic pain. Cells Tissues Organs 2003; 174: 49–62, [PUBMED], [INFOTRIEVE], [CSA], [CROSSREF]
- Balazs E. A., Denlinger J. L. Clinical uses of hyaluronan. Ciba Foundation Symposia 1989; 143: 265–280, [PUBMED], [INFOTRIEVE], [CSA]
- Balazs E. A., Denlinger J. L. Viscosupplementation: a new concept in the treatment of osteoarthritis. J. Rheumatol. 1993; 20: 3–9, [CSA]
- Band P. A. Hyaluronan derivatives: chemistry and clinical applications. The Chemistry, Biology and Medical Applications of Hyaluronan and its derivatives, T. C. Laurent. Portland Press, London 1998; 33–42
- Banerji S., Ni J., Wang S. X., Clasper S., Su J., Tammi R., Jones M., Jackson D. G. LYVE-1, a new homologue of the CD44 glycoprotein, is a lymph-specific receptor for hyaluronan. J. Cell Biol. 1999; 144: 789–801, [PUBMED], [INFOTRIEVE], [CSA], [CROSSREF]
- Banks J., Kreider J. W., Bhavanadan V. P., Davidson E. A. Anionic polysaccharide production and tyrosine activation in clutured human melanima cells. Cancer Res. 1976; 36: 424–431, [PUBMED], [INFOTRIEVE], [CSA]
- Barrett J. P., Siviero P. Retrospective study of outcomes in Hyalgan (R)-treated patients with osteoarthritis of the knee. Clin. Drug Invest. 2002; 22: 87–97, [CSA]
- Basedow A. M., Ebert K. Ultrasonic degradation of polymers in solution. Adv. Polymer Sci. 1977; 22: 83–148, [CSA]
- Beaty N. B. Relative molecular weight and concentration determination of sodium hyaluronate solutions by gel-exclusion high-performance liquid chromatography. Anal. Biochem. 1985; 147: 387–395, [PUBMED], [INFOTRIEVE], [CSA], [CROSSREF]
- Bernatchez S. F., Tabatabay C., Gurny R. Sodium hyaluronate 0.25-percent used as a vehicle increases the bioavailability of topically administered gentamicin. Graefes Arch. Clin. Exp. Ophthalmol. 1993; 231: 157–161, [PUBMED], [INFOTRIEVE], [CSA], [CROSSREF]
- Bitter T., Muir H. M. A modified uronic acid reaction. Anal. Biochem. 1962; 4: 330–334, [PUBMED], [INFOTRIEVE], [CSA], [CROSSREF]
- Blumberg B. S. Light-scattering studies on hyaluronic acid. Science 1954; 120: 432–433, [PUBMED], [INFOTRIEVE], [CSA]
- Braun D. P., Taylor S. G., Hams J. E. Modulation of immunity in cancer patients by prostaglandin antagonists. Immunity to Cancer II, R. Alan. Liss Inc, New York 1989; 439–448
- Brown M. B., Forbes B., Martin G. P. The use of hyaluronan in topical drug delivery. Hyaluronan: Biomedical, Medical and Clinical Aspects, J. Kennedy, G. O. Phillips, P. A. Williams, V. Hascall. Woodhead Publishers, Cambridge 2002; 249–256
- Brown M. B., Ingham S., Moore A., Marriott C., Martin G. P. A preliminary study of the effect of hyaluronan in drug delivery. Hyaluronan in Drug Delivery, D. A. Willoughby. Royal Society of Medicine Press, London 1995a; 48–52
- Brown M. B., Marriott C., Martin G. P. A study of the transdermal drug delivery properties of hyaluronan. Hyaluronan in Drug Delivery, D. A. Willoughby. Royal Society of Medicine Press, London 1995b; 53–71
- Brown M. B., Marriott C., Martin G. P. The effect of hyaluronan on the in vitro deposition of diclofenac within the skin. Int. J. Tissue Reactions—Exp. Clin. Aspects 1995c; 17: 133–140, [CSA]
- Brown M. B., Martin G. P. Comparison of the effect of hyaluronan and other polysaccharides on drug skin partitioning. Int. J. Pharm. 2001; 225: 113–121, [PUBMED], [INFOTRIEVE], [CSA], [CROSSREF]
- Brown M. B., Moore A. The effects of a novel formulation of cyclosporin on antibody and cell-mediated immune reactions in the pleural cavity of rats. Hyaluronan in Drug Delivery, D. A. Willoughby. Royal Society of Medicine Press, London 1996; 121–131
- Brown T. J., Alcorn D., Fraser J. R. E. Absorption of hyaluronan applied to the surface of intact skin. J. Invest. Dermatol. 1999; 113: 740–746, [PUBMED], [INFOTRIEVE], [CSA], [CROSSREF]
- Brunda M. J., Herberman R. B., Holden H. I. Inhibition of munne natural killer cell activity by prostaglandins. J. Immunol. 1980; 124: 2682–2687, [PUBMED], [INFOTRIEVE], [CSA]
- Bucolo C., Mangiafico P. Pharmacological profile of a new topical pilocarpine formulation. J. Ocul. Pharmacol. Ther. 1999; 15: 567–573, [PUBMED], [INFOTRIEVE], [CSA]
- Bucolo C., Spadaro A., Mangiafico S. Pharmacological evaluation of a new timolol/pilocarpine formulation. Ophthalmic Res. 1998; 30: 101–106, [PUBMED], [INFOTRIEVE], [CSA], [CROSSREF]
- Budiaman E. R., Fennema O. Linear rate of water crystallization as influenced by temperature of hydrocolloid suspensions. J. Dairy Sci. 1987; 70: 534–546, [CSA]
- Camber O., Edman P. Sodium hyaluronate as an ophthalmic vehicle—some factors governing its effect on the ocular absorption of pilocarpine. Curr. Eye Res. 1989; 8: 563–567, [PUBMED], [INFOTRIEVE], [CSA]
- Camber O., Edman P., Gurny R. Influence of sodium hyaluronate on the meiotic effect of pilocarpine in rabbits. Curr. Eye Res. 1987; 6: 779–784, [PUBMED], [INFOTRIEVE], [CSA]
- Campoccia D., Doherty P., Radice M., Brun P., Abatangelo G., Williams D. F. Semi-synthetic restorable materials from hyaluronan esterification. Biomaterials 1998; 19: 2101–2127, [PUBMED], [INFOTRIEVE], [CSA], [CROSSREF]
