Abstract
Despite its strong antitumor activity, paclitaxel (Taxol®) has limited clinical applications due to its low aqueous solubility and hypersensitivity caused by Cremophor® EL and ethanol which is the vehicle used in the current commercial product. In an attempt to develop a pharmaceutically acceptable formulation that could replace Taxol®, a paclitaxel incorporated liposome has been constructed to improve solubility and physicochemical stability. The effect of various components of the liposome, including cholesterol and lipid, on the solubility and entrapment efficiency (EE) of paclitaxel was systematically investigated. The results showed that 5% (v/v) of polyethylene glycol 400 in the hydration medium of liposome significantly increased the solubility (up to 3.39 mg/mL) as well as the EE and the paclitaxel content in the liposome formulation composed of 10% (w/v) of S100PC with cholesterol (cholesterol-to-lipid molar ratio = 10:90). When sucrose (sugar-to-lipid molar ratio = 2.3) was added as a lyoprotectant during the freeze-drying of the liposome, physicochemical stability of liposome was significantly improved. Moreover, the cytotoxicity of the final liposome formulation against MDA-MB-231 human breast cancer cell line was not significantly different from that of Taxol®. The enhanced aqueous solubility as well as the physicochemical stability of paclitaxel in the liposome formulation developed in this study could be a safer and effective alternative to the Cremophor® EL and ethanol formulation.
Paclitaxel is a diterpenoid natural product (Wani et al. Citation1971) isolated from the Pacific Yew (Taxus brevifolia) that has demonstrated significant antitumor activity against various solid tumors, including advanced ovarian carcinoma, metastatic breast cancer, nonsmall cell lung cancer, and head and neck carcinomas (Chang et al. Citation2001; Ishitobi, Shin, and Kikkawa Citation2001; Zhang et al. Citation2005b). Due to its low aqueous solubility (less than 2 μ g/mL), paclitaxel is currently formulated in a vehicle composed of a 1:1 blend of Cremophor® EL (polyethoxylated castor oil) and ethanol, which is diluted 5–20-fold in normal saline or dextrose solution (5%) for administration. However, hypersensitivity problems related with Cremophor® EL and ethanol have been reported (Gelderblom et al. Citation2001; Zhang et al. Citation2005a). Thus, attempts to develop soluble paclitaxel formulations without the Cremophor® EL vehicle has been made. Among them, liposomes seem to be one of the most promising approaches, because of good biocompatibility and low toxicity.
Encapsulation of drugs into liposomes alters the pharmacokinetics and biodistribution, resulting in an increased therapeutic efficacy and/or a decreased toxicity (Allen, Hansen, and Lopes de Menezes Citation1995). A liposome formulation of doxorubicin has been approved by the FDA for Kaposi's sarcoma or ovarian carcinoma (Martin Citation1998). However, several studies have shown that it is possible to eliminate the Cremophor® EL vehicle by encapsulating paclitaxel in liposomes (Sharma, Mayhew, and Straubinger Citation1993; Straubinger et al. Citation1993; Singla, Garg, and Aggarwal Citation2002). But low solubility of paclitaxel in liposomal solution together with poor physical stability of the liposome itself have hindered the development and clinical application of the paclitaxel liposomal formulation.
In a study by Meerum Terwogt et al. (Citation1997), among various cosolvent systems 50% triacetin in emulsion increased the solubility of paclitaxel up to 75 mg/mL. Unfortunately, triacetin itself was too toxic for intravenous administration. The polyethylene glycol (PEG) family has played an important role in enhancing the solubility of insoluble drugs through the formation of prodrugs or by direct solubilizing effect. When prodrugs of paclitaxel were prepared by conjugating PEG as a 2′-ester, the solubility increased up to 650 mg/mL while maintaining the cytotoxicity of the parent drug (Panchagnula Citation1998). Cosolvent system with PEG 400 also increased the solubility of paclitaxel up to 16 mg/mL with low toxic effects (Adams, Flora, and Goldspiel Citation1993), but precipitation and cloudiness were noted during the dilution process for infusions. Therefore, to develop a pharmaceutically acceptable liposomal formulation with enhanced solubility for paclitaxel thereby replacing Cremophor® EL and ethanol, the effects of various components of the liposome including PEG 400 and sucrose were systematically investigated.
