Abstract
We prepared different neutral and positively charged niosomal formulations containing sorbitan esters for entrapment of caffeine. Drug entrapment reduced following the incorporation of positively charged molecule. Furthermore, the span 60-containing niosomes showed the highest drug encapsulation efficiency due to solid-state nature of this surfactant's bilayers. There was a regular relationship between lipophilicity (HLB values) of surfactants and mean particle sizes; increasing the HLB value resulted in larger niosomes. By means of diffusion experiments with Franz diffusion cells, the effects of different vesicular components and that of the positive charge on the release of caffeine from various vesicle formulations were studied. Obtained results indicate that a combined erosion-diffusion mechanism regulates the permeation of caffeine through cellulose acetate membranes. High encapsulation efficiency, appropriate size distribution, and good vesicular stability, especially in solid state niosomes, make this type of vesicular systems a good alternative to liposomes for topical delivery of caffeine.
Keywords :
Caffeine is a methylxanthine derivative with various pharmacological activities, such as blockade of adenosine receptors, inhibition of phosphodiesterase, inhibition of 5′-nucleotidase, and modulation of intracellular calcium movement, which may affect neuronal functions (Sawynok and Yaksh Citation1993). Topical application of caffeine formulations has been extensively studied in recent years in different local or systemic disorders; Amato, Isenschemid, and Huppi (Citation1991) have shown percutaneous absorption to be a safe and useful approach for treatment of apnea in infants. Caffeine also can apply topically to stimulate lipolyses in epidermal fat cells (Essig, Costill, and Van Handel Citation1980) and in treatment of hyperproliferative skin diseases (Touitou et al. Citation1994). Also, it has been demonstrated that topical treatment of HSV-infected skin of the mouse with caffeine gel retarded the appearance of zosteriform skin lesions and that 10% caffeine was as potent as 5% acyclovir, an antiherpes virus agent (Yamamura et al. Citation1996). Shiraki et al. (Citation1998) have reported that topical caffeine treatment of the infected skin inhibited sensory abnormality, especially hypoalgesia, induced by HSV infection. Topical application of caffeine also has been shown to decrease the number of nonmalignant and malignant skin tumors in mice (Lu et al. Citation2002) and enhance apoptosis in the tumors by a p53-independent pathway (Lu et al. Citation2004).
In the formulation of topical dosage forms, great attention has been devoted to new structures that can ensure either adequate localization of the drug within the skin to enhance the local effect or to increase the penetration through the stratum corneum and viable epidermis for a systemic effect. For these purposes, colloidal particulate carriers such as liposomes or niosomes have been investigated by several groups (Memisoglu et al. Citation1997; Waranuch, Ramachandran, and Weiner Citation1998; Tabbakhian et al. Citation2006). These carriers can act as drug reservoirs, and modification of their composition or surface can adjust the drug release rate and/or the affinity for the target site. Liposomes or niosomes can carry hydrophilic drugs by encapsulation or hydrophobic drugs by partitioning of these drugs into hydrophobic domains. Xantines, and caffeine in particular, were shown to be active in hyperproliferative skin diseases, especially when administered topically using vesicular carriers (Touitou et al. Citation1994). Liposomal caffeine also is present in several cosmetic formulations (Fadda et al. Citation1998). However, there are some important problems in the general applications of liposomes for drug delivery such as degradation by hydrolysis or oxidation, as well as sedimentation, aggregation, or fusion of liposomes during storage (Alssara et al. Citation2004).
One alternative involves formation of liposome-like vesicles from the hydrated mixture of cholesterol and nonionic surfactants such as alkyl ethers, alkyl esters, or alkyl amides nonionic surfactants (Manosroi et al. Citation2003). Niosomes may have some advantages over liposomes with respect to chemical stability, lower costs of the chemicals, and large amount of surfactant classes available for the design of this vesicular system (Palozza et al. Citation2006). Another advantage is the simple method for the routine and large-scale production of niosomes without the use of unacceptable solvents (Alssara et al. Citation2004).
The goal of the present study was to formulate multilamellar (MLV) niosomal formulations of caffeine to be applied topically and to evaluate the in vitro performance of these formulations. Stability studies were carried out to investigate the leaching of drug from niosomes during storage.
