Abstract
Antineoplastic methotrexate has been loaded through different soaking procedures on silica-based mesoporous materials and, successively, released mimicking an oral administration. The materials were prepared using a self-assembly mechanism in the presence of cationic surfactants with alkyl chain of 16, 12, and 10 carbon atoms in the synthesis mixture to obtain different pore diameter in the porous structure. Mesoporous materials were prepared as pure silica sample and in the presence of Al(OH)3 in the synthesis mixture. Only alumina-silica samples were able to load methotrexate. The amounts of drug loaded and the in vitro release kinetics are a function of the pore size of the materials.
The successful synthesis of ordered mesoporous silica materials (OMS) opened a new investigation area in the field of inorganic molecular sieves. This discovery revealed exciting possibilities for OMS that have significantly larger pores than zeolites and thus allow expansion of the potential applications of the host-guest systems, from catalysis to controlled drug release. The invention of mesoporous molecular sieves by the researchers at the Mobil Research and Development Corporation (Beck et al. Citation1992), enabled escape from the “1 nm prison” imposed by zeolite-based microporous molecular sieves. In contrast to most of the porous materials, that show disordered pores with a broad size distribution and irregular shapes, the OMS materials exhibit exciting structural features like large specific surface area (ca. 1000 m2/g) and arrangements of ordered channel with a different geometry and a narrow size distribution. These mesoporous templated materials are synthesized by self-assembly of silica and surfactant micelles used as structure directing agents. For instance, the synthesis of MCM-41 type materials are performed under hydrothermal conditions in presence of cationic surfactants, such as dodecyl-hexadecyl-trimethylammoniumn bromide.
The structure of these ordered mesoporous materials results from a cooperative organization process between the negatively charged silicate species interacting with positively charged micelles made of quaternary ammonium surfactants. The porosity of the material is available after surfactant removal via calcination or solvent extraction. Indeed, their inner structure consists of unidirectional channel-like pores that form an hexagonal pattern. This pore architecture makes them suitable for hosting and further delivering of a variety of molecules of pharmaceutical interest. Indeed, several articles have been published regarding this new property of mesoporous materials, and the possibility of gaining control over the releasing pattern of the guest drug.
In the first work investigating the possibility of exploiting mesoporous materials to design drug delivery systems (DDSs) (Vallet-Regi et al. Citation2001), MCM-41 matrixes were employed as a support for hosting and delivering ibuprofen molecules. In this work, the authors showed that the release profile in simulating body fluid (SBF) of ibuprofen is deeply affected by the soaking procedure and slightly depends on the pore size. MCM-41 materials were investigated as DDSs for several antiflogistic drugs (Cavallaro et al. Citation2004). In this work it turned out that, in many cases, pore size can strongly influence the effectiveness of the examined DDSs and in particular that the release profile obtained from MCM-41 matrixes loaded with diflunisal is of practical interest. Ibuprofen also was anchored to mesoporous channels of MCM-41 matrixes by exploiting epoxide ring opening of 3-glycidoxypropylsilane grafted on the surface of MCM-41 matrixes (Tourné-Peteilh et al. Citation2003). The ester function resulting from the interaction between 3-glycidoxypropylsilane and the carboxylic group of ibuprofen provides a specific drug-release mechanism induced by the cleavage of the ester bond by esterases in vivo. The effects of both using different solvents for soaking MCM-41 materials and performing successive impregnations on the amount of loaded drugs were studied (Charnay, Begu, and Devoisselle Citation2004).
In particular, double soaking can result in a significant improvement of the amount of ibuprofen loaded into MCM-41. Interactions between ibuprofen and amino-silica matrixes were investigated through 13C and 1H solid state NMR spectroscopy (Babonneau et al. Citation2004). It was shown that the presence of amino groups reduces the mobility of ibuprofen molecules, suggesting the existence of interactions between the amino groups and the carboxylic groups. An alternative methodology synthesis was proposed by Balkus and Coutinho (Citation2003). The invention provides mesoporous materials with a new composition in which the templating molecule used in the synthesis procedure is vitamin E and molecules of the same family. Recently, a receptor specific ligand folic acid, in principle able to be recognized from specific tissues, has been covalently coupled on the external function of as-synthesized mesoporous silica as target function (Pasqua et al. 2006).
The present work reports the preparation of mesoporous materials and their ability to entrap and then release methotrexate (MTX) by reproducing “in vitro” oral administration. Methotrexate is a well-known antineoplastic drug used also in leukemia disease (Chessells et al. Citation1990). It is an excellent disease modifying drug for a whole host of rheumatic diseases, especially rheumatoid arthritis and psoriatic arthritis (Abu-Shakra et. al. Citation1995).