MATERIALS AND METHODS
Paclitaxel was purchased from Taihua Corporation (Xi'an, China). Soybean phosphatidylcholines (S75PC and S100PC), egg phosphatidylcholines (E80PC and E100PC), and hydrogenated soybean phosphatidylcholine (HPC) were generous gifts from Lipoid Company (Ludwigshafen, Germany). S75PC and S100PC contain 20∼ 25% and 0% phosphatidyl-ethanolamine (PE), respectively, while E80PC and E100PC include 20% and 0% PE, respectively. Distearoyl L-3-phosphatidylcholine (DSPC) and dipalmitoyl L-3-phosphatidylcholine (DPPC) were purchased from Doosan Biotech Corporation (Seoul, Korea). Cholesterol (CH) was obtained from Tokyo Kasei Kogyo (Japan). Stearylamine, phosphatidylserine, sucrose, and 3-(4,5-dimethyl-2-tetrazolyl)-2,5-diphenyl tetrazolium bromide (MTT) were obtained from Sigma (St. Louis, MO, USA). Millipore polycarbonate membranes (1.2, 0.4 and 0.2 μ m pore size) were purchased from Millipore Corporation (Isopore™, Ireland). All other reagents were analytical grade or higher and obtained from commercial sources.
Liposome Preparation
Paclitaxel was incorporated in the liposome by the modified thin-film hydration method (Zhang et al. Citation2005a). Briefly, the hydrophobic excipients, such as paclitaxel and lipids (i.e, phosphatidylcholine and CH), were first dissolved in chloroform and then dried in a rotary evaporator (BÜCHI Labortechnik, Flawil Switzerland) under an aspirate vacuum and a water bath (BÜCHI B-490) with temperature maintained at 40°C. The thin film layer formed was flushed with nitrogen gas for 5 min and maintained overnight under vacuum to remove trace of chloroform. The thin film was resuspended in phosphate buffer saline (PBS, pH 4.0) containing Tween 80 (3%, v/v), and PEG 400 (0, 2, or 5%, v/v) by rotating the flask at 300 rpm until the lipid film was completely hydrated. Then, the liposome dispersion was serially passed through 1.2, 0.4, and finally 0.2 μ m pore size filters (Isopore™ membrane filter) under nitrogen gas using an extruder (Northern Lipids, Canada).
Unentrapped paclitaxel was removed from the liposome dispersion by centrifuging at 50,000 rpm for 30 min. Supernatant was discarded, and the liposome pellet was washed two times with PBS. Liposome particles were then suspended in distilled water containing various concentrations (0–2.7, molar ratio to lipid) of sucrose and were freeze-dried (Laboratory floor model freeze-dryer, IlShin FD5512, Korea). The final liposome powders were stored in a tight container at 4°C for further experiments.
Characterization of Liposomes
Particle Size and Zeta-Potential of Liposomes
The mean particle size and particle size distribution of various liposome formulations were determined using the Submicron Particle Sizer (Autodilute Model 370, Nicomp) after resuspending and diluting the freeze-dried liposomes in distilled water. The particle size distribution was processed with a computerized inspection system. Data were automatically analyzed by Gaussian analysis (the least-squares quadratic: cumulates) with the Nicomp 370 software and reported as volume weighted distribution and represented as the mean of three measurements.
The zeta potential of the liposomes resuspended in distilled water was measured by an electrophoretic light scattering spectrophotometer (ELS-8000, Otsuka Electronics Co., Japan) at room temperature after appropriate dilution with distilled water.