MATERIALS AND METHODS
Caffeine was obtained from Merck (Germany). The nonionic surfactants used as vesicle-forming materials were sorbitan monolaurate (span 20), sorbitan monopalmitate (span 40), sorbitan monostearate (span 60), and sorbitan mono-oleate (span 80) and were purchased from Sigma Chemical (St. Louis, MO, USA). Cholesterol (CHOL) and cetyltrimethyl ammonium bromide (CTAB) were bought from Fluka (Switzerland). All organic solvents and the other chemicals were of analytical grade and were obtained from Merck (Germany) .
Preparation of Nonionic Surfactant Vesicles
Niosomes were prepared by the lipid hydration method as described previously with some modifications (Pardakhty, Varshosaz, and Rouholamini Citation2007). The method briefly was as follows: 150 μ mol of surfactant/CHOL (7:3 molar ratio, m.r.) or surfactant/CHOL/CTAB (5:3:2 m.r.) mixtures were dissolved in 10 ml chloroform in a 100-ml round-bottomed flask. The organic solvent was then removed at 60°C, under reduced pressure, in a rotary evaporator (Buchi, Switzerland) to form a thin film on the wall of the flask. Residual chloroform was evaporated in a vacuum oven for 6–8 hr at room temperature. The film was then hydrated with 5 ml isotonic phosphate buffered saline (PBS) (pH 7.4) containing caffeine (20 mg/ml) with a gentle rotation in water bath at 60°C for 30 min. The resulting MLV nonionic surfactant vesicle dispersions were then left to cool slowly. The compositions of the prepared vesicles are listed in . To evaluate the formation of MLVs, the niosomal suspensions were observed by optical microscope (HFX-DX, Nikon, Japan) and some micrographs were prepared by a camera attached to the microscope in 10 × 40 and 10 × 100 magnifications.
TABLE 1 Composition of caffeine loaded niosomes, mean volume diameter of vesicles, and caffeine encapsulation efficiency at different time intervals after preparation and storage at 4°C.
Vesicle Size Measurement
The size of niosomes was measured by static laser light diffraction method in a Malvern particle analyzer (Malvern Instruments, MasterSizer X-100, UK) at 72 hr after preparation. Measurement was carried out using a 100 mm focal length lens, which was capable of measuring vesicles in 0.5–180 μ m size range. The source of light was a low power helium-neon laser. The fundamental size distribution derived by this technique was volume based and the size distribution was expressed in terms of the volumes of equivalent spheres (dv). Size distribution parameters and derived diameters were calculated from the fundamental size distribution by using Sizer-X software.
Caffeine Encapsulation Efficiency
To separate the nonentrapped drug, the vesicle suspensions were centrifuged (E&K Scientific, Spectrafuge 16M, Canada) at 14,000 × g for 30 min at 25°C and washed twice with PBS (pH 7.4). The amount of caffeine in the supernatant and also in the pellets was analyzed by ultraviolet spectrophotometer (Shimadzu 2100, Japan), after disrupting the niosomes by isopropyl alcohol. The used λmax for caffeine assay was 273 nm in PBS. All experiments were run 3 times, and the calibration curve specifications were y = 0.049X ± 0.009 (r2 = 0.9992, n = 9). Drug encapsulation efficiency (EE) was calculated as follows:
EE% = 100 × mass of incorporated drug/ mass used for vesicle preparation
Caffeine Release
Caffeine release from the various formulations was evaluated using all glass Franz-type diffusion cells with an active surface area of 2.37 cm2 and a receptor phase volume of 37 ml (Ashke-shisheh Co., Iran). Prior to the diffusion experiments the acetate cellulose dialysis membrane (Visking tubing, cut-off 12000) was soaked in PBS (pH 7.4) for 12 hr. The membrane was then mounted between the cell's donor and the receptor compartments. Temperature was maintained at 32 ± 1°C by a circulating water bath. The receptor compartment was filled with PBS and the donor compartment with 1 ml vesicular dispersion of caffeine. The medium in the receptor compartment was magnetically stirred (magnetic stirrer Ikamag® RO 5 power, IKA, Germany) at a rate of 50 rpm. A caffeine PBS solution was used as control and empty niosomes as blanks. Samples of the receiving solution were withdrawn at fixed time intervals and replaced with an equal volume of PBS for up to 6 hr for analysis of the substance. Caffeine concentration in the receptor medium was quantified spectrophotometrically.