Several approaches are used for its administration to avoid the toxic side effects related to gastric release: pH-sensitive polymers (Narayani and Rao Citation1995), time-dependent formulations, bacterial degradable coatings, biodegradable polymer matrices and hydrogels (Iemma et. al. Citation2006; Qiu and Park Citation2001; Gil and Hudson Citation2004; Gupta, Vermani and Garg Citation2002). In this article we reported a comparative study of MTX release in MCM-41. The influence of several parameters is evaluated on the release profile of the hosted molecule: the nature of the host-guest chemical interaction, the pore size, and the chemical composition of the matrix. Considerable differences in the release characteristics have been observed. This opens the possibility of the development of a drug delivery system able to modulate drug release.
MATERIALS AND METHODS
Synthesis of MCM-41 and Al-MCM-41 Samples
MCM-41 silica-based materials were synthesized using the following procedure: as an example 1.35 g of NaOH (Carlo Erba, purum) and 10.2 g of dodecyltrimethilammonium bromide [C12H25N(CH3)3Br] (Sigma-Aldrich, 99% purum) were added to 120 g of demineralized and distilled water under continuous magnetic stirring. After complete dissolution 0.52 g of Al(OH)3 (Pfaltz & Bauer) were added. A milky solution was formed. Finally, 10.0 g of silica fume (Sigma-Aldrich) was added. The molar composition of the starting mixture was SiO2-0.04Al(OH)3-0.2NaOH-0.2C12H25N(CH3)3Br-40H2O. The obtained gel was put in a Morey-type Teflon lined autoclave at the temperature of 150°C for 24 hr. After this time the solid product obtained was separated by the liquid phase through filtration, then washed with distilled water, and dried at 80°C overnight. The activated MCM-41 sample was obtained by calcination in a air flow at 550°C with a heating rate of 1°C/min for 9 hr.
Pure silica and aluminium containing samples with cetyltrimethylammonium bromide surfactant [C16H33N(CH3)3 Br], named MCM-41(16) and Al-MCM-41(16), or dodecyltrimethylammonium bromide [C12H25N(CH3)3Br] surfactant, MCM-41(12) and Al-MCM-41(12), were prepared. An alternative synthesis procedure was used for preparation of Al-MCM-41(10) samples. The source of silica was sodium silicate and C10H21N(CH3)3Br surfactant was used. The reaction mixture had the following molar composition: SiO2-0.77NaOH-0.5surfactant-0.7H2SO4-52.8H2O. The obtained gel was poured into a polypropylene vessel and heated at 100°C for 30 hr. Finally, it was filtered, washed with water, and dried at 25.0 ± 0.5°C overnight. The surfactant was removed through thermal activation. This latter sample was named Al-MCM-41(10).
Characterization of MCM-41 and Al-MCM-41 Samples
All the samples were characterized by X-ray diffraction on powder (XRD), thermogravimetrical analysis (TG), energy dispersive spectroscopy (EDS) X-ray microanalysis, and nitrogen adsorption-desorption isotherms. The XRD spectra were acquired on a Philips PW1710 diffractometer (CuKα radiation) between 1 and 15 2 theta angle with a rate scan of 0.005 2theta/sec. The TG analyses were carried out with between 20 and 850°C with a heating rate of 10°C/min in a air flow of 5 cc/min using a Netzsch STA409 apparatus. EDS chemical analyses were performed by using a scanning electron microscope Jeol SM-T 330A equipped with a detector INCA Energy 300. N2 adsorption-desorption isotherms at 77 K were carried out using a Micromeritics ASAP 2010 porosimeter. Pore diameter was calculated using a method previously reported in literature (Galarneau et al. Citation1999) based on data obtained by XRD spectra, TG, and N2 adsorption-desorption analyses. In characterization of different MCM-41 obtained calcined samples is reported.
TABLE 1 Chemical and porous properties of MCM-41 and Al-MCM-41 calcined samples
Drug Loading by Soaking Procedure and In Vitro Drug Release
Soaking of mesoporous samples was performed using dimethylsulphoxide (DMSO) or phosphate buffer solution as solvents. A single or double soaking procedure was used. As an example, 250 mg of Al-MCM-41(16) powder were immersed in a solution made of 2 ml of solvent DMSO and 40 mg of methotrexate and soaked for 24 hr at 25.0 ± 0.5°C. After this period, the bead was dried under vacuum overnight. The impregnated product was separated from the solution by filtration and washed with 2 ml of DMSO. The amount of drug soaked was determined releasing the drug from 25 mg of the impregnated matrix dispersed in 10 ml of a 0.1 M NaOH solution.
