Abstract
Efficacy of α-TEA formulated in liposome or biodegradable poly(D, L-lactide-co-glycolide) (PLGA) nanoparticle was evaluated in a BALB/c clone 66cl-4 mammary cancer mouse model using oral delivery. α-TEA-loaded liposome or nanoparticle at 5 mg, but not 2.5 mg/day, significantly reduced tumor burden (p < 0.001). Both formulations at 5 mg significantly reduced visible lung metastases and lymph node and lung micrometastatic tumor foci. Surprisingly, nanoparticle control mice exhibited significantly reduced tumor burden, lymph node, and lung micrometastatic tumor foci. Both formulations at 5 mg significantly enhanced tumor apoptosis. In summary, liposomes and nanoparticles are effective means for administering the lipophilic anticancer drug α -TEA.
To develop a stable and clinically useful vitamin E-based chemotherapeutic agent, a nonhydrolyzable ether linked acetic acid analogue of RRR-α -tocopherol, namely, α -TEA, has been produced (Lawson et al. Citation2003). α -TEA has been shown to induce human breast, prostate, colon, lung, ovarian, cervical, and endometrial cancer cells to undergo apoptosis in vitro, but not normal human mammary epithelial cells and normal prostate epithelial cells (Anderson et al. Citation2004b). α -TEA formulated in liposomes and delivered by aerosol exhibits antitumor activity in preclinical xenograft models using A2780/cp70 human ovarian cancer cells and human MDA-MB-435 breast cancer cells (Anderson et al. Citation2004a; Zhang et al. Citation2004). Also, α -TEA has been shown to be a potent concentration- and time-dependent inducer of apoptosis of murine mammary 66cl-4-GFP tumor cells in vitro, and to significantly decrease primary tumor burden and lung metastasis of 66cl-4-GFP tumor cells transplanted into syngeneic Balb/c mice when formulated in liposome and administered by aerosol or gavage (Lawson et al. Citation2003, Citation2004).
Breast cancer is the most common cancer and the second leading cause of cancer deaths among women in the United States (Smigal et al. Citation2006). Thus, there is a great need for effective chemotherapy agents for breast cancer. Expectations are that α -TEA, a new, stable, nontoxic vitamin E analog with promising anticancer properties, will prove to be an effective anticancer agent for human breast cancer. Since α -TEA is hydrophobic, an appropriate drug delivery system is needed for better therapeutic efficacy.
Biomedical research has shown the great need to control, regulate, and target the release of drugs in the body to tissues or cells of interest (Lee Citation2006). A primary goal is to use less frequent drug administration to achieve a constant therapeutic level of drug in the systemic circulation or at the specific target organ site to reduce undesirable drug effects. Among the different strategies for drug delivery, colloidal carriers were found to be effective. The most popular and well-studied colloidal drug delivery systems are the liposome systems that permit high drug load and are relatively easy to handle. However, they have some fundamental problems, such as susceptibility to degradation by lysosomes and noncontrollable drug release rate (Hofheinz et al. Citation2005; Zamboni Citation2005). Studies in our lab have used α -TEA/liposome formulations delivered by aerosol and gavage (Anderson et al. Citation2004a; Lawson et al. Citation2003, Citation2004; Zhang et al. Citation2004). Recently, biodegradable polymeric nanoparticle systems have been developed to improve drug delivery. Nanoparticles, in general, can be used to improve oral bioavailability, to sustain drug/gene effect in target tissue, and to improve the stability of therapeutic agents against enzymatic or lysosomal degradation (Lee Citation2006; Panyam and Labhasetwar Citation2003a, Citation2003b).
Biodegradable polymers are natural or synthetic in origin and are degraded in vivo to produce biocompatible, toxicologically safe byproducts that are further eliminated via metabolic pathways (Jain Citation2000; Shive and Anderson Citation1997). Of these polymers, polylactides (PLA) and poly (D,L-lactide-co-glycolide) (PLGA) have been investigated the most. As reviewed by Jain (Citation2000), PLGA has been used to form an effective nanoparticle delivery system, has been approved by the Food and Drug Administration, and has a history of safe use in humans. A Good Manufacturing Practices grade material is available from commercial sources, making it an ideal candidate for use in novel formulations for the poorly water-soluble vitamin E analog.