- Cantor J. O., Cerreta J. M., Armand G., Turino G. M. Aerosolized hyaluronic acid decreases alveolar injury induced by human neutrophil elastase. Proc. Soc. Exp. Biol. Med. 1998; 217: 471–475, [PUBMED], [INFOTRIEVE], [CSA]
- Castellano F., Mautone G. Decongestant activity of a new formulation of xylometazoline nasal spray: a double-blind, randomized versus placebo and reference drugs controlled, dose-effect study. Drugs Exp. Clin. Res. 2002; 28: 27–35, [PUBMED], [INFOTRIEVE], [CSA]
- Chaidedgumjorn A., Suzuki A., Toyoda H., Toida T., Imanari T., Linhardt R. J. Conductivity detection for molecular mass estimation of per-O-sulfonated glycosaminoglycans separated by high-performance size-exclusion chromatography. J. Chromatogr. A 2002; 959: 95–102, [PUBMED], [INFOTRIEVE], [CSA], [CROSSREF]
- Cichy J., Pure E. The liberation of CD44. J. Cell Biol. 2003; 161: 839–843, [PUBMED], [INFOTRIEVE], [CSA], [CROSSREF]
- Cleland R. L., Wang J. L. Ionic polysaccharides. III. Dilute solution properties of hyaluronic acid fractions. Biopolymers 1970; 9: 799, [PUBMED], [INFOTRIEVE], [CSA], [CROSSREF]
- Cowman M. K., Liu J., Hittner D. M., Kim J. S. Hyaluronan interactions: salt, water, ions'. The Chemistry, Biology and Medical Applications of Hyaluronan and Its Derivatives, T. C. Laurent. Portland Press, London 1998; 17–25
- Day A. J., Prestwich G. D. Hyaluronan-binding proteins: tying up the giant. J. Biol. Chem. 2002; 277: 4585–4588, [PUBMED], [INFOTRIEVE], [CSA], [CROSSREF]
- Day A. J., Sheehan J. K. Hyaluronan: polysaccharide chaos to protein organisation. Curr. Opin. Struct. Biol. 2001; 11: 617–622, [PUBMED], [INFOTRIEVE], [CSA], [CROSSREF]
- Del Rosso J. Q. New and emerging topical approaches for actinic keratoses. Cutis 2003; 72: 273, [PUBMED], [INFOTRIEVE], [CSA]
- Denlinger J. L. Hyaluronan and its derivatives as viscoelastics in medicine. The Chemistry, Biology and Medical Applications of Hyaluronan and Its Derivatives, T. C. Laurent. Portland Press, London 1998; 235–242
- Doherty M. M., Hughes P. J., Kim S. R., Mainwaring D. E., Charman W. N. Effect of lyophilization on the physical characteristics of medium molecular-mass hyaluronates. Int. J. Pharm. 1994; 111: 205–211, [CSA], [CROSSREF]
- Dougados M. Sodium hyaluronate therapy in osteoarthritis: arguments for a potential beneficial structural effect. Semin. Arth. Rheum. 2000; 30: 19–25, [CSA], [CROSSREF]
- Drobnik J. Hyaluronan in drug delivery. Adv. Drug Dev. Rev. 1991; 7: 295–308, [CSA], [CROSSREF]
- Durrani A. M., Farr S. J., Kellaway I. W. Influence of molecular-weight and formulation Ph on the precorneal clearance rate of hyaluronic-acid in the rabbit eye. Int. J. Pharm. 1995; 118: 243–250, [CSA], [CROSSREF]
- Eliaz R. E., Szoka F. C. Liposome-encapsulated doxorubicin targeted to CD44: a strategy to kill CD44-overexpressing tumor cells. Cancer Res. 2001; 61: 2592–2601, [PUBMED], [INFOTRIEVE], [CSA]
- Elliott G. R., Tak C., Pellens C., Ben-Efraim S., Bosta I. L. Indomethacin stimulation of macrophage cytostasis against MOPC 315 tumor cells is inhibited by both prostaglandin E2 and norhydroguaiaretic acid, a lipooxygenase inhibitor. Cancer Immun. Immunother. 1988; 27: 133–136, [CSA]
- Falk R. E., Mikkelsaar S., Asculai S., Bryant C., Veenstra J. Evaluation of prostaglandin (PGE) inhibitors combined with cytodestructive therapy in advanced neoplasia. Proc. Int. Clin. Hypertherm. Meeting. Loyon, Frrance, 1992
- Fieldman S. R., Fleischer A. B., McConnell C. Most common dermatological problems identified by internists. Arch. Intern. Med. 1998; 158: 726–730, [CSA], [CROSSREF]
- Figueiredo F., Jones G. M., Thouas G. A., Trounson A. O. The effect of extracellular matrix molecules on mouse preimplantation embryo development in vitro. Repro. Fert. Devel. 2002; 14: 443–451, [CSA], [CROSSREF]
- Fraser J. R. E., Brown T. J., Laurent T. C. Catabolism of hyaluronan. The Chemistry, Biology and Medical Applications of Hyaluronan and its Derivatives, T. C. Laurent. Portland Press, London 1998; 85–92
- Fraser J. R. E., Kimpton W. G., Pierscionek B. K., Cahill R. N. P. The kinetics of hyaluronan in normal and acutely inflamed synovial joints—observations with experimental arthritis in sheep. Semin. Arth. Rheum. 1993; 22: 9–17, [CSA]
- Fraser J. R. E., Laurent T. C., Laurent U. B. G. Hyaluronan: its nature, distribution, functions and turnover. J. Intern. Med. 1997; 242: 27–33, [PUBMED], [INFOTRIEVE], [CSA], [CROSSREF]
- Fujii K., Kawata M., Kobayashi Y., Okamoto A., Nishinari K. Effects of the addition of hyaluronate segments with different chain lengths on the viscoelasticity of hyaluronic acid solutions. Biopolymers 1996; 38: 583–591, [PUBMED], [INFOTRIEVE], [CSA], [CROSSREF]
- Furnus C. C., de Matos D. G., Martinez A. G. Effect of hyaluronic acid on development of in vitro produced bovine embryos. Theriogenology 1998; 49: 1489–1499, [PUBMED], [INFOTRIEVE], [CSA], [CROSSREF]
- Gandolfi S. A., Massari A., Orsoni J. G. Low-molecular-weight sodium hyaluronate in the treatment of bacterial corneal ulcers. Graefes Arch. Clin. Exp. Ophthalmol. 1992; 230: 20–23, [PUBMED], [INFOTRIEVE], [CSA]
- Gardner D. K., Lane M., Stevens J., Schoolcraft W. B. Changing the start temperature and cooling rate in a slow-freezing protocol increases human blastocyst viability. Fertil. Steril. 2003; 79: 407–410, [PUBMED], [INFOTRIEVE], [CSA]
