Abstract
A novel cationic liposome modified with soybean sterylglucoside (SG) and polyethylene glycol-distearoylphosphatidylethanolamine (PEG-DSPE) as a carrier of antisense oligodeoxynucleotide (ODN) for hepatitis B virus (HBV) therapy was constructed. Characteristics of the cationic liposomes modified with SG and PEG (SG/PEG-CL) and their complexes with 15-mer phosphorothioate ODN (SG/PEG-CL-ODN complex) were investigated by incorporation efficiency, morphology, electrophoresis, zeta potentials, and size analysis. Antisense activity of the liposomes and ODN complexes was determined as hepatitis B surface antigen (HBsAg) and hepatitis B e antigen (HBeAg) in HepG2 2.2.15 cells by ELISA. Their tissue and intrahepatic distribution were evaluated following intravenous injection in mice. The complexes gained high incorporation efficiency and intact vesicular structure with mean size at ∼200 nm. The SG/PEG-CL-ODN complexes enhanced the inhibition of both HBsAg and HBeAg expression in the cultured HepG2 2.2.15 cells relative to free ODN. The uptake of SG/PEG-CL and nonmodified cationic liposomes (CL) was primarily by liver, spleen, and lung. Furthermore, the concentration of SG/PEG-CL was significant higher than that of CL in hepatoctyes at 0.5 hr postinjection. The biodistribution of SG/DSPE-CL-ODN complex compare with free ODN showed that liposomes enhanced the accumulation of ODN in the liver and spleen, while decreasing its blood concentration. SG/PEG-CL–mediated ODN transfer to the liver is an effective gene delivery method for cell-specific targeting, which has a potential for gene therapy of HBV infections. SG and PEG-modified cationic liposomes have proven to be an alternative carrier for hepatocyte-selective drug targeting.
Hepatitis B virus (HBV) is an infectious agent with about 200 million carriers worldwide. Many patients with HBV infection develop chronic hepatitis, cirrhosisi, and hepatocellular carcinoma. Unfortunately, treatment for chronic infection by HBV is far from satisfactory. The most successful therapeutic agent so far available is interferon-alpha, that shows a 40% response rate for patients after completion of therapy (Dusheiko Citation1995). Since several viruses have become successful targets of the antisense oligodeoxynucleotide (ODN) approach, this strategy may be promising in targeting HBV infection. Several studies have already shown that ODNs are capable of suppressing HBV in vitro (Blum et al. Citation1991; Wu and Wu Citation1992) and in vivo (Offensperger et al. Citation1993; Soni et al. Citation1998). ODNs are synthetic DNA molecules that can inhibit gene expression within cells by their capability to bind a complementary mRNA sequence and prevent translation of mRMA, thus providing potentially powerful therapeutic tools against viral diseases and cancer (Wagner Citation1994). However, these large polyanionic macromolecules possess several inherent characteristics that restrict their preclinical and clinical utility for the therapy of chronic diseases. These include degradation and inactivation by nucleases in plasma and cells (Akhtar, Kole, and Juliano Citation1991), poor intracellular delivery (Hope et al. Citation1998), rapid plasma elimination (Crooke et al. Citation1996), as well as obvious toxicities.
The inclusion of cationic lipids into the liposome formulations could improve the association with polyanionic nucleic acids and facilitate binding and uptake by cell for the presence of positive surface charge on the particles (Hope et al. Citation1998). Polyethylene glycol-distearoylphosphatidylethanolamine (PEG-DSPE) is a phospholipid derivative of the hydrophilic polymer polyethylene (Mr = 2,000). Recently it Meyer et al. (Citation1998) found that such derivative of PEG can stabilize the particle of cationic liposome plasmid-DNA complexes in vitro and that sterically stabilized liposomes containing PEG-DSPE have long circulation lifetime. Therefore, incorporation of PEG-DSPE into our cationic liposomes would increase particle stability of liposome-ODN complexes and would enhance more targeting by combination of ligand.
Gene transfer to hepatocytes should be of great therapeutic potential since hepatocytes are responsible for the synthesis of a wide variety of proteins, which play important physiological roles in or outside the hepatocytes. Cationic liposomes have been used for hepatic gene transfer to a variety of cell types or for somatic gene therapy. An attempt has been made to transfer the hepatitis C virus (HCV) genome into rat liver with Lipofectin, and the expression of HCV RNA transcripts and HCV core protein has been confirmed (Takehara et al. Citation1995). Additionally, asialoglycoprotein (AGP)-labeled cationic liposomes have been shown to be highly effective in targeting HepG2 cells through a receptor–mediate gene transfer mechanism, because there are AGP receptors (AGP-R) on hepatocytes and HepG2 cells (Koike et al. Citation1994).