Physical Stability of Vesicles at Different Temperatures
Aggregation or fusion of the vesicles as a function of storage duration was determined as the changes in vesicle diameter by laser light scattering method. The vesicles were stored in glass vials in refrigerator (4°C) for 3 months. The changes in morphology of MLVs and also the constituent separation were assessed by the optical microscope. The retention of entrapped caffeine also was measured 72 hr and/or 3 months after preparation of the formulations. No special precautions were taken to improve the stability of vesicles.
Statistics and Data Analysis
The release data were fitted to various models using linear regression analysis. These models are zero-order (Qt = Q0 + K0t), first-order (ln Qt = ln Q0 + K1t), Higuchi (Qt = KHt1/2), Hixson-Crowell (Q01/3 − Qt1/3 = KSt), Korsmeyer-Peppas (Qt/Q∞ = Ktn) (Varshosaz,Tavakoli, and Kheirolahi Citation2006), and Baker-Lonsdale (3/2[1 − (1− Q t)2/3] − Qt = KBt)) (Baker and Lonsdale Citation1974).
All data are presented as the arithmetic mean values ± standard deviation (mean ± SD). Significant differences were calculated by analysis of variance (ANOVA) by SPSS 11.5 version and differences at p < 0.05 were considered as significant.
RESULTS AND DISCUSSION
Vesicle Characterization
The bilayer vesicles were prepared with the mixture of the amphiphilic substances (sorbitan esters) and cholesterol at different molar ratios. CTAB was used as a positively charged inducing agent. All used surfactants formed niosome suspensions in the presence of cholesterol. The micrographs in demonstrate the formation of vesicular structures from some sorbitan esters by classic film hydration method.
FIGURE 1 Optical micrographs (× 1000 magnification) of caffeine-containing niosomes prepared by film hydration method. Niosomes were composed of (a) Span 20/CHOL/CTAB, (b) Span 60/CHOL, and (c) Span 40/CHOL. Scale bar = 10 μ m.
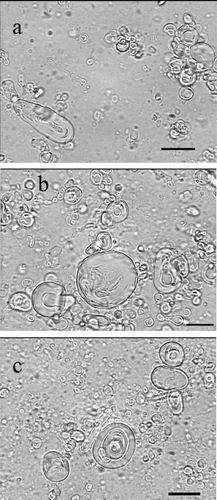
Niosomes were uniform in size and almost spherical in shape. The particle sizes of different formulations are presented in . Span 20/CHOL niosomes showed bimodal distribution of particle size. The smallest size of 6.81 μ m was observed in the span 80/CHOL/CTAB vesicles. All niosome formulations demonstrated log-normal particle size distribution, ranging from 6.81 to 22.10 μ m (). The size distribution curve of span 60-containing niosomes is presented in as an example.
FIGURE 2 The vesicle size distribution of caffeine-containing niosomes prepared by film hydration method. Niosomes were composed of span 60/CHOL (7:3 m.r.) or span 60/CHOL/CTAB (5:3:2 m.r.).
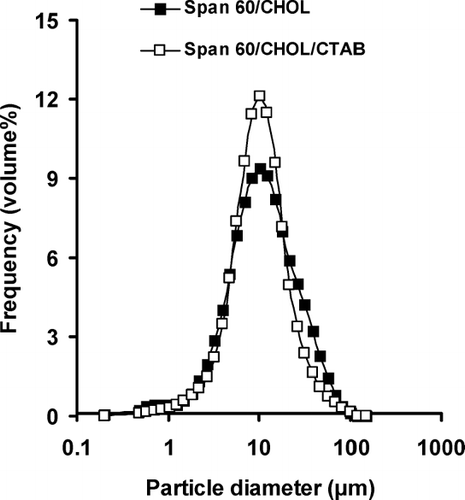
The mean size of neutral niosomes increased with progressive increase in the HLB value in different sorbitan ester surfactants (). The same result was reported about niosomes composed of sorbitan monoesters (span 20, 40, 60, and 80) or a sorbitan triester (span 85)/CHOL (Yoshioka, Strenberg, and Florence Citation1994). With the exception of span 20/CHOL/CTAB niosomes, in the other positive vesicular formulations, the mean volume diameter of niosomes also showed a regular increase with increasing HLB from span 80 (HLB 4.3) to span 40 (HLB 6.7) (). These results might be anticipated since surface-free energy decreases with increasing hydrophobicity (Yoshioka et al. Citation1994).
FIGURE 3 The effect of surfactants HLB values on the mean volume diameter of neutral and positive niosomes.