In this study, we used DLPC (1,2-dilauroyl-sn-glycero-3-phosphocholine) as lipid for liposomes and PLGA as polymer for nanoparticles. we compared the ability of these two α -TEA formulations in two drug dosages (5 mg/day or 2.5 mg/day) to reduce BALB/c 66cl-4 tumor burden and metastasis to lung and lymph nodes when delivered by gavage.
MATERIALS AND METHODS
α − TEA (formula weight = 488.8) was prepared as described previously (Lawson et al. Citation2003); 1,2-dilauroyl-sn-glycero-3-phosphocholine (DLPC) was purchased from Avanti Polar-Lipids, (Alabaster, AL, USA); PLGA, (MW 143,900, copolymer ratio 50:50), Tween 80, and dichloromethane (HPLC grade) were purchased from Sigma Chemicals (St. Louis, MO, USA).
Preparation of Liposomal and Nanoparticle α -TEA
An α -TEA/DLPC ratio of 1:3 (w/w) has been determined previously to be optimal (Lawson et al. Citation2003). To prepare the α -TEA/DLPC combinations, the components were first brought to room temperature. The lipid DLPC, at a concentration of 120 mg/ml, was dissolved in tertiary-butanol (Fisher Scientific, Houston, TX, USA) and then sonicated to obtain a clear solution. α -TEA at 40 mg/ml was dissolved in tertiary-butanol and vortexed until all solids were dissolved. DLPC and α -TEA were combined in equal amounts (v:v) to achieve the desired ratio of 1:3 α -TEA/liposome, mixed by vortexing, frozen at -80°C for 1-2 hr, and lyophilized overnight to a dry powder prior to storing at −20°C until needed. Particle size range of α -TEA liposomes delivered orally was determined to be 4–10 μ m (Lawson et al. Citation2004).
α -TEA-loaded nanoparticles were prepared by an emulsion/solvent evaporation method based on previous work (Davda and Labhasetwar Citation2002; Feng et al. Citation2004; Sinha et al. Citation2002) and modified for α -TEA. The main variables that influence the microencapsulation process and the final product are, (a) the nature and solubility of the drug being encapsulated, (b) the polymer concentration, (c) the drug/polymer ratio, (d) the organic solvent used, (e) the concentration and nature of the emulsifier used, (f) the status of emulsifier in aqueous solution, (g) the temperature and stirring speed of the emulsification process, (h) the evaporation time, and (i) the viscosities and volume ratio of the dispersed and continuous phases (Jain Citation2000). These factors were all taken into consideration when modifying the published protocols. In brief, PLGA (200 mg) was first dissolved in a water immiscible, volatile organic solvent, dichloromethane (16 ml).
α -TEA at 20 mg was added to the polymer solution. A 0.4% solution of Tween 80 was prepared in 250 ml of distilled water maintained at 40°C and then filtered through a 0.22 μ m filter. The formed PLGA solution was subsequently added to the Tween 80 solution. The resulting emulsion was sonicated with an energy output of 50W in a pulse mode for 90 sec. The oil-in-water emulsion was stirred for 3 hr at room temperature on a magnetic stir plate to allow the evaporation of dichloromethane and formation of the nanoparticles.
The suspension was transferred into ultracentrifuge tubes and centrifuged at 12,000 rpm for 30 min at room temperature. The pellet was washed with distilled water three times to remove residual Tween 80. The supernatant and the washings were collected and used to determine the amount of drug that was not trapped in the nanoparticles. The suspension was collected and frozen at −80°C overnight and subsequently lyophilized for 1 day. The lyophilized nanoparticles were stored desiccated at −20°C. The nanoparticles were stable for at least 3 months.
Nanoparticle Size Distribution
To measure nanoparticle size distribution using dynamic laser light scattering, nanoparticles were suspended in distilled water and then subjected to particle size analysis using a Zeta Plus™ particle size analyzer (Brookhaven Instruments, NY, USA). The size and morphology of the nanoparticles were further analyzed by transmission electron microscopy. Briefly, 2 μ l of nanoparticle suspension was placed on a carbon film coated on a TEM copper grid. The particles were immediately frozen with liquid nitrogen, dried by vacuum and visualized.
Nanoparticle Drug Encapsulation Efficiency
The drug encapsulation efficiency (DEE) is calculated as DEE = [(total amount of drug–free amount of drug)/total amount of drug] × 100. The supernatant and washings generated during the production of nanoparticles were collected and used to determine the amount of α -TEA that was not trapped in the nanoparticles (free drug). Free α -TEA was extracted using 100% chloromethane. Reverse phase HPLC analyses were conducted as previously described (Tirmenstein et al. Citation1998). In this case, DEE = 99%. This indicates complete drug encapsulation can be achieved if the ratio of drug and polymer is appropriate (Lin et al. Citation2000).