Entrapment Efficiency and Content of Paclitaxel in Liposomes
The entrapment efficiency (EE) is defined as the ratio of the amount of the paclitaxel encapsulated in the liposome to that of the total paclitaxel in liposome suspension. The amount of paclitaxel encapsulated in liposomes was measured following the ultracentrifuge method with slight modification (Shieh et al. Citation1997). Briefly, aliquots (0.1 mL each) of liposome suspensions diluted to 1.1 mL by PBS (pH 7.4) immediately after preparation were centrifuged at 1,000 rpm for 10 min to remove any paclitaxel particle already released from the liposomes. Then 1.0 mL of liposome supernatant was precipitated by ultracentrifugation at 50,000 rpm for 30 min (Beckman XL-100, Fullerton, CA, USA). After removing the supernatant by decantation, the precipitate (i.e, liposome pellet) was washed twice with phosphate buffered saline (PBS, pH = 7.4). The liposome pellets were then dissolved with 6 mL of the mixture (50:50, v/v) of water and organic solvents, composed of isopropanol, ether, and ethanol (2:1:2, v/v/v). The amount of paclitaxel was determined by using a high performance liquid chromatography (HPLC) after appropriate dilution with the mixed solvent that was used to destroy the liposome pellet. An aliquot (0.1 mL each) of liposome suspension also was dissolved with the same mixed solvent to determine the total amount of paclitaxel in the liposome suspension, and then EE was calculated from the following equation:
An aliquot (50 mg each) of freeze-dried liposomes was dissolved with the same mixed solvent (4 mL) to determine the content of paclitaxel in the liposome after appropriate dilution with the same mixed solvent, using the following equation:
The EE and content were determined in three separately prepared liposome suspensions and were expressed as the mean ± standard deviation.
HPLC Analysis of Paclitaxel
Paclitaxel concentration in all samples was directly analyzed by a Waters HPLC system (Milford, MA, USA) equipped with a dual pump (Waters 515), UV detector (Waters 2487), and automatic injector (Waters 717 plus). A reverse phase LiChrospher®100 RP-18 column (250 × 4 mm, 5 μ m, Merck, Germany) was used at room temperature. The detector wavelength was set at 227 nm and injection volume was 20 μ L. The mobile phase was a mixture of acetonitrile and water (50:50, v/v) at a flow rate of 1.0 mL/min.
Cytotoxicity Study
MDA-MB-231 human breast cancer cell line was purchased from the American Type Culture Collection (Manassas, VA, USA), and was cultured in Dulbecco's Modified Eagle Medium (DMEM, Gibco BRL) supplemented with 10% (v/v) heat-inactivated fetal bovine serum (FBS) and antibiotics (100 U/mL penicillin and 0.1 mg/mL streptomycin) at 37°C and 5% CO2 atmosphere.
The cytotoxicity of paclitaxel encapsulated in various liposomes was compared with that of the free paclitaxel solution (Taxol®) by using the MTT assay (Mosmann Citation1983). Taxol® (6 mg/mL) was prepared by dissolving paclitaxel in 50:50 (v/v) mixture of Cremophor® EL and dehydrated alcohol and was diluted to make various concentrations. MTT solution (0.5 mg/mL) was first prepared by dissolving in PBS, followed by filtration (0.2 μ m pore size, Sartorius, Germany) to sterilize and remove insoluble residues.
MDA-MB-231 cells were suspended in culture medium at a density of 2 × 104 cells/well in 96-well flat-bottom tissue-culture plates. The cells were incubated at 37°C and 5% CO2 atmosphere for 24 hr, during which cells were attached and resumed to grow. Freeze-dried liposomes or Taxol® were diluted with culture medium (i.e, DMEM) to make various concentrations of paclitaxel and were added in triplicate (0.2 mL). Control wells were treated with equivalent volume of paclitaxel-free media. After 24 hr, the supernatant was removed, and MTT and culture medium (100 μ L each) were added to each well and incubated for 4 hr at 37°C and 5% CO2 atmosphere. The unreduced MTT and medium were then discarded. Each well was washed with 200 μ L of PBS, and 200 μ L of DMSO was added to dissolve the MTT formazan crystals. Plates were shaken for 20 min and absorbance was read at 560 nm using a microplate reader (Molecular Devices Corporation, USA). The IC50 values (i.e, concentration resulting in 50% growth inhibition) of paclitaxel were graphically calculated from concentration-viability curves, considering the optical density of the control well as 100% viable (Sharma et al. Citation1996).