- Gardner D. K., Rodriegez-Martinez H., Lane M. Fetal development after transfer is increased by replacing protein with the glycosaminoglycan hyaluronan for mouse embryo culture and transfer. Hum. Reprod. 1999; 14: 2575–2580, [PUBMED], [INFOTRIEVE], [CSA], [CROSSREF]
- Ghosh P., Guidolin D. Potential mechanism of action of intra-articular hyaluronan therapy in osteoarthritis: Are the effects molecular weight dependent?. Semin. Arth. Rheum. 2002; 32: 10–37, [CSA], [CROSSREF]
- Ghosh P., Hutadilok N., Adam N., Lentini A. Interactions of hyaluronan (hyaluronic-acid) with phospholipids as determined by gel-permeation chromatography, multi-angle laser-light-scattering photometry and h-1-nmr spectroscopy. Int. J. Biol. Macromol. 1994; 16: 237–244, [PUBMED], [INFOTRIEVE], [CSA], [CROSSREF]
- Ghosh S., Jassal M. Use of polysaccharide fibres for modem wound dressings. Ind. J. Fibre Textile Res. 2002; 27: 434–450, [CSA]
- Gibbs D. A., Merrill E. W., Smith K. A., Balazs E. A. Rheology of hyaluronic acid. Biopolymers 1968; 6: 777–791, [PUBMED], [INFOTRIEVE], [CSA], [CROSSREF]
- Goodison S., Urquidi V., Tarin D. CD44 cell adhesion molecules. J. Clin. Path.-Mol. Path. 1999; 52: 189–196, [CSA]
- Gribbon P., Heng B. C., Hardingham T. E. The molecular basis of the solution properties of hyaluronan investigated by confocal fluorescence recovery after photobleaching. Biophys. J. 1999; 77: 2210–2216, [PUBMED], [INFOTRIEVE], [CSA]
- Gribbon P., Heng B. C., Hardingham T. E. The analysis of intermolecular interactions in concentrated hyaluronan solutions suggest no evidence for chain-chain association. Biochem. J. 2000; 350: 329–335, [PUBMED], [INFOTRIEVE], [CSA], [CROSSREF]
- Grimshaw J., Kane A., Trocha-Grimshaw J., Douglas A., Chakravrthy U., Archer D. Electrophoresis 1994; 15: 936–940, [PUBMED], [INFOTRIEVE], [CSA], [CROSSREF]
- Guidolin D. D., Ronchetti I. P., Lini E., Guerra D., Frizziero L. Morphological analysis of articular cartilage biopsies from a randomized. Clinical study comparing the effects of 500-730 kDa sodium hyaluronate (Hyalgan (R)) and methylprednisolone acetate on primary osteoarthritis of the knee. Osteoarthr. Cartilage 2001; 9: 371–381, [CSA], [CROSSREF]
- Gura E., Huckel M., Muller P. J. Specific degradation of hyaluronic acid and its rheological properties. Polym. Degrad. Stab. 1998; 59: 297–302, [CSA], [CROSSREF]
- Gurny R., Ibrahim H., Aebi A., Buri P., Wilson C. G., Washington N., Edman P., Camber O. Design and evaluation of controlled release systems for the eye. J. Control. Rel. 1987; 6: 367–373, [CSA], [CROSSREF]
- Hamburger M. I., Lakhanpal S., Mooar P. A., Oster D. Intra-articular hyaluronans: a review of product-specific safety profiles. Semin. Arth. Rheum. 2003; 32: 296–309, [CSA], [CROSSREF]
- Hawkins C. L., Davies M. J. Direct detection and identification of radicals generated during the hydroxyl radical-induced degradation of hyaluronic acid and related materials. Free Radic. Biol. Med. 1996; 21: 275–290, [PUBMED], [INFOTRIEVE], [CSA], [CROSSREF]
- Herrero-Vanrell R., Fernandez-Carballido A., Frutos G., Cadorniga R. Enhancement of the mydriatic response to tropicamide by bioadhesive polymers. J. Ocul. Pharmacol. Ther. 2000; 16: 419–428, [PUBMED], [INFOTRIEVE], [CSA]
- Hochberg M. C. Role of intra-articular hyaluronic acid preparations in medical management of osteoarthritis of the knee. Semin. Arth. Rheum. 2000; 30: 2–10, [CSA], [CROSSREF]
- How M. J., Long V. J. W. Separation and fractionation of hyaluronic acid from humam synovial fluid on agrose gels. Clin. Chim. Acta 1969; 23: 251–256, [PUBMED], [INFOTRIEVE], [CSA], [CROSSREF]
- Inoue M., Katakami C. The effect of hyaluronic-acid on corneal epithelial-cell proliferation. Invest. Ophthal. Visual Sci. 1993; 34: 2313–2315, [CSA]
- Iqbal Z. Determination of molecular weight of hyaluronic acid in bovine vitreous humour and Healon by high performance gel permeation chromatography and its depolymerization with ascorbic acid. Pharm. World Sci. 1997; 19: 246–250, [PUBMED], [INFOTRIEVE], [CSA], [CROSSREF]
- Itano N., Kimata K. Mammalian hyaluronan synthases. Iubmb Life 2002; 54: 195–199, [PUBMED], [INFOTRIEVE], [CSA]
- Jaakma U., Zhang B. R., Larsson B., Niwa K., RodriguezMartinez H. Effects of sperm treatments on the in vitro development of bovine oocytes in semidefined and defined media. Theriogenology 1997; 48: 711–720, [CSA], [CROSSREF]
- Jackson C., Larsen B., McEwen C. Comparison of most probable peak values as measured for polymer distributions by MALDI mass spectrometry and by size exclusion chromatography. Anal. Chem. 1996; 68: 1303–1308, [CSA], [CROSSREF]
- Jang G., Lee B. C., Kang S. K., Hwang W. S. Effect of glycosaminoglycans on the preimplantation development of embryos derived from in vitro fertilization and somatic cell nuclear transfer. Repro. Fert. Devel. 2003; 15: 179–185, [CSA], [CROSSREF]
- Jarvinen K., Jarvinen T., Urtti A. Ocular absorption following topical delivery. Adv. Drug Dev. Rev. 1995; 16: 3–19, [CSA], [CROSSREF]