Soybean sterylglucoside (SG) remains in the residue of soybean after the oil has been extracted. A previous study showed that dipalmitoylphosphatidylcholine (DPPC)-liposome containing SG had a high degree of liver association in vivo that might be related to recognition of glucose residues of SG by ASGP-R in hepatocytes. SG in the membrane of cationic liposomes may improve the cell specification of the liposomes and could be helpful for the development of hepatocyte specific ODN-carrier (Shimizu et al. Citation1998).
In the present study, we constructed a novel liposome carrier of a 15-mer ODN for the HBV therapy. To improve the stability and liver targeting of the cationic-ODN complexes, modification of PEG-DSPE and SG was introduced into the liposome. Their tissue and intrahepatic distribution of the novel liposome were evaluated following intravenous injection in mice.
MATERIALS AND METHODS
Human hepatoma cell line HepG2 2.2.15 cells were kindly provided by People's Hospital of Peking University (Beijing, China). KM male mice (18–25 g) were obtained from the Institute of Zoology, Chinese Academy of Sciences (Beijing, China). ODN was synthesized with phosphorothioate backbone chemistry by Beijing AUGCT Biological Co. (Beijing, China) and purified by SDS-PAGE, the 5′-3′ sequence of ODN was 5′ > GAT GAC TGT CTC TTA < 3′, the target site of ODN is the cap site of mRNA transcribed from the SP-II promoter (cap site/SPII). SG was generously supplied by Ryukakusan Co. (Tokyo, Japan). DPPC and PEG-DSPE were purchased from NOF (Tokyo, Japan); 3β -[N-(N′,N′-dimethylaminoethane)-carbamoyl] cholesterol (DC-Chol) was synthesized according to the method described by Gao and Huang (Citation1991). As for radiolabeled liposome, 3H-cholesterol (3H-chol) and 125I-ODN were purchased form China Institute of Atomic Energy (Beijing, China). Collagenase (II) was purchased from Sigma (St. Louis, MO, USA). All other chemicals were of reagent grade.
Preparation of Cationic Liposomes
Multilamellar vesicles were prepared by thin film method. Briefly, the lipids mixture was dissolved in a small volume of chloroform and the organic solvent was slowly removed under reduced pressure on a rotary evaporator for ∼2 hr. The thin film was hydrated by adding 1ml 5% glucose solution (5% Glu) with or without ODN at room temperature by vortex mixture. Then the multilamellar vesicles were sonicated in bath sonicator for 10 min at room temperature and then extruded through 0.2-μ m pore sized polycarbonate filters to generate large unilamellar liposomes. The 3H-labeled liposomes included cationic liposomes without modification (CL: DPPC/DC-Chol, 10:10, molar ratio) and SG/PEG-ESPE modified cationic liposomes (SG/PEG-CL: DPPC/DC-Chol/PEG-DSPE/SG, 10: 10:1.34:1.34, molar ratio) were prepared. The radioactivity of liposomes was 4 μ Ci/200 μ l. As for ODN complex, cationic liposomes-ODN complexes without modification (CL-ODN complexes: DC-Chol/ODN, 1:15, molar ratio) and SG/PEG-DSPE-modified cationic liposomes-ODN complex (SG/PEG-CL-ODN complexes: DC-Chol/ODN, 1:15, molar ratio) were prepared, and the amount of 125I-radiolabel was 6 μCi/200 μ l.
The incorporation efficiency was determined by equilibrium dialysis. Briefly 1 ml of ODN cationic liposomes complexes (ODN at 0.33 μ g) was taken separately in a dialysis tubing (SpectraPor 12,000 to 14,000 mwco) and placed in 10 ml of 5% Glu and incubated at 0°C for 12 hr. The outer phase was taken and the concentration of ODN was detected by ultraviolet spectrophotometer at 260 nm.
Liposomes size and zeta potentials in 5% Glu were determined using a Zetasizer 3000HS (Malvern Instruments, Ltd., UK). The morphology of liposomes and complex also was researched by the Hitachi-500 transmission electron microscope (Hitachi, Japan).
Electrophoresis
First, 450 mg Agarose (Sigma) was dissolved in 150 ml of electrode buffer (pH adjusted to 8.0) and cast as a 2.5-mm thick slab using a Horizon 58 (Bethesda Research Laboratories, Life Technologies) apparatus. Electrophoresis was at 100 V for 10 min. Then, the gel was graphed by an AmpGene UV 3000 gel image system (Yongding Technical Development Co. Ltd).