RESULTS AND DISCUSSION
Effect of Tween 80 in Hydration Medium
To improve the solubility of paclitaxel and thereby eliminate the Cremophor® EL and ethanol in the formulation, effect of various components of liposome, such as CH content, lipid type, and PEG 400, was investigated systematically. In our preliminary study, the addition of 3% (v/v) Tween 80 in the hydration medium completely hydrated the dry lipid film within 2 h as well as stabilized particle size of the liposome for at least 1 week (data not shown). Since it also was reported that paclitaxel is most stable in a pH 3–5 solution (Dordunoo and Burt Citation1996; Koziara et al. Citation2004), PBS (pH 4.0 adjusted with 1.0 M HCl) solution with 3% (v/v) Tween 80 was selected as a control hydration medium for the preparation of liposomes in this study.
The mean zeta potential of liposome solution including 3% (v/v) Tween 80 was −7.87 and −7.93 mV for S75PC and S100PC, respectively, whereas −6.63 mV was reported for the liposome prepared with a neutral lipid and Tween 80 (Lee et al. Citation2005). The reason for the lower zeta potential in this study could be due to the acidic medium used for the hydration process. Although Tween 80 is a nonionic surfactant, it can be partially hydrolyzed in acidic or basic condition, making the liposome surface negatively charged. Moreover, the negative charge on the liposome surface also can decrease the attraction among the liposome particles by offering repulsive force and thereby stabilizing the liposome by preventing their aggregation.
Effect of Cholesterol Content
It is well known that regardless of the liposome surface charge, cholesterol-rich liposome is more stable than cholesterol-poor or cholesterol-free liposomes both in vivo and in vitro (Kirby, Clarke, and Gregoriadis Citation1980; Senior and Gregoriadis Citation1982). However, cholesterol can increase the ordered arrangement of lipid membrane. Thus high content of cholesterol in the liposome can decrease the flexibility of membrane in the preparation process and thereby hinder the penetration of paclitaxel into the lipid bilayer (Bi and Ping Citation1999). As shown in , the entrapment efficiency of paclitaxel in liposome also decreased with the increase in the molar percent of cholesterol in the formulation. Highly lipophilic paclitaxel molecules may compete with cholesterol for the hydrophobic space in the lipid bilayer. Since the addition of 10% molar ratio of cholesterol already stabilized liposome particles for up to 10 weeks () and did not significantly decrease the EE of paclitaxel (), the molar ratio of phosphatidylcholine and cholesterol was fixed to be 90:10 in the preparation of liposomes in this study.
FIG. 1 The effect of CH-to-lipid molar ratio on (A) the entrapment efficiency (%) of paclitaxel in the liposomes and (B) the changes of the mean particle size of liposome containing 10% cholesterol (molar percent to lipid). The liposomes were prepared using 5.4% (w/v) S100PC with various CH-to-lipid molar ratio of cholesterol. The amount of paclitaxel loaded in the formulation was 0.6 mg/mL, and the hydration medium was PBS (pH 4.0) containing 3.0% (v/v) of Tween 80.