- Jarvis B., Figgitt D. P. Topical 3% diclofenac in 2.5% hyaluronic acid gel—a review of its use in patients with actinic keratoses. Am. J. Clini. Derm. 2003; 4: 203–213, [CSA]
- Joly T., Nibart M., Thibier M. Hyaluronic-acid as a substitute for proteins in the deep-freezing of embryos from mice and sheep—an in vitro investigation. Theriogenology 1992; 37: 473–480, [CSA], [CROSSREF]
- Juhlin L. Hyaluronan in skin. J. Intern. Med. 1997; 242: 61–66, [PUBMED], [INFOTRIEVE], [CSA], [CROSSREF]
- Kakehi K. Hyaluronic acid: separation and biological implications. J. Chromat. 2003; 797: 347–355, [CSA], [CROSSREF]
- Kano K., Miyano T., Kato S. Effects of glycosaminoglycans on the development of in vitro matured and fertilized porcine oocytes to the blastocyst stage in vitro. Biol. Reprod. 1998; 58: 1226–1232, [PUBMED], [INFOTRIEVE], [CSA]
- Karamanos N. K., Hjerpe A. High-performance capillary electrophoretic analysis of hyaluronan in effusions from human malignant mesothelioma1. J. Chromat. B: Biomed. Sci. Appl. 1997; 697: 277–281, [CSA], [CROSSREF]
- Karim S. The determination of the viscosity-average molecular weight of hyaluronan by capillary viscosity. Hyaluronan in Drug Delivery-4th International Workshop, D. Willoughby. Royal Society of Medicine. Press, London 1996; 97–107
- Karim S., Brown M. B., Ingham S., Martin G. P., Marriott C. Rheological and molecular weight studies of hyaluronan. Eur. J. Pharm. Sci. 1996; 4: S175, [CSA], [CROSSREF]
- Karlsson G., Bergman R. Determination of the distribution of molecular masses of sodium hyaluronan by high-performance anion-exchange chromatography. J. Chromat. A. 2003; 986: 67–72, [CSA], [CROSSREF]
- Kaufmann J., Mohle K., Hofmann H. J., Arnold K. Molecular dynamics study of hyaluronic acid in water. Theochem—J. Mol. Struc. 1998; 422: 109–121, [CSA], [CROSSREF]
- Kaur I. P., Smitha R. Penetration enhancers and ocular bioadhesives: Two new avenues for ophthalmic drug delivery. Drug Dev. Ind. Pharm. 2002; 28: 353–369, [PUBMED], [INFOTRIEVE], [CSA], [CROSSREF]
- Kelly M. A., Goldberg V. M., Healy W. L., Pagnano M. W., Hamburger M. I. Osteoarthritis and beyond: a consensus on the past, present, and future of hyaluronans in orthopedics. Orthopedics 2003; 26: 1064–1079, [PUBMED], [INFOTRIEVE], [CSA]
- Kemmann E. Creutzfeldt-Jakob disease (CJD) and assisted reproductive technology (ART)—quantification of risks as part of informed consent. Hum. Reprod. 1998; 13: 1777, [PUBMED], [INFOTRIEVE], [CSA], [CROSSREF]
- Kim A., Checkla D. M., Dehazya P., Chen W. L. Characterization of DNA-hyaluronan matrix for sustained gene transfer. J. Control. Rel. 2003; 90: 81–95, [CSA], [CROSSREF]
- Kirwan J. Is there a place for intra-articular hyaluronate in osteoarthritis of the knee?. Knee 2001; 8: 93–101, [PUBMED], [INFOTRIEVE], [CSA], [CROSSREF]
- Knudson W., Chow G., Knudson C. B. CD44-mediated uptake and degradation of hyaluronan. Matrix Biol. 2002; 21: 15–23, [PUBMED], [INFOTRIEVE], [CSA], [CROSSREF]
- Kobayashi Y., Okamoto A., Nishinari K. Viscoelasticity of hyaluronic-acid with different molecular weights. Biorheology 1994; 31: 235–244, [PUBMED], [INFOTRIEVE], [CSA]
- Koshiishi I., Horikoshi E., Imanari T. Quantification of hyaluronan and chondroitin/dermatan sulfates in the tissue sections on glass slides. Anal. Biochem. 1999; 267: 222–226, [PUBMED], [INFOTRIEVE], [CSA], [CROSSREF]
- Lane M., Maybach J. M., Hooper K., Hasler J. F., Gardner D. K. Cryo-survival and development of bovine blastocysts are enhanced by culture with recombinant albumin and hyaluronan. Mol. Reprod. Dev. 2003; 64: 70–78, [PUBMED], [INFOTRIEVE], [CSA], [CROSSREF]
- Langer K., Mutschler E., Lambrecht G., Mayer D., Troschau G., Stieneker F., Kreuter J. Methylmethacrylate sulfopropylmethacrylate copolymer nanoparticles for drug delivery—Part III: evaluation as drug delivery system for ophthalmic applications. Int. J. Pharm. 1997; 158: 219–231, [CSA], [CROSSREF]
- Lapcik L., Lapcik L., De Smedt S., Demester J., Chabrecek P. Hyaluronan: preparation, structure, properties, and applications. Chem. Rev. 1998; 98: 2663–2684, [PUBMED], [CSA], [CROSSREF]
- Larsen N. E., Balazs E. A. Drug delivery systems using hyaluronan and its derivatives. Adv. Drug Dev. Rev. 1991; 7: 279–293, [CSA], [CROSSREF]
- Laurent T. C., Gergely J. Light scattering studies on hyaluronic acid. J. Biol. Chem. 1955; 212: 36–40, [CSA]
- Laurent T. C. Fraction of hyaluronic acid: the polydispersity of hyaluronic acid from the bovine vitreous body. Biochim. Biophys. Acta 1960; 42: 476–485, [PUBMED], [INFOTRIEVE], [CSA], [CROSSREF]
- Laurent T. C. Structure of hyaluronic acid. Chemistry and the Molecular Biology of the Intracellular Matrix, E. A. Balazs. Academic Press, London 1970; 703–732
- Laurent T. C. An early look at macromolecular crowding. Biophys. Chem. 1995; 57: 7–14, [CSA], [CROSSREF]
- Laurent T. C., Fraser J. R. E. Hyaluronan. FASEB J. 1992; 6: 2397–2404, [PUBMED], [INFOTRIEVE], [CSA]
- Lebel L. Clearance of hyaluronan from the circulation. Adv. Drug Dev. Rev. 1991; 7: 221–235, [CSA]
- Lee H. G., Cowman M. K. An agarose gel electrophoretic method for analysis of hyaluronan molecular weight distribution. Ana. Biochem. 1994; 219: 278–287, [CSA]