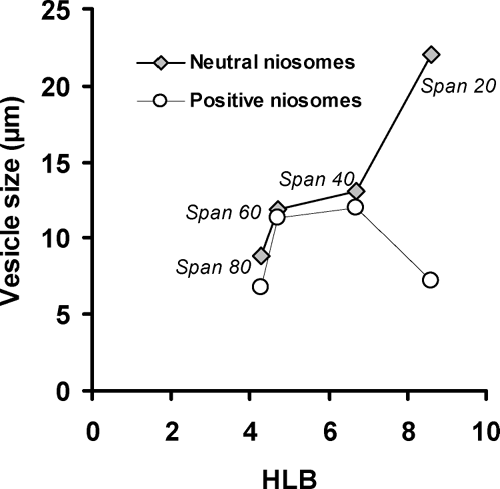
In all formulations, the incorporation of positively charged surfactant, CTAB, led to a decrease of vesicle size in comparison to neutral niosomes (). This effect was statistically significant in span 20-, span 40-, and span 80-containing vesicles (p < 0.05). We recently reported similar results about decreasing the average volume diameter of polyoxyethylene alkyl ether niosomes-entrapping insulin, following the insertion of negatively charged dicetylphosphate (DCP) (Pardakhty et al. Citation2007). A disproportionate distribution of CTAB in the bilayers may increase the curvature of the bilayer through electrostatic repulsion between the ionized head group, thus increasing the hydrophilic surface area. This effect will result in smaller vesicles (Israelachvili Citation1985).
Caffeine Encapsulation Efficiency
The percentage of caffeine entrapped in all niosome formulations varied between 30.44 and 81.75% (). Caffeine is sparingly soluble in water (2.13 g/100 ml at 25°C) and in organic solvents as indicated also by its low octanol/water partition coefficient (log Pow = −0.07). Thus it should be abble to enter into a vesicular structure both in the lipophilic bilayer and in the hydrophilic core (Fadda et al. Citation1998).The pKa of caffeine is equal to 14, and in PBS (pH 7.4), it will be as a positive ion. Therefore, the caffeine encapsulation efficiencies (EE) were reduced following the inclusion of CTAB in bilayers in comparison to neutral vesicles. Span 60/CHOL niosomes had the highest amount of entrapped drug (). This may be related to a high glass transition temperature of this amphiphile and the gel state nature of its bilayers which results in low permeability of vesicles. However, the high EE of caffeine in liquid state span 20/CHOL niosomes might be due to large vesicular size of this formulation (). Caffeine was successfully entrapped within the lipid bilayers of the present study vesicles with high efficiency in comparison to liposomes with 16% entrapment efficiency of drug (Touitou et al. Citation1994).
Release of Caffeine
The release characteristics of topical products can be assessed for quality assurance by using membranes other than skin. The usual types of membranes employed are those with porous characteristics, e.g., cellulose acetate or homogeneous permeable polymers such as silicone (Dias et al. Citation1999). In the present study we used cellulose acetate for assessment of drug release from MLVs.
As indicates, an initial rapid release of the drug without detectable lag-time and an equilibrium state or a slower release phase are observed with all formulations. The rapid initial phase may be originated from permeation of free caffeine and desorption of drug from the surface of niosomes and the slower phase related primarily to the diffusion of caffeine through the bilayers. Such effect also has been observed in the release of human insulin from niosomal suspensions (Pardakhty et al. 2006). In comparison to free caffeine solution, MLV structures always delayed caffeine release from the Franz's cell donor compartment (). However, incomplete release and diminishing drug flux was observed both in free solution or niosomal formulations.
FIGURE 4 Permeation profiles of caffeine through cellulose acetate after application of drug-containing neutral or positively charged niosomal formulations and free drug solution using Franz diffusion cells. Each point represents the mean ± SD (n = 3).