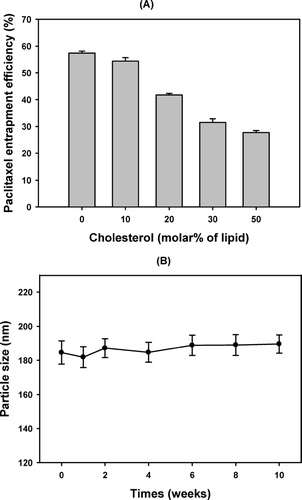
Effect of Paclitaxel and Lipid Content
shows that when the amount of paclitaxel loaded in the formulation with 5.4% (v/v) lipid was increased up to 2.00 mg/mL, the amount of paclitaxel encapsulated in the liposome solution also increased up to 1.82 mg/mL and 1.56 mg/mL for S75PC and S100PC, respectively, while the EE of paclitaxel remained constant (∼55%). However, when 2.50 mg/mL of paclitaxel was loaded in the same formulation (i.e, the molar ratio of lipid-to-paclitaxel was lower than 30), paclitaxel easily precipitated during the extrusion and/or storage, resulting in the decrease of EE below 40% and increase of the particle size. This implies that the lipid-to-paclitaxel molar ratio needs to be maintained at higher than 30 for the lipid to form enough space for paclitaxel. A similar result has been reported (Zhang et al. Citation2005a). Moreover, the increase of the S100PC and S75PC content resulted in a significant increase in EE up to 84.65% and 76.87%, respectively, probably since higher lipid content offers more lipophilic space for paclitaxel.
TABLE 1 Effect of lipid and paclitaxel contents on the entrapment of paclitaxel in the liposome
However, when the lipid content was higher than 12%, the lipid film was not completely hydrated and it was difficult for the liposome suspension to pass through the extrusion membrane due to its high viscosity. For further studies, the lipid content was fixed to be 10% (w/v), which maintains the lipid-to-paclitaxel molar ratio at 32.6 when 3.5 mg/mL of paclitaxel is loaded in the formulation.
Effect of Lipid Type
shows the effect of lipid types on EE of paclitaxel and its content in liposome (%). When 3.5 mg/mL of paclitaxel was loaded in 10% (w/v) of S100PC, the highest paclitaxel content in liposome (2.09%) and EE of paclitaxel (70.83%) were obtained. Moreover, it is noteworthy that the paclitaxel concentration in liposome solution was 3.39 mg/mL (), which exceeded the highest concentration (1.5 mg/mL) reported in the literature (Crosasso et al. Citation2000).
FIG. 2 Effect of lipid types on the entrapment efficiency (%) of paclitaxel and paclitaxel content in liposome (%). The liposomes were prepared using 10% (w/v) of various lipids with cholesterol (CH-to-lipid molar ratio = 10:90) and were loaded with 3.5 mg/mL paclitaxel (lipid-to-paclitaxel molar ratio is 32.6). The hydration medium was PBS (pH 4.0) containing 3.0% (v/v) of Tween 80.
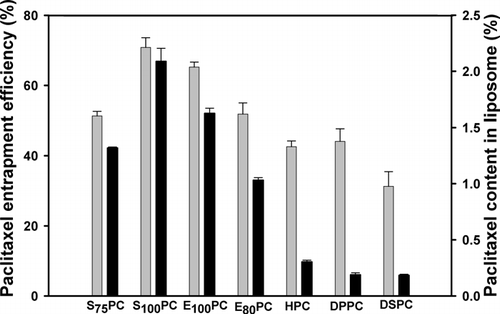
TABLE 2 Effect of PEG 400 on the entrapment of paclitaxel in the liposome
However, HPC, DSPC, and DPPC which are highly saturated lipids due to the high rigidity of the lipid bilayers of the prepared liposomes, seem to have low flexibility for paclitaxel to be loaded in the bilayers. Only 0.2% to 0.3% of paclitaxel contents in liposome were observed for these liposomes (). Moreover, paclitaxel encapsulated in such liposomes is known to be easily released from the lipid bilayers, resulting in precipitation within 24 hr (Zhang et al. Citation2005a).