- Lee J. Y., Spicer A. P. Hyaluronan: a multifunctional, megaDalton, stealth molecule. Curr. Opin. Cell Biol. 2000; 12: 581–586, [PUBMED], [INFOTRIEVE], [CSA], [CROSSREF]
- Lim S. T., Forbes B., Berry D. J., Martin G. P., Brown M. B. In vivo evaluation of novel hyaluronan/chitosan microparticulate delivery systems for the nasal delivery of gentamicin in rabbits. Int. J. Pharm. 2002; 231: 73–82, [PUBMED], [INFOTRIEVE], [CSA], [CROSSREF]
- Lim S. T., Martin G. P., Berry D. J., Brown M. B. Preparation and evaluation of the in vitro drug release properties and mucoadhesion of novel microspheres of hyaluronic acid and chitosan. J. Control. Rel. 2000; 66: 281–292, [CSA], [CROSSREF]
- Lin W., Maibach H. I. Percutaneous absorption of diclofenac in hyaluronic acid gel: in vitro study in human skin. Hyaluronan in Drug Delivery, D. Willoughby. R. S. M. Press, London 1996; 167–174
- Liu J., Cowman M. K. Thermal analysis of semi-dilute hyaluronan solutions. J. Thermal Anal. Calorimetry 2000; 59: 547–557, [CSA], [CROSSREF]
- Lowry K. M., Beavers E. M. Thermal-stability of sodium hyaluronate in aqueous-solution. J. Biomed. Mater. Res. 1994; 28: 1239–1244, [PUBMED], [INFOTRIEVE], [CSA], [CROSSREF]
- Luo Y., Prestwich G. D. Synthesis and selective cytotoxicity of a hyaluronic acid-antitumor bioconjugate. Bioconjug. Chem. 1999; 10: 755–763, [PUBMED], [INFOTRIEVE], [CSA], [CROSSREF]
- Luo Y., Ziebell M. R., Prestwich G. D. A hyaluronic acid-taxol antitumor bioconjugate targeted to cancer cells. Biomacromolecules 2000; 1: 208–218, [PUBMED], [INFOTRIEVE], [CSA], [CROSSREF]
- Mabuchi K., Obara T., Ikegami K., Yamaguchi T., Kanayama T. Molecular weight independence of the effect of additive hyaluronic acid on the lubricating characteristics in synovial joints with experimental deterioration. Clin. Biomech. 1999; 14: 352–356, [CSA], [CROSSREF]
- Maheu E., Ayral X., Dougados M. A hyaluronan preparation (500–730 kDa) in the treatment of osteoarthritis: a review of clinical trials with Hyalgan((R)). Int. J. Clin. Pract. 2002; 56: 804–813, [PUBMED], [INFOTRIEVE], [CSA]
- McEwan L. E., Smith J. G. Topical diclofenac/hyaluronan gel in the treatment of solar keratoses. Aust. J. Derm. 1997; 38: 187–189, [CSA]
- Mendichi R., Schieroni A. G., Grassi C., Re A. Characterisation of ultra-high molar mass hyaluronan: 1. Off-line static methods. Polymer 1998; 39: 6611–6620, [CSA], [CROSSREF]
- Menzel E. J., Farr C. Hyaluronidase and its substrate hyaluronan: biochemistry, biological activities and therapeutic uses. Cancer Lett. 1998; 131: 3–11, [PUBMED], [INFOTRIEVE], [CSA], [CROSSREF]
- Meyer L., Palmer J. The polysaccharide of the vitreous humour. Biol. Chem. 1934; 107: 629–634, [CSA]
- Milas M., Rinaudo M., Roure I., Al Assaf S., Phillips G. O., Williams P. A. Comparative rheological behavior of hyaluronan from bacterial and animal sources with cross-linked hyaluronan (hylan) in aqueous solution. Biopolymers 2001; 59: 191–204, [PUBMED], [INFOTRIEVE], [CSA], [CROSSREF]
- Miltner O., Schneider U., Siebert C. H., Niedhart C., Niethard F. U. Efficacy of intraarticular hyaluronic acid in patients with osteoarthritis—a prospective clinical trial. Osteoarth. Cartilage 2002; 10: 680–686, [CSA], [CROSSREF]
- Miyano T., Hirooka R. E., Kano K., Miyake M., Kusunoki H., Kato S. Effects of hyaluronic-acid on the development of 1-cell and 2-cell porcine embryos to the blastocyst stage in vitro. Theriogenology 1994; 41: 1299–1305, [CSA], [CROSSREF]
- Miyazaki T., Miyauchi S., Nakamura T., Takeshita S., Horie K. The effect of sodium hyaluronate on the growth of rabbit cornea epithelial cells in vitro. J. Ocul. Pharmacol. Ther. 1996; 12: 409–415, [PUBMED], [INFOTRIEVE], [CSA]
- Miyazaki T., Yomota C., Okada S. Change in molecular weight of hyaluronic acid during measurement with a cone-plate rotational viscometer. J. Appl. Polym. Sci. 1998; 67: 2199–2206, [CSA], [CROSSREF]
- Miyazaki T., Yomota C., Okada S. Hyaluronate depolymerization following thermal decomposition of oxytetracycline. Chem. Pharma. Bull. 2001; 49: 118–122, [CSA], [CROSSREF]
- Mo Y., Takaya T., Nishinari K., Kubota K., Okamoto A. Effects of sodium chloride, guanidine hydrochloride, and sucrose on the viscoelastic properties of sodium hyaluronate solutions. Biopolymers 1999; 50: 23–34, [CSA], [CROSSREF]
- Moreira C. A., Armstrong D. K., Jelliffe R. W., Moreira A. T., Woodford C. C., Liggett P. E., Trousdale M. D. Sodium hyaluronate as a carrier for intravitreal gentamicin—an experimental-study. Acta Ophthalmol. (Copenh). 1991a; 69: 45–49, [CSA]
- Moreira C. A., Moreira A. T., Armstrong D. K., Jelliffe R. W., Woodford C. C., Liggett P. E., Trousdale M. D. In vitro and in vivo studies with sodium hyaluronate as a carrier for intraocular gentamicin. Acta Ophthalmol. (Copenh). 1991b; 69: 50–56, [CSA]
- Moreland L. W. Intra-articular hyaluronan (hyaluronic acid) and hylans for the treatment of osteoarthritis: mechanisms of action. Arth. Res. Ther. 2003; 5: 54–67, [CSA], [CROSSREF]
- Morimoto K., Metsugi K., Katsumata H., Iwanaga K., Kakemi M. Effects of low-viscosity sodium hyaluronate preparation on the pulmonary absorption of rh-insulin in rats. Drug Dev. Ind. Pharm. 2001; 27: 365–371, [PUBMED], [INFOTRIEVE], [CSA], [CROSSREF]