Samples tested in the electrohporesis analysis included SG/PEG-CL-ODN complexes, SG/PEG-CL-ODN complexes destructed by Triton X-100, and SG/PEG-CL-ODN complexes incubated with 50% (v/v) rat plasma for 2 hr, respectively. Next, 10 μ l containing 0.6 μ g of ODN in each sample were analyzed.
Antisense Activity of Cationic Liposomes-ODN Complexes
HepG2 2.2.15 was culture in DMEM containing 10% FBS at 37°C and 5% CO2. For lipofection, suspension cells were seeded at an initial concentration of 1 × 104 per well for 96-well plates. When adherent cells reached ∼80% confluence, the cells were washed extensively to remove the previously produced HBsAg and HBeAg from the medium. After washing, 100 μ l ODN or SG/PEG-CL-ODN complexes at the ODN concentration of 1.25, 2.5, or 5.0 μ M with 100 μ l DMEM containing 10% serum were added. After washing, 100 μ l SG/PEG-CL-ODN complex along with 100 μ l DMEM containing 10% serum were added. Culture supernatants were collected at 24, 48, and 72hr, separately, and at each time the cells were washed and ODN or SG/PEG-CL-ODN complexes and DMEM were added again. Culture supernatants were collected at 24, 48, and 72 hr, separately, each time the cells were washed and new complexes and DMEM were added. The concentration of HBsAg and HBeAg in the culture supernatants was determined by ELASA, following the manual provided by manufacturers.
Biodistribution of 3H-Labeled Cationic Liposomes
The 3H-chol-labeled CL and SG/PEG-CL were injected (4.2 μ g DC-Chol per 200 μ l) into male mice (18–24 g) via the tail vein at a dose of 4 μ Ci/20 g body weight. At adequate time periods after injection, mice were sacrificed and the blood was collected. The heart, liver, spleen, kidney, and lung were excised and weighed. Next, 100 μ l blood and 50–100 mg tissue samples were taken and decolored by 200 μ l HClO4 and 300 μ l 60% H2O2, finally measured in a Pharmacia-Wallac 1410 liquid scintillation analyzer (Turku, Finland).
Hepatic Cell Distribution of 3H-Labeled Cationic Liposomes
Mice were injected intravenously with 3H-labeled CL and 3H-labeled SG/PEG-CL, respectively, at 4 μ Ci/20 g body weight. At 0.5 and 4 hr after administration, the mice were anesthetized. After perfused by 0.9% NaCl to remove blood through vena cava, the liver was excised and cut into small pieces in ∼1 mm3. Then the liver was incubated with 0.05% (w/v) collagenase for 30 min. The dispersed cells were filtered through cotton mesh sieves, followed by centrifugation at 500 rpm for 3 min; the pellets containing hepatocytes cells were washed three times with isotonic PBS (pH 7.4) by centrifuging at 500 rpm for 3 min. The supernatant containing nonhepatocytes cells was similarly centrifuged and washed three times at 1500 rpm for 5 min. Then, 100 μ l hepatocytes and nonhepatocytes cells were taken, treated, and determined the same way as the organ samples.
Biodistribution of 125I-Labeled Cationic Liposomes-ODN Complexes
The ODN solution and SG/PEG-CL-ODN complexes labeled with trace amounts of 125I-ODN (2.5 μ g ODN in 200 μ l; molar ratio of cationic lipid and ODN was 15:1) were injected into male mice (18–24 g) via the tail vein at 6 μ Ci/20 g body weight. At adequate time periods after injection, mice were sacrificed and 100 μ l blood were collected. The heart, liver, spleen, kidney, and lung were excised and weighed. Then the samples were counted directly by SN-605B γ -counter (Shanghai, China). The total area under the curve (AUC) from time 0 to infinity was estimated from the sum of successive trapezoids between each data point, plus an estimation of the tail area from the last concentration-time point to infinity.
RESULTS
Characteristics of SG/PEG-CL and its ODN Complexes
Characteristics of these liposomes and liposomes-ODN complexes are shown in . The complexes yielded high incorporation efficiency when the charge ratio of anionic ODN/cationic lipid was 1:1 (1:15 in molar ratio). The incorporation efficiency of CL-ODN complexes was approximately 91.1%, whereas the encapsulation efficiency of SG/PEG-CL slightly decreased to 89.5%. CL and SG/PEG-CL consisted of homogeneous populations of vesicles with mean size in ∼131.3 nm and 132.4 nm, respectively. After corporation of liposomes with ODN, the average size of CL-ODN complexes increased sharply to 436.4 nm, while the size of SG/PEG-CL–ODN complex increased slightly to 197.5 nm. Compared with the cationic liposoems composed of only DC-Chol and DPPC (CL), addition of SG and PEG-DSPE (SG/PEG-CL) increased the positive zeta potential of cationic liposomes from 47.0 mV to 59.6 mV. As for ODN cationic liposomes, the loading of ODN eventually changed the zeta potential to almost neutral values; the zeta potential of SG/PEG-CL-ODN complexes was −3.3 mV, when the ODN /DC-Chol was 1:1 in charge ratio.