Effect of PEG 400
The addition of cosolvent(s) is probably one of the most common approaches used to solubilize insoluble drugs such as paclitaxel. It was reported that 75% (v/v) of PEG 400 in water increased the solubility of paclitaxel up to 16 mg/mL (Adams et al. Citation1993). However, precipitation on the necessary dilution during preparation of the infusion fluid still remains a major problem when cosolvent system is used (Meerum Terwogt et al. Citation1997).
Liposome particles prepared with 10% (w/v) of S100PC and 3.5 mg/mL of paclitaxel were separated and freeze dried, and the actual paclitaxel contents in freeze dried liposome particles were determined. To further increase the content of paclitaxel in the liposome particles by enhancing its solubility in aqueous inner phase of liposomes, PEG 400 (2 or 5%, v/v) was added in the hydration medium (i.e, PBS, pH 4.0) during the preparation of liposomes. It is interesting to note that both the content of paclitaxel in liposome particles and the EE of paclitaxel significantly increased up to 2.53% (p < 0.05) and 89.26% (p < 0.05), respectively, when 5% (v/v) of PEG 400 was added in hydration medium. But there was no significant (p > 0.05) change in zeta potential and paclitaxel in liposome solution (). Thus, we suggest that PEG 400 increased the paclitaxel distribution in the interior water phase of liposome, thereby increasing the paclitaxel content in freeze-dried liposome particles. However, higher than 5% of PEG increased the viscosity of the liposome solution and made it difficult to pass through the extruder.
Effect of Sucrose on Freeze Drying
The effect of sucrose as a protecting agent of liposome was studied by adding various molar ratios of sucrose before freezing drying the liposome particles, to observe the changes of the mean particle size after reconstitution. When the freeze-dried liposome composed of 10% (w/v) S100PC, cholesterol (CH-to-lipid molar ratio = 10:90), and 3.5 mg/mL of paclitaxel was resuspendsed with normal saline solution, the mean particle size of liposome increased from 177.3 (± 6.9) to 465.2 (± 28.8) nm (). However, when the sucrose-to-lipid molar ratio increased up to 2.3, the difference between the mean particle size before and after freeze drying became insignificant, and the particle size of liposome was constant for at least 48 hr at room temperature (). In a separate study, mannitol of the same amount was added but did not prove to be as effective as sucrose in protecting the liposome during the freeze drying process (data not shown). Thus, sucrose (sugar-to-lipid molar ratio = 2.3) was chosen as the lyoprotectant in the final formulation.
FIG. 3 (A) Effect of sucrose on the change of the mean particle size of liposome before (•) and after (ˆ) freeze drying. The liposomes were prepared using 10%% (w/v) of S100PC with cholesterol (CH-to-lipid molar ratio = 10:90) and were loaded with 3.5 mg/mL paclitaxel. The hydration medium was PBS (pH 4.0) containing 3.0% (v/v) Tween 80 and 5% (v/v) PEG 400. Liposome particles were separated by ultrafiltration and were suspended with distilled water containing various concentrations (molar ratio to lipid) of sucrose before freeze drying. (B) Change of particle size of liposome containing sucrose as a lyoprotectant (sugar-to-lipid molar ratio = 2.3) at room temperature when freeze-dried liposome powder was reconstituted with 0.9% (w/v) NaCl solution.
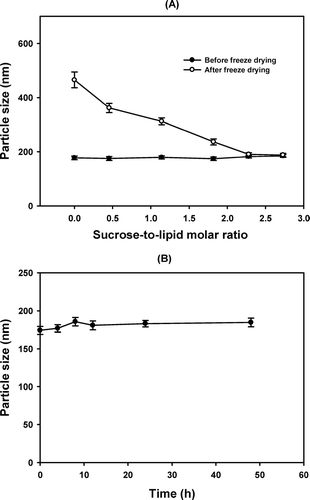
Cytotoxicity of Liposome-Based Paclitaxel Formulations
The cytotoxicity of free paclitaxel in a 1:1 blend of Cremophor® EL and ethanol (Taxol®) against MDA-MB-231 cell line was compared with that of various paclitaxel-loaded liposomes in an MTT assay. The liposome prepared by 10% (w/v) S100PC with CH (CH-to-lipid molar ratio = 10:90), 3.5 mg/mL of paclitaxel, and 5% PEG 400 (v/v) showed similar cytotoxicity (p > 0.05) to that of the free paclitaxel solution (). This result is contradicts the expectation that the liposome formulation would make the drug less cytotoxic. The possible explanation for this would be that paclitaxel was completely released from the formulation since the assay was conducted 24 hr after incubation.