- Morimoto K., Yamaguchi H., Iwakura Y., Morisaka K., Ohashi Y., Nakai Y. Effects of viscous hyaluronate-sodium solutions on the nasal absorption of vasopressin and an analog. Pharm. Res. 1991; 8: 471–474, [PUBMED], [INFOTRIEVE], [CSA], [CROSSREF]
- Moy R. L. Clinical presentation of actinic keratoses and squamous cell carcinoma. J. Am. Acad. Dermatol. 2000; 42: S8–S10, [CSA], [CROSSREF]
- Nazir T., Martin G. P., Brown M. B. Dermal delivery of cyclosporin A entrapped liposomal gels—preformulation Franz cell diffusion studies using silastic membranes. Pharm. Sci. 2001; 3: 1429, suppl.[CSA]
- Ng C. K., Handley C. J., Preston B. N., Robinson H. C. The extracellular processing and catabolism of hyaluronan in cultured adult articular-cartilage explants. Arch. Biochem. Biophys. 1992; 298: 70–79, [PUBMED], [INFOTRIEVE], [CSA], [CROSSREF]
- Ogston A. G., Stainer J. E. On the state of hyaluronic acid in synovial fluid. Biochem. J. 1950; 46: 364–376, [CSA]
- Ogston A. G., Stainer J. E. The dimension of the particle of hyaluronic acid complex in synovial fluid. Biochem. J. 1951; 49: 585–590, [PUBMED], [INFOTRIEVE], [CSA]
- Ogston A. G., Stainer J. E. Further observation on the preparation and compasition of the hyaluronic acid complex of ox synovial fluid. Biochem. J. 1952; 52: 149–156, [PUBMED], [INFOTRIEVE], [CSA]
- Ortonne J. P. A controlled study of the activity of hyaluronic acid in the treatment of venous leg ulcers. J. Dermat. Treat. 1996; 7: 75–81, [CSA]
- Panie W. R. Regression of head and neck carcinoma with a prostaglandin-synthesis inhibitor. Arch. Otolaryngol. 1981; 107: 658–663, [CSA]
- Pasquali Ronchetti I., Quaglino D., Mori G., Bacchelli B., Ghosh P. Hyaluronan-phospholipid interactions. J. Struct. Biol. 1997; 120: 1–10, [PUBMED], [INFOTRIEVE], [CSA], [CROSSREF]
- Peer D., Florentin A., Margalit R. Hyaluronan is a key component in cryoprotection and formulation of targeted unilamellar liposomes. Biochimi. Biophys. Acta-Biomemb. 2003; 1612: 76–82, [CSA], [CROSSREF]
- Peer D., Margalit R. Physicochemical evaluation of a stability-driven approach to drug entrapment in regular and in surface-modified liposomes. Arch. Biochem. Biophys. 2000; 383: 185–190, [PUBMED], [INFOTRIEVE], [CSA], [CROSSREF]
- Pigman W., Rizvi S., Holley H. Preparation and stability of hyaluronic acid. Biochim. Biophys. Acta-Biomemb. 1961; 46: 100, [CSA], [CROSSREF]
- Platzer M., Ozegowski J. H., Neubert R. H. H. Quantification of hyaluronan in pharmaceutical formulations using high performance capillary electrophoresis and the modified uronic acid carbazole reaction. J. Pharm. Biomed. Anal. 1999; 21: 491–496, [PUBMED], [INFOTRIEVE], [CSA], [CROSSREF]
- Poggi M. M., Klein M. B., Chapo G. A., Cuono C. B. Effects of cryopreservation and deconstruction on the dermal glycosaminoglycan content of human skin. J. Burn Care Rehab. 1999; 20: 201–206, [CSA]
- Ponta H., Sherman L., Herrlich P. A. CD44: from adhesion molecules to signalling regulators. Nat. Revi. Mol. Cell Biol. 2003; 4: 33–45, [CSA], [CROSSREF]
- Praest B. M. Assay of synovial fluid parameters: hyaluronan concentration as a potential marker for joint diseases. Clin. Chim. Acta. 1997; 266: 117–128, [PUBMED], [INFOTRIEVE], [CSA], [CROSSREF]
- Prevo R., Banerji S., Ferguson D. J. P., Clasper S., Jackson D. G. Mouse LYVE-1 is an endocytic receptor for hyaluronan in lymphatic endothelium. J. Biol. Chem. 2001; 276: 19420–19430, [PUBMED], [INFOTRIEVE], [CSA], [CROSSREF]
- Prisell P. T., Camber O., Hiselius J., Norstedt G. Evaluation of hyaluronan as a vehicle for peptide growth factors. Int. J. Pharm. 1992; 85: 51–56, [CSA], [CROSSREF]
- Pritchard K., Lansley A. B., Martin G. P., Helliwell M., Marriott C., Benedetti L. M. Evaluation of the bioadhesive properties of hyaluronan derivatives: detachment weight and mucociliary transport rate studies. Int. J. Pharm. 1996; 129: 137–145, [CSA], [CROSSREF]
- Reed C. E. L. The effects of pH on hyaluronate as observed by light scattering. Biopolymers 1989; 28: 1981–2000, [PUBMED], [INFOTRIEVE], [CSA], [CROSSREF]
- Reed C. E., Reed W. F. Light-scattering power of randomly cut random coils with application to the determination of depolymerization rates. J. Chem. Phys. 1989; 91: 7193–7199, [CSA], [CROSSREF]
- Risberg B. Adhesions: preventive strategies. Eur. J. Surg. 1997; 163: 32–39, [CSA]
- Saettone M. F., Chetoni P., Torracca M. T., Burgalassi S., Giannaccini B. Evaluation of muco-adhesive properties and in vivo activity of ophthalmic vehicles based on hyaluronic-acid. Int. J. Pharm. 1989; 51: 203–212, [CSA], [CROSSREF]
- Saettone M. F., Giannaccini B., Chetoni P., Torracca M. T., Monti D. Evaluation of high-molecular-weight and low-molecular-weight fractions of sodium hyaluronate and an ionic complex as adjuvants for topical ophthalmic vehicles containing pilocarpine. Int. J. Pharm. 1991; 72: 131–139, [CSA], [CROSSREF]
- Saettone M. F., Monti D., Torracca M. T., Chetoni P. Mucoadhesive ophthalmic vehicles—evaluation of polymeric low-viscosity formulations. J. Ocul. Pharmacol. 1994; 10: 83–92, [PUBMED], [INFOTRIEVE], [CSA]