TABLE 1 Characteristic of cationic liposomes and their ODN complexes
Transmission electron micrographs of CL and SG/PEG-CL showed spherical vesicles, as shown in . The presence of SG/PEG-DSPE had little affect on the structure and size of blank cationic liposomes. Vesicle structure and size were greatly influenced by the loading of ODN. As for CL-ODN, no spherical vesicles were observed, the presence of ODN seemed to transform small liposomes into large membrane shape. The addition of SG/PEG-DSPE reserved the spherical shape of liposome-ODN complexes, although aggregation of some of the vesicles also can be observed. The size distribution of complexes seen on electron micrographs corresponded to the values of particle size obtained by dynamic light scattering analysis. Furthermore, in blank cationic lipsomes, the boundaries of the vesicles were smooth, continuous, and intact, whereas when ODN was loaded, the boundaries were often dotted and discontinuous. Thus, the interacting ODN seemed to distort the normally smooth boundaries of the vesicle and caused aggregation of the complexes.
FIG. 1 Transmission electron microscopy of cationic liposomes and their oligonucleotides complexes. (A) Cationic liposomes without modification (CL); (B) SG/PEG-DSPE-modified liposomes (SG/PEG-CL); (C) complexes of nonmodified cationic liposomes with ODN (CL-ODN complexes); (D) complexes of SG- and PEG-DSPE-modified catiomic liposomes with ODN (SG/PEG-CL-ODN complexes).
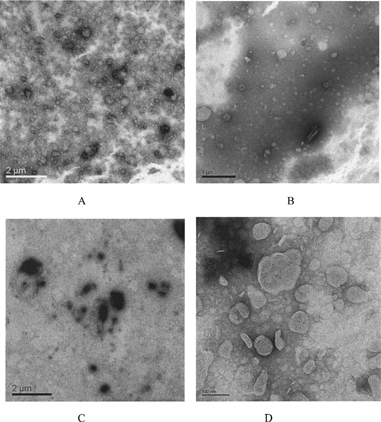
Electrophoresis Analysis
To determine the integrality of SG/PEG-CL-ODN complexes, electrophoresis analysis in used. The result of agarose electrophoresis is shown in . Compared with control (only ODN) in the first line, SG/PEG-CL-ODN complexes showed two blots, one faint blot had the same site as control, and the other was bright and still in the initial place. In the third line, the destructed complexes showed only one blot in the control level. This result demonstrated that a part of ODN interacted with cationic liposomes and formed the system in neutral charge, which could not move in the electric field. However, no ODN blot was observed after incubating the complexes with plasma (line D), and such result may relate to the interaction of complexes with plasma components.
FIG. 2 Agarose gel electrophoresis of SG/PEG-CL-ODN complexes. Fully 10 μ l containing 0.6 μ g ODN of each sample were analyzed. (A) ODN control; (B) SG/PEG-CL-ODN complexes; (C) SG/PEG-CL-ODN complexes destructed by Triton X-100; (D) SG/PEG-CL-ODN complexes incubated with 50% (v/v) rats plasma for 2 hr.
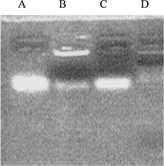
Effect of SG/PEG-CL-ODN Complexes on HepG2 2.2.15 Expression
shows the effect of cellular treatment with free ODN and SG/PEG-CL-ODN complexes on the level of expression of HBsAg and HBeAg protein in HepG2 2.2.15 cells. The percent of inhibition by ODN varied depending on the concentration of the ODN in the culture medium, especially for the SG/PEG-CL-ODN complexes. When concentration of ODN added in the medium increased from 1.25 μ M to 5.0 μ M, the inhibition percent of HBsAg by SG/PEG-CL-ODN complexes increased from 59.15% to 90.37% at 72 hr, whereas the inhibition of HBeAg increased from 42.9% to 73.43%. As for inhibition of HBsAg, the effect of free ODN and SG/PEG-CL-ODN complexes was almost the same at 24 hr after incubation, but showed significant difference in the following two time points with the decrease of inhibition effect by free ODN and the increase of inhibition effect by SG/PEG-CL-ODN complexes. The HBeAg inhibition expression showed different tendencies compared with the HBsAg; the inhibition effect of free ODN was overcome by SG/PEG-CL-ODN complexes only when incubation time reached 72 hr.