66cl-4-GFP Murine Mammary Tumor Cell Line
66cl-4 cells were derived from a spontaneous mammary tumor in a BALB/cfC3H mouse and isolated as a 6-thioguanine-resistant clone (Dexter et al. Citation1978; Miller et al. Citation1983). These cells were stably transfected with green fluorescence protein as previously described (Lawson et al. Citation2003). 66cl-4-GFP cells are highly metastatic, 100% of animals develop micrometastatic lesions detectable with fluorescent microscopy in the lungs 26 days following subcutaneous injection of 2× 105 tumor cells into the inguinal area (Lawson et al. Citation2003).
BALB/c Mice
Female BALB/c mice at 6 weeks of age (about 25 g body weight) were purchased from Jackson Labs (Bar Harbor, ME, USA) and allowed to acclimate for 1 week. Mice were housed 5/cage and given water and standard lab chow (Harlan Teklad #2018 Global 18% Protein Rodent Diet; Madison, WI, USA) ad libitum at the Animal Resource Center at the University of Texas at Austin. Mice were maintained in an environment of 74 ± 2°F with 30%–70% humidity and a 12-hr alternating light-dark cycle. Guidelines for the humane treatment of animals were followed as approved by the University of Texas Institutional Animal Care and Use Committee.
Tumor Cell Inoculation
66cl-4-GFP cells were harvested by trypsinization, collected by centrifugation, and resuspended at a density of 2 × 105 cells/100 μ l in McCoy's media, containing no supplements. Mice were injected with 2 × 105 cells/100 μ l in the inguinal area at a point equal distance between the fourth and fifth nipples on the right side using a 25-gauge needle.
Fully 60 mice, 10/group, were placed in 6 groups [(liposome/gavage control, liposome α -TEA/gavage low dose (2.5 mg), liposome α -TEA/gavage high dose (5 mg), nanoparticle (NP) gavage control, NP α -TEA/gavage low dose (2.5 mg), and NP α -TEA/gavage high dose (5 mg)] such that the average tumor volume for all groups was closely matched. Each group had an average tumor volume/group of 1.94 mm3, 1.91 mm3, 1.84 mm3, 1.84 mm3, 1.88 mm3, and 1.84 mm3, respectively, at the start of treatments, that were begun 12 days following tumor cell inoculation. Tumors were measured using calipers every other day, and tumor volumes were calculated using the following formula: volume (mm3) = [width (mm2) × length (mm)]/2 (Clarke Citation1997). Body weights were determined weekly.
Gavage Delivery
For gavage delivery, lyophilized preparations of liposome α -TEA, nanoparticle α -TEA, liposome only and nanoparticle only, were brought to room temperature and reconstituted by adding 1 ml (low dose groups) or 2 ml (high dose and control groups) distilled water to achieve the final desired concentration of 25 mg/ml α -TEA. Treatments were vortexed vigorously immediately prior to administration by gavage. The total amount of α -TEA administered by gavage was 100 μ l/mouse per day for low dose groups and 200 μ l/mouse/day for high dose groups (final concentration, 2.5 or 5 mg α -TEA/mouse/day). High dose and control group treatments were given twice/day, approximately 4 hrs apart. All treatments were given 7 days/week, for a total of 20 days.
Lung and Lymph Node Metastasis
Macroscopic metastases in all 5 lung lobes were counted visually at time of euthanasia. Fluorescent microscopic lung lesions were counted as described previously, using a Nikon fluorescence microscope [TE-200; 3200 magnification (Lawson et al. Citation2003)]. Fluorescent microscopic lesions were scored by size into four size groupings: < 20 μ m, 20–50 μ m, 50-100 μ m, and > 100 μ m. On the basis of a typical 66cl-4-GFP tumor cell size of 10–20 μ m in diameter, the < 20 μ m grouping is thought to represent solitary cells; the 20–50 μ m grouping 2 to 5 cells; 50-100 μ m grouping 5 to 10 cells, and the > 100 μ m grouping microscopic lesions of greater than 10 cells.