- Sakai S., Yasuda R., Sayo T., Ishikawa O., Inoue S. Hyaluronan exists in the normal stratum corneum. J. Invest. Dermatol. 2000; 114: 1184–1187, [PUBMED], [INFOTRIEVE], [CSA]
- Sakurai K., Andoh M., Yamada M., Kodera Y., Nishimura H., Hiroto M., Matsushima A., Aoyama M., Yamamoto H., Inada Y. Suppression of ischemic edema in mice by manganese-hyaluronate conjugate. Jpn. J. Pharmacol. 1997a; 74: 117–120, [PUBMED], [INFOTRIEVE], [CSA]
- Sakurai K., Miyazaki K., Kodera Y., Nishimura H., Shingu M., Inada Y. Anti-inflammatory activity of superoxide dismutase conjugated with sodium hyaluronate. Glycoconj. J. 1997b; 14: 723–728, [PUBMED], [INFOTRIEVE], [CSA]
- Sasaki H., Yamamura K., Nishida K., Nakamura J., Ichikawa M. Delivery of drugs to the eye by topical application. Prog. Retin. Eye Res. 1996; 15: 583–620, [CSA]
- Schartz R. A. The actinic keratoses. A perspective and update. Dermatol. Surg. 1997; 23: 1009–1019, [CSA]
- Scott J. E. The periphery of the developing collagen fibril—quantitative relationships with dermatan sulfate and other surface-associated species. Biochem. J. 1984; 218: 229–233, [PUBMED], [INFOTRIEVE], [CSA]
- Scott J. E., Heatley F. Hyaluronan forms specific stable tertiary structures in aqueous solution: a C-13 NMR study. Proc. Natl. Acad. Sci. USA 1999; 96: 4850–4855, [PUBMED], [INFOTRIEVE], [CSA]
- Scott J. E., Heatley F. Biological properties of hyaluronan in aqueous solution are controlled and sequestered by reversible tertiary structures, defined by NMR spectroscopy. Biomacromolecules 2002; 3: 547–553, [PUBMED], [INFOTRIEVE], [CSA]
- Seno N., Anno K., Kondo S., Nagase S., Saito S. Improved method for electrophoretic separation and rapid quantitation of isomeric chondroitin sulfates on cellulose acetate strips. Anal. Biochem. 1970; 1970: 197–202, [CSA]
- Sheehan J. K., Arundel C., Phelps C. F. Effect of the cations sodium, potassium and calcium on the interaction of hyaluronate chains—a light-scattering and viscometric study. Int. J. Biol. Macromol. 1983; 5: 222–228, [CSA]
- Shimada E., Matsumura G. Viscosity and molecular weight of hyaluronic acids. J. Biochem. 1975; 78: 513–517, [PUBMED], [INFOTRIEVE], [CSA]
- Shinomiya K., Hoshi Y., Imanari T. Sensitive detection of unsaturated disaccharides from chondroitin sulfates by thin-layer chromatography as their dansylhydrazine derivatives. J. Chromatogr. 1989; 462: 471–474, [PUBMED], [INFOTRIEVE], [CSA]
- Simon A., Safran A., Revel A., Aizenman E., Reubinoff B., Porat-Katz A., Lewin A., Laufer N. Hyaluronic acid can successfully replace albumin as the sole macromolecule in a human embryo transfer medium. Fertil. Steril. 2003; 79: 1434–1438, [PUBMED], [INFOTRIEVE], [CSA]
- Soldati D., Rahm F., Pasche P. Mucosal wound healing after nasal surgery. A controlled clinical trial on the efficacy of hyaluronic acid containing cream. Drugs Exp. Clin. Res. 1999; 25: 253–261, [PUBMED], [INFOTRIEVE], [CSA]
- Spicer A. P., Nguyen T. K. Mammalian hyaluronan synthases: investigation of functional relationships in vivo. Biochem. Soc. Trans. 1999; 27: 109–115, [PUBMED], [INFOTRIEVE], [CSA]
- Stiebel-Kalish H., Gaton D. D., Weinberger D., Loya N., Schwartz-Ventik M., Solomon A. A comparison of the effect of hyaluronic acid versus gentamicin on corneal epithelial healing. Eye 1998; 12: 829–833, [PUBMED], [INFOTRIEVE], [CSA]
- Suchanek E., Simunic V., Juretic D., Grizelj V. Follicular-fluid contents of hyaluronic-acid, follicle-stimulating-hormone and steroids relative to the success of in-vitro fertilization of human oocytes. Fertil. Steril. 1994; 62: 347–352, [PUBMED], [INFOTRIEVE], [CSA]
- Sundblad L. Glycosaminoglycans and glycoproteins in synovial fluid. The Amino Sugars. The Chemistry and Biology of the Compounds Containing the Amino Sugars, E. A. Balazs, R. W. Jeanloz. Academic Press, New York 1965; 229–250
- Surendrakumar K., Martyn G. P., Hodgers E. C. M., Jansen M., Blair J. A. Sustained release of insulin from sodium hyaluronate based dry powder formulations after pulmonary delivery to beagle dogs. J. Control. Rel. 2003; 91: 385–394, [CSA]
- Surini S., Akiyama H., Morishita M., Nagai T., Takayama K. Release phenomena of insulin from an implantable device composed of a polyion complex of chitosan and sodium hyaluronate. J. Control. Rel. 2003a; 90: 291–301, [CSA]
- Surini S., Akiyama H., Morishita M., Takayama K., Nagai T. Polyion complex of chitosan and sodium hyaluronate as an implant device for insulin delivery. Stp Pharma Sciences 2003b; 13: 265–268, [CSA]
- Takayama K., Hirata M., Machida Y., Masada T., Sannan T., Nagai T. Effect of interpolymer complex-formation on bioadhesive property and drug release phenomenon of compressed tablet consisting of chitosan and sodium hyaluronate. Chemi. Pharm. Bull. 1990; 38: 1993–1997, [CSA]
- Tammi M. I., Day A. J., Turley E. A. Hyaluronan and homeostasis: a balancing act. J. Biol. Chem. 2002; 277: 4581–4584, [PUBMED], [INFOTRIEVE], [CSA]
- Tammi R., Agren U. M., Tuhkanen A. L., Tammi M. Hyaluronan metabolism in skin—introduction. Hyaluronan Metabol. in Skin 1994; 29: 1–77, [CSA]