FIG. 3 Effect of SG/PEG-CL-ODN complexes on HBsAg (A) and HBeAg (B) concentration in culture medium. HepG2 2.2.15 cells were incubated at 37°C in medium containing ODN solution (the continuous line and the solid symbols) or SG/PEG-CL-ODN complexes (the dotted line and the empty symbols). The ODN concentration was 1.25 μ M (square), 2.5 μ M (round), and 5.0 μ M (triangle), respectively.
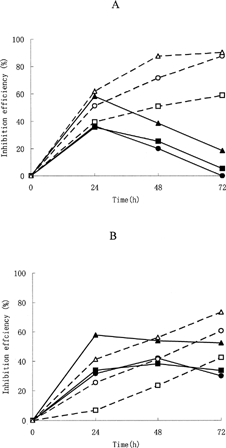
Biodistribution of 3H-Labeled Cationic Liposomes
The area under the curve (AUC) of the two preparations in different tissues after intravenous injection is shown in . No significant different in radioactivity was observed at all time points. The injected CL and SG/PEG-CL accumulated primarily in the liver (∼70% of the injected dose). Accumulation in the spleen (∼15% or 9% of the injected dose of CL and SG/PEG-CL, respectively) and in the lung (∼9% or 7% of the injected dose of CL and SG/PEG-CL, respectively) also was observed. About 2% or less of the injected dose accumulated in the heart and kidney. Thus, the majority of the injected lipid accumulated in the liver. The uptake of injected cationic liposomes by the liver was quite rapid. By 10 min postinjection, ∼70% of the injected dose had accumulated in the liver, while only ∼14%–18% of the injected dose remained in the circulation. No significant difference between two preparations was observed.
TABLE 2 AUC0−∞ values of different tissues of the 3H-labeled cationic liposomes after intravenous injection in mice at 4 μ Ci/20 g body weight
Intrahepatic Cell Distribution of 3H-Labeled Cationic Liposomes
Hepatocytes and nonhepatocytes were separated by infusion method to determine the radioactivity amount in hepatocytes and nonhepatocytes, respectively, as shown in . The radioactivity of SG/PEG-CL was significantly higher than that of CL in hepatocytes at 0.5h postinjection (p < 0.01), whereas the radioactivity of SG/PEG-CL was significantly lower than that of CL in nonhepatocytes at both 0.5 and 4 hr after intravenous injection (p < 0.01).
TABLE 3 Radioactivity (dpm) distribution of 3H-labeled cationic liposomes in hepatocytes and nonhepatocytes after intravenous injection in mice at 4 μ Ci/20 g body weight
Biodistribution of 125I-Labeled Cationic Liposomes-ODN Complexes
The biodistribution of the SG/PEG-CL-ODN complexes, labeled with trace amount of 125I-ODN, was determined by intravenous injection, compared with the ODN solution. Rapid clearance was observed in both SG/PEG-CL-ODN complexes and ODN solution. The AUC of the two preparations in different tissues is shown in . The AUC of SG/PEG-CL-ODN complexes in the liver and spleen were significantly higher than that of ODN solution (p < 0.01), meanwhile the AUC in the blood was significantly lower than ODN solution (p < 0.01), which may be attributed to the different fate of liposomal particle in vivo.
TABLE 4 AUC0−∞ values of different tissues of the 125I-labeled ODN solution and SG/PEG-CL-OND complexes after intravenous injection in mice at 6 μ Ci/20 g body weight
DISCUSSION
It is relatively straightforward to passively encapsulate anionic ODN in the aqueous space of conventional lipid vesicles. However, to achieve even modest incorporation efficiencies of 5–10% in a population of large unilamellar vesicles, extreme concentrations of lipid and ODN must be employed. The inclusion of certain amounts of cationic lipid improves the association of anionic ODN with the vesicles through electrostatic interactions. Entrapment of ODN to cationic liposomes was very efficient even when containing relatively high amounts of SG and PEG-DSPE (6.7 mol %) in the present study. Evidently, PEG coating seldom shields the positively charged liposome surface from interaction with ODN.