Alternatively, a double soaking procedure was performed. As an example, 40 mg of methotrexate were dispersed in 100 ml of sodium phosphate buffer solution (25 ml of 0.1 M HCl, 1.2 ml 1 M NaOH, and 4 ml 0.6 M Na3PO4.10H2O) at pH 6.8 and kept under mild magnetic stirring for 24 hr at 25.0 ± 0.5°C to allow a complete dissolution of the drug. Then 250 mg of Al-MCM-41(16) were added to this solution and kept at 25.0 ± 0.5°C for 24 hr. Successively, the powder was separated from the solution by filtration and the samples were washed with 5 ml of DMSO to ensure the removal of methotrexate adsorbed on the external surface. Finally, the described procedure was repeated on the same soaked sample using a fresh buffer solution. Release studies were carried out according to the method reported in USP XXII (United States Pharmacopoeia, drug-release test). First 25 mg of the impregnated mesoporous sample were immersed in 10 ml of HCl 0.1 M solution (pH 1.0, simulating gastric fluid) and maintained at 37.0 ± 0.5°C in a water bath under magnetic stirring (∼ 50 rpm). Second, sodium phosphate buffer solution was added to raise pH to 6.8 (simulating intestinal fluid). At prefixed time samples were filtered and the obtained solution was recovered.
Evaluation of Drug Loading and Drug Release
Methotrexate concentrations in all the solutions were estimated by UV-VIS analysis. The UV-VIS spectrophotometer used was a Perkin-Elmer Lambda 3. The concentration of drug was monitored at 302 nm in 0.1 M NaOH solution or sodium phosphate buffer solution and 307 nm in 0.1 M HCl.
RESULTS AND DISCUSSION
X-ray powder diffraction patterns (not reported) of all MCM-41 samples show a very intense peak at low angle corresponding to the (100) reflection and a broader reflection at higher angle assigned to (110) and (200) directions. This indicates a good long-range order for all the samples. reports the nitrogen adsorption isotherms for Al-MCM-41 samples.
FIG. 1 Nitrogen (▵) ads.-(○) des. isotherms for Al-MCM-41 (16) (a), Al-MCM-41(12) (b), Al-MCM-41(10) (c) samples.
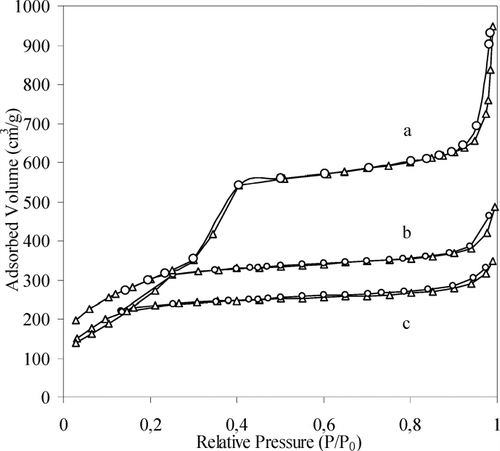
All the curves show type IV pattern typical of samples with a pore diameter higher than 20 Å. Silica-based mesoporous materials were synthesized using cationic surfactant with different apolar chain length. The size of micelles in aqueous solution determines the pore size in the silica-based materials. In characterization of different MCM-41 and Al-MCM-41 calcined samples obtained is reported. Si/Al ratio is quite similar in Al-MCM-41(16) and Al-MCM-41(12) materials. Al-MCM-41(10) sample has a higher Si/Al ratio and has been synthesized using a procedure involving sodium silicate (see Methods section). This source of silica contains relevant amount of aluminium as impurity and it is found in the amorphous structure. Unfortunately, the attempts to modulate the Si/Al ratio of the mesoporous materials self-assembled by C10 cationic surfactants using Al(OH)3 and silica fumed were unsuccessful. Samples synthesized with C16 surfactant show, as expected, a pore diameter (Dp) higher than samples prepared in the presence of C12 surfactant. MCM-41(12) sample has BET surface area (SBET) and pore volume (Vp) lower than the other materials prepared. The X-ray diffraction pattern and the nitrogen adsorption-desorption isotherms, typical of well-ordered mesoporous samples, have suggested their use in the drug-loading experiments. Mesoporous samples were prepared with and without aluminium to investigate interactions between inorganic substrates and organic molecule. Aluminium incorporated in tetrahedral coordination in the inorganic framework produces weak Bronsted acidity on the surface (Galarneau et al. Citation1999).