- Tammi R., Saamanen A. M., Maibach H. I., Tammi M. Degradation of newly synthesized high molecular mass hyaluronan in the epidermal and dermal compartments of human skin in organ culture. J. Invest. Dermatol. 1991; 97: 126–130, [PUBMED], [INFOTRIEVE], [CSA]
- Tani E., Katakami C., Negi A. Effects of various eye drops on corneal wound healing after superficial keratectomy in rabbits. Jpn. J. Ophthal. 2002; 46: 488–495, [CSA]
- Tascioglu F., Oner C. Efficacy of intra-articular sodium hyaluronate in the treatment of knee osteoarthritis. Clin. Rheumatol. 2003; 22: 112–117, [CSA]
- Tilden A. B., Balch C. M. Immune modulatory effects of indomethacin in melanoma are not related to prostaglandin E2-mediated suppression. Surgery 1982; 92: 528, [PUBMED], [INFOTRIEVE], [CSA]
- Toida T., Shima M., Azumaya S., Maruyama T., Toyoda H., Imanari T., Linhardt R. J. Detection of glycosaminoglycans as a copper(II) complex in high-performance liquid chromatography. J. Chromat. A. 1997; 787: 266–270, [CSA]
- Tokita Y., Ohshima K., Okamoto A. Degradation of hyaluronic acid during freeze drying. Polym. Degrad. Stab. 1997; 55: 159–164, [CSA]
- Tokita Y., Okamoto A. Hydrolytic degradation of hyaluronic-acid. Polym. Degrad. Stab. 1995; 48: 269–273, [CSA]
- Tool B. P. Hyaluronan in morphogenesis. Sem. Cell Devel. Biol. 2001; 12: 79–87, [CSA]
- Toole B. P., Wight T. N., Tammi M. I. Hyaluronan-cell interactions in cancer and vascular disease. J. Biol. Chem. 2002; 277: 4593–4596, [PUBMED], [INFOTRIEVE], [CSA]
- Turino G. M., Cantor J. O. Hyaluronan in respiratory injury and repair. Am. J. Respir. Crit. Care Med. 2003; 167: 1169–1175, [PUBMED], [INFOTRIEVE], [CSA]
- Turley E. A., Noble P. W., Bourguignon L. Y. W. Signaling properties of hyaluronan receptors. J. Biol. Chem. 2002; 277: 4589–4592, [PUBMED], [INFOTRIEVE], [CSA]
- Uthman I., Raynauld J. P., Haraoui B. Intra-articular therapy in osteoarthritis. Postgrad. Med. J. 2003; 79: 449–453, [PUBMED], [INFOTRIEVE], [CSA]
- Vanos H. C., Drogendijk A. C., Fetter W. P. F., Heijtink R. A., Zeilmaker G. H. The influence of contamination of culture-medium with hepatitis-B virus on the outcome of invitro fertilization pregnancies. Am. J. Obstet. Gynecol. 1991; 165: 152–159, [CSA]
- Vazquez J. R., Short B., Findlow A. H., Nixon B. P., Boulton A. J. M., Armstrong D. G. Outcomes of hyaluronan therapy in diabetic foot wounds. Diabetes Res. Clin. Pract. 2003; 59: 123–127, [PUBMED], [INFOTRIEVE], [CSA]
- Vercruysse K. P., Lauwers A. R., Demeester J. M. Absolute and empirical determination of the enzymatic-activity and kinetic investigation of the action of hyaluronidase on hyaluronan using viscosimetry. Biochem. J. 1995; 306: 153–160, [PUBMED], [INFOTRIEVE], [CSA]
- Vercruysse K. P., Prestwich G. D. Hyaluronate derivatives in drug delivery. Crit. Rev. Ther. Drug Carrier Syst. 1998; 15: 513–555, [PUBMED], [INFOTRIEVE], [CSA]
- Volpi N., Maccari F. Detection of submicrogram quantities of glycosaminoglycans on agarose gels by sequential staining with toluidine blue and Stains-All. Electrophoresis. 2002; 23: 4060–4066, [PUBMED], [INFOTRIEVE], [CSA]
- Volpi N. Hyaluronic acid and chondroitin sulfate unsaturated disaccharides analysis by high-performance liquid chromatography and fluorimetric detection with dansylhydrazine. Anal. Biochem. 2000; 277: 19–24, [PUBMED], [INFOTRIEVE], [CSA]
- Wedlock D. J., Phillips G. O., Davies A., Gormally J., Wynjones E. Depolymerization of sodium hyaluronate during freeze-drying. Int. J. Biol. Macromol. 1983; 5: 186–188, [CSA]
- Wolf J. E., Taylor J. R., Tschen E., Kang S. W. Topical 3.0% diclofenac in 2.5% hyaluronan gel in the treatment of actinic keratoses. Int. J. Dermatol. 2001; 40: 709–713, [PUBMED], [INFOTRIEVE], [CSA]
- Wuthrich R. P. The proinflammatory role of hyaluronan-CD44 interactions in renal injury. Nephrol. Dial. Transplant. 1999; 14: 2554–2556, [PUBMED], [INFOTRIEVE], [CSA]
- Yerushalmi N., Arad A., Margalit R. Molecular and cellular studies of hyaluronic acid-modified liposomes as bioadhesive carriers for topical drug-delivery in wound-healing. Arch. Biochem. Biophys. 1994; 313: 267–273, [PUBMED], [INFOTRIEVE], [CSA]
- Yerushalmi N., Margalit R. Hyaluronic acid-modified bioadhesive liposomes as local drug depots: effects of cellular and fluid dynamics on liposome retention at target sites. Arch. Biochem. Biophys. 1998; 349: 21–26, [PUBMED], [INFOTRIEVE], [CSA]
- Yeung B., Marecak D. Molecular weight determination of hyaluronic acid by gel filtration chromatography coupled to matrix-assisted laser desorption ionisation mass spectrometry. J. Chromat. A 1999; 852: 573–581, [CSA]
- Yun Y. H., Goetz D. J., Yellen P., Chen W. Hyaluronan microspheres for sustained gene delivery and site-specific targeting. Biomaterials 2004; 25: 147–157, [PUBMED], [INFOTRIEVE], [CSA]
- Zhou B., Weigel J. A., Fauss L. A., Weigel P. H. Identification of the hyaluronan receptor for endocytosis (HARE). J. Biol. Chem. 2000; 275: 37733–37741, [PUBMED], [INFOTRIEVE], [CSA]
- Zhu Y. X., Granick S. Biolubrication: hyaluronic acid and the influence on its interfacial viscosity of an antiinflammatory drug. Macromolecules 2003; 36: 973–976, [CSA]