The introduction of PEG-DSPE is meant to improve the physical stability of cationic liposomes after complexed with ODN. It was reported that PEG modification could increase the stability of cationic liposome-ODN complexes against aggregation (Remy et al. Citation1995), and the stabilizing effect of PEG coating on the cationic liposome-ODN complexes is likely to result from preventing cross-linking of vesicles by ODN (Woodle et al Citation1992).
In our study, PEG-DPSE actually improved the stability of liposome-ODN according to characteristic results. Modification of PEG and SG significantly reduced the particle size of complexes while the spherical vesicle had been investigated in the SG-PEG-CL-ODN complexes instead of the large membrane morphology of CL-ODN complexes ( and ). However, aggregation of SG/PEG-CL-ODN complexes also was observed in our study when viewed by transmission electron micrographs. Meanwhile, we also observed that both the CL-ODN complexes and SG/PEG-CL-ODN complexes would form white precipitation after being reserved over a week (data not shown). Therefore, we postulated that although a certain amount of PEG chain had some steric effect on the interaction of vesicles, it could not completely conceal such action, PEG may have a contrary effect when used in the cationic liposomes as others reported: the polymer depletion effect of PEG chain at low concentration (2–5% mol) induce liposomes aggregation (Kuhl et al. Citation1996).
Addition of ODN sharply modified the zeta potential of SG/PEG-CL-ODN complexes to a value of −3.3 mV, an approximate neutral charge value corresponding to the charge ratio of ODN/cationic lipid in our formulation. The change of zeta potential may result in the loss of stability of the complexes compared with the blank liposmes (SG/PEG-CL) because of the disappearance of static electric repulsion, and these changes may further influence the in vivo properties of cationic liposomes and their ODN complexes.
A previous study showed that such ODN could inhibit the expression of HBsAg without significant effect on total synthesized protein in the cells (Wu andWu Citation1992). According to the results of our study, the initial concentration of ODN and incubation time appeared to be important factors in obtaining the optimum inhibition effect. With the increase of ODN concentration and incubation time, the inhibition efficiency of SG/PEG-CL-ODN complexes also increased. However, a larger amount of ODN and cationic lipid would lead to higher toxicity to the cells, therefore concentration of cationic liposome-ODN complexes should be restricted in a certain level (Cao and Huang 1991). As for the present SG/PEG-CL-ODN complex, the concentration of DC-Chol lower than 10 μ g/ml with cationic lipid/ODN at 1:1 in charge ratio was suitable (data not shown).
In the study of biodistribution of 3H-labeled cationic liposomes, almost no difference was found between the in vivo fate of CL and SG/PEG-CL. These results indicate that the PEG chain and glucose residue in SG did not necessarily improve the in vivo behavior of cationic lipid. Therefore, we estimated that the cationic charge on the surface of cationic liposomes may be a key factor in their pharmacokinetics and biodistribution. Some investigators have shown that the in vivo behavior of cationic liposomes is mainly decided by their zeta potential or the amount of cationic lipid contained in the formulation. Aoki et al. (Citation1997) showed that when the cationic liposomes containd only 5 mol% of charge lipid with small zeta potential, the behavior of the cationic liposomes would not be different from that of neutral liposomes (Zalipsky et al. Citation1994). Liposomes containing 10 mol% cationic lipid or bearing a zeta potential of ∼ +15 mV would remain in blood longer and accumulated less in liver (Aoki et al. Citation1997; Abraham, Goundalkar, and Mezei Citation1984). Cationic liposomes containing ∼50% of charged lipid were reported to accumulate in the liver rapidly after intravenous injection, and the corresponding zeta potential may be 25 mV or above (Litzinger et al. Citation1996). These findings agree with ours.
However, in the present study, with almost the same amount of radioactivity detected in the total liver between CL and SG/PEG-CL, hepatocytes targeting of SG/PEG-CL was demonstrated. These results could be attributed to the special behavior of SG to target the hepatocytes. Previous study showed that the glucose residue in SG on the surface of liposomes could be selectively recognized by hepatocytes cells (Shimizu et al. Citation1997). The result also demonstrated that the hepatocyte targeting effect of SG has not been hindered thoroughly by the PEG chain and positive charge on the surface of liposomes.
The 125I-labeled ODN solution and SG/PEG-CL-ODN complexes showed different in vivo behavior compared with SG/PEG-CL. The entrapment of cationic liposome had only a little effect on the biodistribution of ODN. One of the most important factors that may cause such a result is the interaction of SG/PEG-CL-ODN complexes and plasma or serum component. The interaction between cationic liposomes complexes and blood component has always been an obstacle in the application of cationic liposome gene delivery system in vivo (Zelphati and Szoka Citation1996). Zelphati et al.(Citation1998) demonstrated that there were two explanations for serum component inhibition: coating of the complexes with serum components and dissociation of the complex by serum component. Such interactions also may exist when the complexes are incubated with plasma.