TUNEL Assay for Detection of Apoptosis In Vivo
Deparaffinized sections (5 μ m) of tumor tissue were used to assess apoptosis using reagents supplied in the ApopTag In Situ Apoptosis Detection kit (Intergen, Purchase, NY, USA) according to the manufacturer's instructions. Nuclei that stained brown were scored as positive for apoptosis, and those that stained blue were scored as negative. At least 16 microscopic fields (× 400) were scored per tumor. Data are presented as the mean ± SE number of apoptotic cells counted in 6 separate tumors from each group.
Detection of Immune Cell Infiltration in Tumor Tissue
Deparaffinized tumor sections (5 μ m) were stained with antibodies to CD45R or CD3 to determine B or T-cell infiltration. Briefly, endogenous peroxidase activity was blocked with 3% H2O2 and nonspecific antibody binding was blocked with 10% normal rabbit serum. Tumor sections were incubated with either CD45R or CD3 antibody (rat anti mouse antibody; Serotec, Raleigh, NC, USA; 1:200 dilution) overnight at 4°C. Next, the tissue sections were incubated with biotinylated rabbit-antirat IgG (Vector Laboratories, Burlingame, CA, USA) at a 1:200 dilution for 30 min at room temperature, washed, and then incubated with avidin-biotin complex (ABC-HRP, Vector Laboratories) for 30 min at room temperature. Immunoreactivity was visualized via incubation with diaminobenzidine dihydrochloride. Slides were lightly counterstained with hematoxylin. Then 16 or more microscopic fields (400×) were scored for positive stained cells/tumor. Data were collected using 3 separate tumors from each group. In addition, H&E-stained tumor sections were used for the detection of cellular infiltration.
Statistical Analyses
Tumor growth was evaluated by transforming volumes using a logarithmic transform (base 10) and analyzed using a nested two-factor ANOVA with Tukey HSD, using SPSS (SPSS, Chicago, IL, USA). The differences in the number of macroscopic and microscopic metastatic lesions/group, T-and B-stained cells/group and TUNEL positive cells/group were analyzed by the two tailed Mann-Whitney rank test using SPSS. A level of p < 0.05 was regarded as statistically significant.
RESULTS
Nanoparticle Morphology, Size Determination, and Distribution
The mean size and distribution pattern of α -TEA loaded nanoparticles was determined by dynamic light scattering (DLS) (De and Robinson Citation2004). The mean size diameter of the vesicle was found to be approximately 272 nm, and polydispersity, which is a measure of the distribution of molecular weights in a given polymer sample, was equal to 0.313 (). The size distribution pattern as determined by measuring the intensity of the nanoparticles by DLS is shown in . The size and morphology of the nanoparticles were further checked by transmission electron microscopy (TEM) (). Using TEM, the nanoparticles were found to be of spherical shape with a mean diameter of 190 ± 65 nm. The nanoparticle mean diameter measured using TEM was much smaller than the mean diameter obtained with the DLS method. This discrepancy in size of nanoparticles is because the DLS method gives the hydrodynamic diameter rather than the actual diameter of nanoparticles.
FIG. 1 Nanoparticle size distribution. (A) and (B) Size distribution of nanoparticles (PLGA-α -TEA) determined by dynamic light scattering, using a neon laser of λ = 677.0 nm and measured at 90° degree. The effective diameter of nanoparticles = 272 nm, polydispersity = 0.313. Measurements were repeated three times, each time with similar patterns. (C) Transmission electron microscopy of α -TEA-loaded nanoparticles (PLGA: α -TEA = 10:1). Nanoparticles were visualized directly after freeze-drying. The mean diameter was 190 ± 65 nm. Bar represents 500 nm.