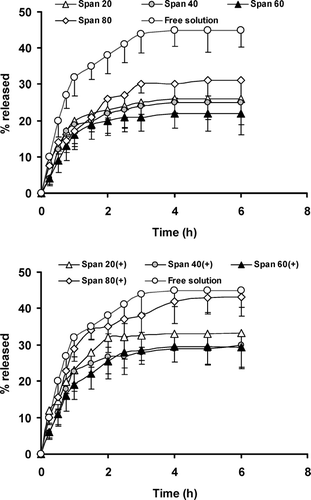
Decreasing permeation with proceeding incubation time is a sign for violating the sink conditions (Schreiber et al. Citation2005) and reducing the driving force for diffusion because of the depletion in concentration of diffusible caffeine. However, in our study the contacting fluid is water-based (PBS) and this solution cannot destroy the integrity of cellulose acetate membrane (Dias et al. Citation1999). The maintenance of membrane integrity during the release study beside the reduction of diffusion driving force can better explain the overall release percent less than 50% even in free solution (). The overall release amount of caffeine from different formulations presented in shows data analysis of release profiles according to different kinetic models.
Statistically there were not any significant differences between the overall release percent of caffeine from different niosomal formulations (, p > 0.05). However, positively charged niosomes delivered more caffeine due to electrostatic repulsion between bilayers and positively charged caffeine molecule and more nonentrapped drug in these formulations ().
With the exception of positively charged span 80 niosomes, drug release profile better fits with a Hixson-Crowell model and Peppas equation indicated the Fickian diffusion (). This finding suggests two mechanisms (i.e., erosion and diffusion) are involved in the release of caffeine from neutral and positive niosomes. In span 80/CHOL/CTAB formulation, the dominant mechanism is diffusion of caffeine through positively charged and liquid states bilayers ().
TABLE 2 Diffusion exponent (n) of Peppas model, calculated release rate (K) constants, and regression coefficient (r2, in brackets) of caffeine release data from studied niosomes according to different kinetic models
Physical Stability of Niosomes
exhibits the change in particle size and the level of entrapped caffeine as two major indicators for niosome stability during storage at 4°C for 3 months. Furthermore, precipitation of the membrane components was evaluated by microscopic observation. Lawrence et al. (Citation1996) suggested that size increment was due to fusion of the membranes and growth rather than vesicle aggregation. This effect can be avoided by electrostatic repulsion among vesicles or steric stabilization following surface modification of particles. In our study we used the first option by including the positive charged surfactant, i.e., CTAB. As expected, the positive niosomes showed less size change due to electrostatic repulsion ().
Niosomes prepared from solid-state surfactants i.e., span 40 and span 60, could better maintain the entrapped caffeine under storage conditions and were stable morphologically. However-in the neutral span 80 niosomes, the mean vesicle volume diameter increased from 8.89 ± 0.11 to 15.27 ± 0.63 μ m and EE of caffeine was changed from 38.49 ± 4.81 to 15.19 ± 5.89% (p < 0.05, ). These significant alterations in stability markers may result from the fluidity of span 80 bilayers leading to more leaky and unstable structure. Microscopic observation also revealed the formation of needle-like and plate crystals in this formulation. In another liquid state amphiphile i.e., span 20, the change of drug EE was statistically significant both in neutral and positive formulations (p < 0.05, ).
CONCLUSION
Caffeine was entrapped successfully within the lipid bilayers of the vesicles with high efficiency. The in vitro permeation of caffeine from niosomes of various compositions and presence of positive charge also have been studied and evaluated. The obtained data provide direct evidence that neutral vesicles, prepared with span 60 and cholesterol, entrap a higher caffeine amount, at pH 7.4, than positively charged vesicles. Niosomes may be a promising carrier for caffeine, especially due to their simple production and facile scale up.