Meyer et al. (Citation1998) reported that after mixing ODN with liposome composed of dioleoylphosphatidylethanolamine, polyethylene glycol-phosphatidylethanolamine, and a cationic lipid, although good incorporation was achieved, ∼40% of ODN dissociation occurred upon intravenous injection. A pharmacokinetic experiment indicated that even more dissociation occurred upon intravenous injection (Stuart and Allen Citation2000). We found in electrophoresis analysis that no blot of ODN was observed after incubating the SG/PEG-CL-ODN complexes with 50% plasma; this may be due to the interaction of plasma component with complexes. The biodistribution result in our study also coincided with these explanations. After the complexes were administered via tail vain, the complexes quickly dissociate in blood. Therefore, the in vivo behavior of the complexes was similar to free ODN. However, a portion of ODN was still entrapped in the cationic liposomes, so liver accumulation was observed.
From these studies we propose that interaction with blood components presents a major hurdle for efficient ODN delivery by cationic liposome in vivo. However, we also found that SG and PEG-modified cationic liposome is an effective gene delivery method for hepatocyte targeting, which appears to have a potential for gene therapy of HBV infections. Significant progress has been made in recent years in bringing ODN into the clinical setting. By reducing the interaction of plasma components with the injected complex, these barriers to ODN/DNA delivery via cationic liposomes may be overcome.
We thank Prof. Lai Wei and his research group at the Institute of Hepatology, Peking University, for assistance in cell culture. This work was supported by the National Natural Science Foundation of China, 30371265.
REFERENCES
- Abraham I., Goundalkar A., Mezei M. Effect of liposomal surface charge on the pharmacokinetics of an encapsulated model compound. Biopharm. Drug Dispos. 1984; 5: 387–398, [PUBMED], [INFOTRIEVE], [CSA]
- Akhtar S., Kole R., Juliano R. L. Stability of antisense DNA oligodeoxynucleotide analogs in cellular extracts and sera. Life Sci. 1991; 49: 169–174, [CSA], [CROSSREF]
- Aoki H., Tottori T., Sakurai F., Fuji K., Miyajima K. Effects of positive charge density on the liposomal surface on disposition kinetics of liposomes in rats. Int. J. Pharm. 1997; 156: 163–174, [CSA], [CROSSREF]
- Blum H. E., Galun E., Weizsacker F., Wands J. R. Inhibition of hepatitis B virus by antisense oligodeoxynucleotides. Lancet 1991; 337: 1230, [PUBMED], [INFOTRIEVE], [CSA], [CROSSREF]
- Crooke S. T., Graham M. J., Zuckerman J. E., Brooks D., Conklin B. S., Cummins L. L., Greig M. J., Guinosso C. J., Kornbrust D., Manoharan M., Sasmor H. M., Schleich T., Tivel K. L., Griffey R. H. Pharmacokinetic properties of several novel oligonucleotide analogs in mice. J. Pharmacol. Exp. Ther. 1996; 277: 923–937, [PUBMED], [INFOTRIEVE], [CSA]
- Dusheiko G. M. Treatment and prevention of chronic viral hepatitis. Pharmacol. Therapeut. 1995; 65: 47–73, [CSA], [CROSSREF]
- Gao X., Hang L. A novel cationic liposome reagent for efficient transfection of mammalian cells. Biochem. Biophys. Res. Commun. 1991; 179: 280–285, [PUBMED], [INFOTRIEVE], [CSA], [CROSSREF]
- Hope M. J., Mui B., Ansell S., Ahkong Q. F. Cationic lipids, phosphatidylethanolamine and the intracellular delivery of polymeric, nucleic acid-based drugs. Mol. Membr. Biol. 1998; 15: 1–14, [PUBMED], [INFOTRIEVE], [CSA]
- Koike K., Hara T., Aramak Y., Takada S., Tsuchiya S. Receptor-mediated gene transfer into hepatic cells using asialoglycoprotein-labeled liposomes. Ann. NY Acad. Sci. 1994; 716: 331–333, [PUBMED], [INFOTRIEVE], [CSA]