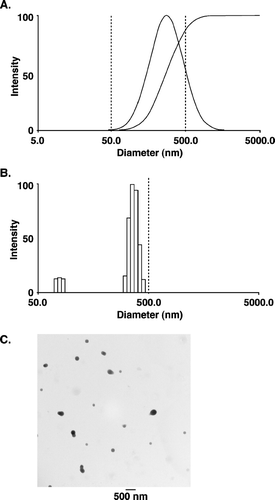
Liposomal α -TEA High Dose and All Nanoparticle Treatments Significantly Suppressed Tumor Growth
Mean tumor volumes in mice receiving liposomal and nanoparticle α -TEA at 5 mg/mouse/day were significantly lower than liposome or nanoparticle controls, respectively, over 20 days of treatment (p < 0.001; ). Mean tumor volume in mice receiving nanoparticle α -TEA at 2.5 mg/mouse/day was not significantly different from nanoparticle control, but it was significantly different from liposome control (p < 0.001; ). Liposomal α -TEA at 2.5 mg/mouse/day reduced tumor burden but not significantly from liposome control. Mean tumor volumes in mice receiving nanoparticle α -TEA at 5 mg/mouse/day, in comparison to all the other treatment groups, was significantly lower over the 20 days of treatment (p < 0.001 in comparison to liposomal α -TEA at 2.5 mg/ml; p < 0.002 in comparison to liposomal α -TEA at 5 mg/ml; and p < 0.017 in comparison to nanoparticle α -TEA at 2.5 mg/ml). Surprisingly, data show that the nanoparticle control, in comparison to liposome control, significantly reduced tumor burden to a degree comparable to liposomal α -TEA at 5 mg/mouse/day or nanoparticle α -TEA at 2.5 mg/mouse/day treatment groups ().
FIG. 2 Effects of liposome- or nanoparticle-formulated α -TEA delivered by gavage on tumor burden. 66cl-4-GFP cells at 2 × 105/mouse were injected into the inguinal area at a point equal distance between the fourth and fifth nipples. Then 12 days after tumor injection, mice were assigned to control and α -TEA treatment groups such that the mean tumor volume of each group was closely matched (average tumor volume/group = 1.88 mm3). Mice (10/group) were treated with liposomal control, nanoparticle control, liposomal α -TEA, or nanoparticle α -TEA at high dose (5 mg/mouse/day) or low dose (2.5 mg/mouse/day) for 20 days. Tumor volumes (mm3) are depicted as mean ± SE. (1) = significantly reduced in comparison to liposome control, (2) = significantly reduced in comparison to nanoparticle control, and (3) = significantly reduced in comparison to liposomal α -TEA at 2.5 mg/day.
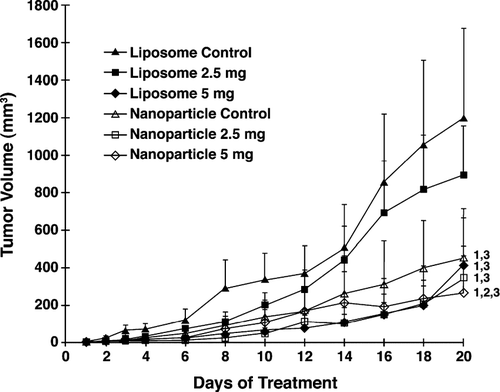
Reduced Visible Macroscopic Lung Metastases
At sacrifice, all 5 lung lobes from each mouse were examined for visible metastases. All mice in the liposome and nanoparticle control groups as well as nanoparticle α -TEA at 2.5 mg/mouse/day had visible lung tumor foci. But 4/10 mice in liposomal and nanoparticle high dose treatment groups did not have visible lung tumor foci; but this was not significantly different from controls. However, the average number of macroscopic lung tumor foci was significantly reduced in all treatment groups when compared with their respective control group(); namely, in comparison to liposome control: liposomal α -TEA at 2.5 mg/mouse/day, p < 0.011; liposomal α -TEA at 5 mg/mouse/day, p < 0.002; and in comparison to nanoparticle control: nanoparticle α -TEA at 2.5 mg/mouse/day, p < 0.028; nanoparticle α -TEA at 5 mg/mouse/day, p < 0.001.
TABLE 1 α -TEA formulated into liposome or nanoparticle reduced the average number of visible macroscopic lung metastases.
Reduced Lung Micrometastatic Tumor Foci
Green fluorescing microscopic lung lesions were classified into four size groups: < 20, 20-50, 50-100, and > 100 μ m. The mean total number of microscopic lung tumor foci in liposomal and nanoparticle treatment groups were significantly reduced in comparison to liposome control (p < 0.001 for all) or nanoparticle control (p < 0.001) for all except liposomal α -TEA at 2.5 mg/mouse/day, in which p < 0.044 (). The mean total number of microscopic lung tumor foci in nanoparticle α -TEA at 2.5 mg/mouse/day were significantly reduced in comparison to liposomal α -TEA at 2.5 mg/mouse/day (p < 0.003). This significant reduction appears to be attributable mainly to lesions in the < 20 μ m size grouping. Although nanoparticle α -TEA at 5 mg/mouse/day had a comparable total number of microscopic lung lesions as liposomal α -TEA at 5 mg/mouse/day, the microscopic lung tumor foci in the 20–50 μ m and 50–100 μ m size grouping were significantly lower (p < 0.024 for both size groupings). The mean total number of microscopic lung lesions in the nanoparticle control group itself was significantly reduced in comparison to the liposome control group (p < 0.005)().