TABLE 3 Cytotoxicity of paclitaxel in 1:1 blend of Cremophor® EL and ethanol (Taxol®) and in various liposome formulations against MDA-MB-231 human breast cancer cells after 24 h incubation
It is interesting to note that the addition of a positively charged lipid (i.e, stearylamine) significantly increased cytotoxicity of the liposome compared with free paclitaxel and liposome without charged lipid, whereas negatively charged lipid (i.e, phosphatidylserine) significantly decreased cytotoxicity. It is well known that positively charged liposomes have higher affinity against tumor cells compared with the neutral or negatively charged liposomes (Shinkai et al. Citation1996; Ito et al. Citation2004). Thus they seemed to make the paclitaxel molecules easily uptaken by the tumor cells resulting in increased cytotoxicity.
CONCLUSION
The addition of 5% (v/v) PEG 400 in the hydration medium of liposome significantly increased the solubility (up to 3.39 mg/mL), EE, and paclitaxel content in the liposome formulation composed of 10% (w/v) of S100PC with cholesterol (CH-to-lipid molar ratio = 10:90). Moreover, freeze drying the liposome with sucrose as a lyoprotectant improved its physicochemical stability. The cytotoxicity of the final liposome formulation against MDA-MB-231 human breast cancer cell line was equivalent to that of Taxol®. Thus the liposome product may be able to replace the Cremophor®EL and ethanol formulation.
The authors thank Lipoid Company (Germany) for supplying the phosphatidylcholine. This research was financially supported by the Ministry of Science and Technology (M1052801004-06N2801-00410) in Korea.
REFERENCES
- Adams J. D., Flora K. P., Goldspiel B. R. Taxol: a history of pharmaceutical development and current pharmaceutical concerns. J. Natl. Cancer Inst. Monogr. 1993; 15: 141–147
- Allen T. M., Hansen C. B., Lopes de Menezes D. E. Pharmacokinetics of long circulating liposomes. Adv. Drug Deliv. Rev. 1995; 16: 267–284
- Bi D. Z., Ping Q. N. Pharmaceutics, 3rd ed. People's Health Publication, Beijing 1999
- Chang A. Y., Rubins J., Asbury R., Boros L., Hui L. F. Weekly paclitaxel in advanced non-small cell lung cancer. Semi. Oncol. 2001; 28: 10–13
- Crosasso P., Ceruti M., Brusa P., Arpicco S., Dosio F., Cattel L. Preparation, characterization and properties of sterically stabilized paclitaxel-containing liposomes. J. Control. Rel. 2000; 63: 19–30
- Dordunoo S. K., Burt H. M. Solubility and stability of taxol: effects of buffers and cyclodextrins. Int. J. Pharm. 1996; 133: 191–201
- Gelderblom H., Verweij J., Nooter K., Sparreboom A. Cremophor EL: the drawbacks and advantages of vehicle selection for drug formulation. Eur. J. Cancer 2001; 37: 1590–1598
- Ishitobi M., Shin E., Kikkawa N. Metastatic breast cancer with resistance to both anthracycline and docetaxel successfully treated with weekly paclitaxel. Int. J. Clin. Oncol. 2001; 6: 55–58
- Ito A., Kuga Y., Honda H., Kikkawa H., Horiuchi A., Watanabe Y., Kobayashi T. Magnetite nanoparticle-loaded anti-HER2 immunoliposomes for combination of antibody therapy with hyperthermia. Cancer Lett. 2004; 212: 167–175