- Kuhl T., Guo Y., Alderfer J. L., Berman A. D., Leckband D., Israelachvili J., Hui S. W. Direct measurement of polyethylene glycol induced depletion attraction between lipid bilayers. Langmuir 1996; 12: 3003–3014, [CSA], [CROSSREF]
- Litzinger D. C., Brown J. M., Wala I., Kaufman S. A., Van G. Y., Farrell C. L., Collins D. Fate of cationic liposomes and their complex with oligonucleotide in vivo. Biochim. Biophys. Acta 1996; 1281: 139–149, [PUBMED], [INFOTRIEVE], [CSA]
- Meyer O., Kirpotin D., Hong K., Sternberg B., Park J. W., Woodle M. C., Papahadjopoulos D. Cationic liposomes coated with polyethlene glycol as carriers for oligonucleotides. J. Biol. Chem. 1998; 273: 15621–15627, [PUBMED], [INFOTRIEVE], [CSA], [CROSSREF]
- Offensperger W. B., Offensperger S., Walter E., Teubner K., Lgloi G., Blum H. E., Gerok W. In vivo inhibition of duck hepatitis B virus replication and gene expression by phosphorothioate modified antisense oligodeoxynucleotides. EMBO J. 1993; 12: 1257–1262, [PUBMED], [INFOTRIEVE], [CSA]
- Remy J. S., Kichler A., Mordvinov V., Schuber F., Behr J. P. Targeted gene transfer into hepatoma cells with lipopolyaminecondensed DNA particles presenting galactose ligands: a stage toward artificial viruses. Proc. Natl. Acad Sci. USA 1995; 92: 1744–1748, [PUBMED], [INFOTRIEVE], [CSA]
- Soni P. N., Brown D., Saffie R., Savage K., Moore D., Gregoriadis G., Dusheiko G. M. Biodistribution, stability, and antiviral efficacy of liposome-entrapped phosphorothioate antisense oligodeoxynucleotides in ducks for the treatment of chronic duck hepatitis B virus infection. Hepatology 1998; 28: 1402–1410, [PUBMED], [INFOTRIEVE], [CSA], [CROSSREF]
- Shimizu K., Maitani Y., Takahashi N., Takayama K., Nagai T. Association of liposomes containing a soybean-derived sterylglucoside mixture with rat primary cultured hepatocytes. Biol. Pharm. Bull. 1998; 21: 818–822, [PUBMED], [INFOTRIEVE], [CSA]
- Shimizu K., Maitani Y., Takayama K., Nagai T. Formulation of liposomes with a soybean-derived sterylglucoside mixture and cholesterol for liver targeting. Biol. Pharm. Bull. 1997; 29: 81–886, [CSA]
- Stuart D. D., Allen T. M. A new liposomal formulation for antisense oligodeoxynucleotides with small size, high incorporation efficiency and good stability. Biochim. Biophys. Acta 2000; 1463: 219–229, [PUBMED], [INFOTRIEVE], [CSA]
- Takehara T., Hayashi N., Miyamoto Y., Yamamoto M., Mita E., Fusamoto H., Kamada T. Expression of the hepatitis C virus genome in rat liver after cationic liposome-mediated in vivo gene transfer. Hepatology. 1995; 21: 746–751, [PUBMED], [INFOTRIEVE], [CSA], [CROSSREF]
- Wagner R. W. Gene inhibition using antisense oligodeoxynucleotides. Nature 1994; 372: 333–335, [PUBMED], [INFOTRIEVE], [CSA], [CROSSREF]
- Woodle M. C., Collins L. R., Sponsler E., Kossovsky N., Papahaduopoulos D., Martin F. J. Sterically stabilized liposomes: reduction in electrophoretic mobility but not electrostatic surface potential. Biohpys. J. 1992; 61: 902–910, [CSA]
- Wu G. Y., Wu C. H. Specific inhibition of hepatitis B viral gene expression in vitro by targeted antisense oligonucleotides. J. Biol. Chem. 1992; 267: 12436–12439, [PUBMED], [INFOTRIEVE], [CSA]
- Zalipsky S., Brandeis E., Newman M. S., Woodle M. C. Long circulating, cationic liposomes containing amino-PEG-phosphatiylethanolamine. FEBS Lett. 1994; 353: 71–74, [PUBMED], [INFOTRIEVE], [CSA], [CROSSREF]
- Zelphati O., Szoka F. C., Jr. Intracellular distribution and mechanism of delivery of oligomucleotides mediated by cationic lipid. Pharm. Res. 1996; 13: 1367–1372, [PUBMED], [INFOTRIEVE], [CSA], [CROSSREF]
- Zelphati O., Uyechi L. S., Barron L. G., Szoka F. C., Jr. Effect of serum components on the physicochemical properties of cationic lipid/oligonucleotide complexes and on their interaction with cells. Biochim. Biophys. Acta 1998; 1390: 119–133, [PUBMED], [INFOTRIEVE], [CSA]