Three procedures were performed to load MCM-41 materials with MTX: a single soaking procedure using DMSO (named SSD), a sodium phosphate buffer solution (named SSB), and a double soaking procedure using only sodium buffer solution (named DSB) (see Methods). The amounts of drug adsorbed are reported in .
TABLE 2 Amount of adsorbed methotrexate through the different soaking procedures
Drug-loading experiments on the pure silica materials MCM-41(16) and MCM-41(12) show very low amounts of MTX entrapped. For this reason the soaking procedure with sodium phosphate solution were not carried out on these samples. One can see that the best loading capacity (SSB and SSD procedures) is exhibited by Al-MCM-41(12) material. The Al-MCM-41(16) material is characterized by large pore size and the diffusion rate of the solution into its cavities is faster than in Al-MCM-41(12). Consequently, Al-MCM-41(16) material entrapped lower quantities of MTX. The molecular size of MTX, in fact, is ∼ 23 × 7 Å, and this value is more compatible with materials having a range of pore sizes 25–30 Å for Al-MCM-41(12) and Al-MCM-41(10), with respect to 39 Å typical of Al-MCM-41(16).
When DSB loading procedure was used the amount of impregnated MTX is very high. This impregnation enables an almost complete pore filling and results in a relevant improvement of the amount of drug molecule encapsulated, as already reported in the literature (Charnay et al. Citation2004). In the X-ray diffraction pattern of Al-MCM-41(12) loaded with DSB procedure is reported. This pattern shows characteristic peaks of MTX in crystalline form at of 9.24, 11.50, 12.88, 19.46, and 26.96 2 theta. Al-MCM-41(16) and Al-MCM-41(10) show similar patterns. It is worth noting that the presence of crystalline MTX is not desirable since the crystalline form has low solubility and low biocompatibility. We did not report the double loading procedure in DMSO because the amount of incorporated MTX is very low. DMSO, in fact, is a too good solvent for MTX and does not encourage its adsorption.
FIG. 2 X-ray diffraction pattern of sample Al-MCM-41(12) after double soaking with sodium buffer procedure.
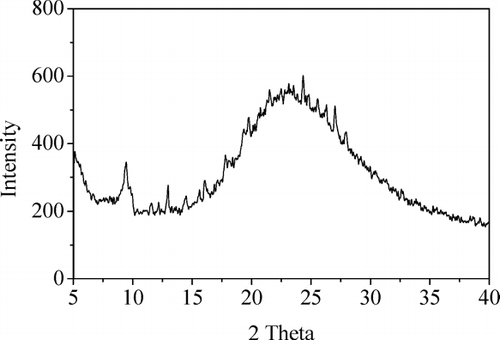
To determine the ability of these materials to release MTX, we carried out in vitro delivery studies at 37 ± 0.5 °C at pH 1 (simulated gastric fluid) for 2 hr and then at pH 6.8 (simulated intestinal fluid) using the pH change method. We supposed, in analogy with loading studies, that Al-MCM-41(10) and Al-MCM-41(12) materials have a better ability to control the drug release in physiological medium compared with the Al-MCM-41 (16) material. The release profiles of MTX loaded using SSD method confirmed this hypothesis. The experimental data (), indeed, revealed a better controlled release of drug from Al-MCM-41(10) and Al-MCM-41(12) than that obtained from Al-MCM-41(16) material. Especially in the first 2 hr Al-MCM-41(10) and Al-MCM-41(12) released, respectively, 37% and 42% of encapsulated MTX in respect to 54% for Al-MCM-41(16). This observation supports a model of retention mechanism that assumes that the delivery rate decreases strongly depending on the decreased pore sizes and that the diffusion of the physiological medium is affected by the effective pore size and by the interaction MTX/silica-aluminium matrix. At low pH (1.0) the MTX is undissociated form and the considerably different behavior between the materials depends on just two factors: the interactions MTX–silica/aluminium matrices and different diffusion rates of the buffer in the different pore size [it is faster in Al-MCM-41(16) cavities than Al-MCM-41(12) cavities and the last one is faster than Al-MCM-41(10) cavities]. At higher pH (6.8) the dissociated MTX form prevails and this factor promotes a significant increase in the amount of released drug.
Thus, the release contributions, depending on different diffusion rate of the buffer, are not appreciable in all release profiles. Instead, the delivery studies for the materials loaded using DSB procedure showed, in the first hours, an opposite behavior (): Al-MCM-41(16) materials have better performance than Al-MCM-41(12) and Al-MCM-41(10). These experimental data can be explained by assuming that Al-MCM-41(16) materials, having the largest pore size, can entrap larger amounts of drug in their cavities, compared with Al-MCM-41(12) and especially Al-MCM-41(10). Thus, for Al-MCM-41(12) and in a larger extent for Al-MCM-41(10) materials, the greatest amount of crystalline MTX is deposited on the surface. Consequently, in the first 2 hr, higher quantities of MTX were delivered by Al-MCM-41(10) and Al-MCM-41(12) materials.