REFERENCES
- Alssara I. A., Bosela A. A., Ahmed S. M., Mahrous G. M. Proniosomes as a drug carrier for transdermal delivery of ketorolac. Eur. J. Pharm. Biopharm. 2004; 20: 1–6
- Amato M., Isenschemid M., Huppi P. Percutaneous caffeine application in the treatment of neonatal apnoea. Eur. J. Pediatr. 1991; 150: 592–594
- Baker R. W., Lonsdale H. S. Controlled release: mechanisms and rates. Controlled Release of Biologically Active Agents, A. C. Tanquary, R. E. Lacey. Plenum Press, New York 1974; 15–71
- Dias M., Farinha A., Faustino E., Hadgraft J., Pais J., Toscano C. Topical delivery of caffeine from some commercial formulations. Int. J. Pharm. 1999; 182: 41–47
- Essig D., Costill D. L., Van Handel P. J. Effects of caffeine ingestion on muscle glycogen and lipid during leg ergometer cycling. Int. J. Sports Med. 1980; 1: 86–90
- Fadda A. M., Baroli B. M., Maccioni A. M., Sinico C., Valenti D., Alhaique F. Phospholipid-detergent systems: effects of polysorbates on the release of liposomal caffeine. Il Farmaco 1998; 53: 650–654
- Israelachvili J. N. Intermolecular and Surface Forces, , et al. Academic Press, Sydney 1985
- Lawrence M. J., Chauhan S., Lawrence S. M., Barlow D. J. The formation, characterization and stability of nonionic surfactant vesicles. STP Pharma. Sci. 1996; 6: 49–60
- Lu Y. P., Lou Y. R., Peng Q. Y., Xie J. G., Conney A. H. Stimulatory effect of topical application of caffeine on UVB-induced apoptosis in the epidermis of p53 and Bax knockout mice. Cancer Res. 2004; 64: 5020–5027
- Lu Y. P., Lou Y. R., Xie J. G., Peng Q. Y., Liao J., Yang C. S., Huang M. T. Topical applications of caffeine or (−)-epicatechin gallate (EGCG) inhibit carcinogenesis and selectively increase apoptosis in UVB-induced skin tumors in mice. Proc. Natl. Acad. Sci. USA 2002; 99: 12455–12460
- Manosroi A., Wongtrakul P., Manosroi J., Saki H., Sugawara F., Yuasa M., Abe M. Characterization of vesicles prepared with various nonionic surfactants mixed with cholesterol. Colloid. Surfaces B: Biointerf. 2003; 30: 129–138
- Memisoglu E., Öner F., Ayhan A., Basaran I., Hincal A. A. In vivo evaluation of rhGM-CSF wound-healing efficacy in topical vehicles. Pharm. Dev. Tech. 1997; 2: 171–180
- Palozza P., Muzzalupo R., Trombino S., Valdannini A., Picci N. Solubilization and stabilization of β -carotene in niosomes: delivery to cultured cells. Chem. Phys. Lipids 2006; 139: 32–42
- Pardakhty A., Varshosaz J., Rouholamini A. In vitro study of polyoxyethylene alkyl ether niosomes for delivery of insulin. Int. J. Pharm. 2007; 328: 130–141
- Sawynok J., Yaksh T. L. Caffeine as an analgesic adjuvant: a review of pharmacology and mechanisms of action. Pharmacol. Rev. 1993; 45: 43–85
- Schreiber S., Mahmoud A., Vuia A., Rubbelke M. K., Schmidt E., Schaller M., Kandarova H., et al. Reconstructed epidermis versus human and animal skin in skin absorption studies. Toxicol. In Vitro 2005; 19: 813–822
- Shiraki K., Andoh T., Imakita M., Kurokawa M., Kuraishi Y., Niimura M., Kageyama S. Caffeine inhibits paresthesia induced by herpes simplex virus through action on primary sensory neurons in rats. Neurosci. Res. 1998; 31: 235–240
- Tabbakhian M., Tavakoli N., Jaafari M. R., Daneshamouz S. Enhancement of follicular delivery of finasteride by liposomes and niosomes: 1. In vitro permeation and in vivo deposition studies using hamster flank and ear models. Int. J. Pharm. 2006; 323: 1–10
- Touitou E., Levy-Schaffer F., Dayan N., Alhaique F., Riccieri F. M. Modulation of caffeine skin delivery by carrier design: liposomes versus permeation enhancers. Int. J. Pharm. 1994; 103: 131–136
- Varshosaz J., Tavakoli N., Kheirolahi F. Use of hydrophilic natural gums in formulation of sustained-release matrix tablets of tramadol hydrochloride. AAPS Pharm. Sci. Tech. 2006; 7(1), article 24
- Waranuch N., Ramachandran C., Weiner N. D. Controlled topical delivery of cyclosporin-A from nonionic liposomal formulation: mechanistic aspects. J. Liposome Res. 1998; 8: 225–238
- Yamamura J., Koyasu M., Sato H., Kurokawa M., Yoshida Y., Amahabu Y., Shiraki K. Topical treatment of cutaneous herpes simplex virus-1 infection in mice with a specially formulated caffeine gel (Cafon). J. Dermatol. Sci. 1996; 12: 50–55
- Yoshioka T., Strenberg B., Florence A. T. Preparation and properties of vesicles (niosomes) of sorbitan monoesters (Span 20, 40, 60, and 80) and a sorbitan triester (Span 85). Int. J. Pharm. 1994; 105: 1–6