- Kirby C., Clarke J., Gregoriadis G. Effect of the cholesterol content of small unilamellar liposomes on their stability in vivo and in vitro. Biochem. J. 1980; 186: 591–598
- Koziara J. M., Lockman P. R., Allen D. D., Mumper R. J. Paclitaxel nanoparticles for the potential treatment of brain tumors. J. Control. Rel. 2004; 99: 259–269
- Lee E. H., Kim A., Oh Y. K., Kim C. K. Effect of edge activators on the formation and transfection efficiency of ultradeformable liposomes. Biomaterials 2005; 26: 205–210
- Martin F. J. Clinical pharmacology and antitumor efficacy of DOXIL (pegylated liposomal doxorubicin). Medical Applications of Liposomes, D. D. Lasic, D. Papahadjopoulos. Elsevier Science Publishers, Amsterdam 1998; 635–688
- Meerum Terwogt J. M., Nuijen B., Ten Bokkel Huinink W. W., Beijnen J. H. Alternative formulations of paclitaxel. Cancer Treat. Rev. 1997; 23: 87–95
- Mosmann T. Rapid colorimetric assay for cellular growth and survival: application to proliferation and cytotoxicity assays. J. Immunol. Meth. 1983; 65: 55–63
- Panchagnula R. Review: pharmaceutical aspects of paclitaxel. Int. J. Pharm. 1998; 172: 1–15
- Senior J., Gregoriadis G. Stability of small unilamellar liposomes in serum and clearance from the circulation: the effect of the phospholipid and cholesterol components. Life Sci. 1982; 30: 2123–2136
- Sharma A., Mayhew E., Straubinger R. M. Antitumor effect of taxol-containing liposomes in a taxol-resistant murine tumor model. Cancer Res. 1993; 53: 5877–5881
- Sharma A., Sharma U. S., Straubinger R. M. Paclitaxel-liposomes for intracavitary therapy of intraperitoneal P338 leukemia. Cancer Lett. 1996; 107: 265–272
- Shieh M. F., Chu I. M., Lee C. J., Kan P., Hau D. M., Shieh J. J. Liposomal delivery system for taxol. J. Ferment. Bioeng. 1997; 83: 87–90
- Shinkai M., Yanase M., Honda H., Wakabayashi T., Yoshida J., Kobayashi T. Intracellular hyperthermia for cancer using magnetite cationic liposomes: in vitro study. J. Cancer Res. 1996; 87: 1179–1183
- Singla A. K., Garg A., Aggarwal D. Paclitaxel and its formulations. Int. J. Pharm. 2002; 235: 179–192
- Straubinger R. M., Sharma A., Murray M., Mayhew E. Novel taxo1 formulations: taxol-containing liposomes. J. Natl. Cancer Inst. Monogr. 1993; 15: 69–78
- Wani M. C., Taylor H. L., Wall M. E., Caggon P., McPhail A. T. Plant antitumor agents: VI The isolation and structure of taxol, a novel anti leukemic and antitumor agent from Taxus brevifolia. J. Am. Chem. Soc. 1971; 93: 2325
- Zhang J. A., Anyarambhatla G., Ma L., Ugwu S., Xuan T., Sardone T., Ahmad I. Development and characterization of a novel Cremophor®EL free liposome-based paclitaxel (LEP-ETU) formulation. Eur. J. Pharm. Biopharm. 2005a; 59: 177–187
- Zhang X. F., Li Y. X., Chen X. S., Wang X. H., Xu X. Y., Liang Q. Z., Hu J. L., Jing X. B. Synthesis and characterization of the paclitaxel/MPEG-PLA block copolymer conjugate. Biomaterials 2005b; 26: 2121–2128