CONCLUSION
We have introduce-methotrexate in the MCM-41 materials in powder form and the particles offer a sustained release. This good control of release depends principally on two factors: the presence of aluminium in the mesoporous materials and the pore size. The silica-aluminium blend offered better interactions with the drug in respect to only silica materials. Furthermore pore size influenced considerably the different diffusion rate of the solution in the cavities since it is slow in the cavities and faster on the surface of synthesized materials. Consequently, the materials with too large pore size [Al-MCM-41(16)] did not exhibit good sustained release. Finally, Al-MCM-41(12) showed a potential for the controlled delivery of drugs to prepare new kind of DDS. Under the experimental conditions used in this work, double soaking procedure (DSB) induced the partial crystallization of MTX and does not seem an efficient method for the loading of drug molecules in mesoporous materials.
This work was financially supported by MIUR (Programma di ricerca di rilevante interesse nazionale 2005) and the University funds.
REFERENCES
- Abu-Shakra M., Gladman D. D., Thorne J. C., Long J., Gough J., Frewell V. T. Long-term methotrexate therapy in psoriatic-arthritis-clinical and radiological. J. Rheumatol. 1995; 22(2)241
- Babonneau F., Yeung L., Steunou N., Gervais C., Ramila A., Vallet-Regi M. Solid state NMR characterisation of encapsulated molecules in mesoporous silica. J. Sol-Gel Sci. Tech. 2004; 31: 219
- Balkus J. R., Kenneth J., Coutinho Decio H. Mesoporous compositions and method of preparation. U.S. Patent 6630170, 2003
- Beck J. S., Chu C. T.-W., Johnson I. D., Kresge C. T., Leonowicz M. E., Roth W. J., Vartuli J. C. Synthesis of mesoporous crystalline material. U.S. Patent 5 108 725, 1992, (Mobil Oil, invs)
- Cavallaro G., Pierro P., Palumbo Testa F., Pasqua L., Aiello R. Drug delivery devices based on mesoporous silicate. Drug Del. 2004; 11: 41
- Charnay C., Begu S., Devoisselle J. M. Inclusion of ibuprofen in mesoporous templated silica: drug loading and release property. Eur. J. Pharm. Biopharm. 2004; 57: 533
- Chessells J. M., Cox T. C., Kendall B., Cavanagh N. P., Jannoun L., Richards S. Neurotoxicity in lymphoblastic-leukemia—comparison of oral and intramuscular methotrexate and 2 doses of radiation. Arch. Dis. in Childhood 1990; 65: 416
- Galarneau A., Desplantier D., Dutartre R., Di Renzo F. Micelle-templated silicates as a test bed for methods of mesopore size evaluation. Micropo. Mesopor. Mat. 1999; 27: 297
- Gil E. S., Hudson S. M. Stimuli-reponsive polymers and their bioconjugates. Prog. Polymer Sci. 2004; 29: 1173
- Gupta P., Vermani K., Garg S. Hydrogels: from controlled release to pH-responsive drug delivery. Drug Disc. Today 2002; 7: 569
- Iemma F., Spizzirri U. G., Puoci F., Muzzalupo R., Trombino S., Cassano R., Leta S., Picci N. pH-Sensitive hydrogels based on bovine serum albumin for oral drug delivery. Inter. J. Pharm. 2006; 312: 151
- Narayani R., Rao K. P. pH-responsive gelatin microspheres for oral delivery of anticancer drug methotrexate. J. Appl. Polymer Sci. 1995; 58: 1761
- Pasqua L., Testa F., Aiello R., Cundari S., Nagy J. B. Preparation of bifunctional ibrid mesoporous silica potentially useful for drug targeting. Micropor. Mesopor. Mat., 103: 166
- Qiu Y., Park K. Environment-sensitive hydrogels for drug delivery. Adv. Drug Del. Rev. 2001; 53: 321
- Tourne-Peteilh C., Brunel D., Begu S., Chice B., Fajula F. Synthesis and characterisation of ibuprofen-anchored MCM-41 silica and silica gel. New J. Chem. 2003; 27: 1415
- Vallet-Regi M., Rámila A., Del Real R. P., Pérez-Pariente J. A new property of MCM-41: drug delivery system. Chem. Mat. 2001; 13: 308