FIG. 3 Effects of liposome- and nanoparticle-formulated α -TEA delivered by gavage on micrometastatic tumor foci in lung and lymph node. (A) Using a Nikon fluorescence microscope, the number of fluorescent microscopic tumor foci on the surface (top and bottom) of flattened left lung lobes was determined for control and treatment groups. Micrometastatic tumor foci were grouped into four size groupings (< 20 μ m, 20-50 μ m, 50–100 μ m, or > 100 μ m). (B) Using a Nikon fluorescence microscope, the number of fluorescent microscopic metastases in brachial and axillary lymph nodes was determined for control and treatment groups. For both (A) and (B), average number of total lesions was calculated. Data are depicted as mean ± SE of total number of microscopic metastases. (1) = significantly reduced in comparison to liposome control, (2) = significantly reduced in comparison to nanoparticle control, and (3) = significantly reduced in comparison to liposomal α -TEA at 2.5 mg/day.
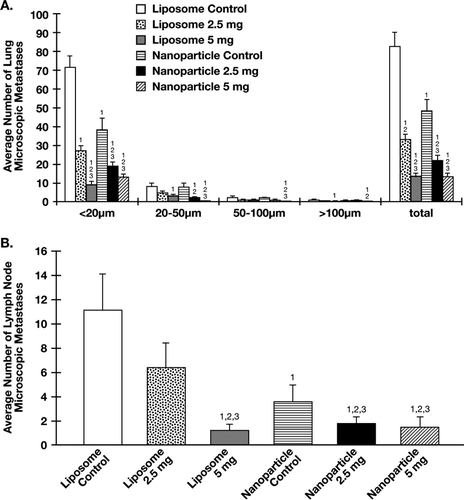
Reduced Microscopic Lymph Node Tumor Foci
Green fluorescent microscopic lymph node metastatic lesions, like the lung microscopic lesions, were classified into four different size groups: < 20, 20–50, 50–100, and > 100 μ m. Data are shown for the total number of microscopic tumor foci. The mean total number of microscopic lymph node metastases in liposomal high dose and both of the nanoparticle treatment groups were significantly reduced in comparison to liposome control (p < 0.001 for all) or nanoparticle control (liposomal α -TEA at 5 mg/mouse/day, p < 0.006; nanoparticle α -TEA at 2.5 mg/mouse/day, p < 0.048; and nanoparticle α -TEA at 5 mg/mouse/day, p < 0.005). The mean total number of microscopic lymph node metastases in nanoparticle α -TEA at 2.5mg/mouse/day was significantly reduced in comparison to liposomal α -TEA at 2.5 mg/mouse/day (p < 0.002). The mean total number of microscopic lymph node metastases in the nanoparticle control group itself was significantly reduced in comparison to liposome control group (p < 0.001)().
Tumor Cells Undergo Apoptosis
Apoptosis was evaluated using TUNEL staining of 5 μ m tumor sections from control and treatment groups. The mean number of TUNEL positive cells in the high dose liposomal and nanoparticle groups were significantly higher than their respective controls (liposomal α -TEA at 5 mg/mouse/day, p < 0.009; nanoparticle α -TEA at 5 mg/mouse/day, p < 0.003). TUNEL positive cells in the liposome and nanoparticle α -TEA low dose groups were not significantly different from their respective controls; however, the average number of TUNEL positive cells in the low dose nanoparticle group was significantly higher than the liposomal control p < 0.016 (). The average number of TUNEL positive cells in the nanoparticle and liposome control groups were not significantly different from each other.
FIG. 4 α -TEA formulated into liposome or nanoparticle and delivered by gavage-induced 66cl-4-GFP cells to undergo apoptosis. Fully 5 μ micron tumor tissue sections from control and treatment groups were examined for levels of apoptosis by TUNEL. Data are depicted as mean ± SE of TUNEL positive-stained cells/field. (1) = significantly enhanced in comparison to liposome control, (2) = significantly enhanced in comparison to nanoparticle control, and (3) = significantly enhanced in comparison to liposomal α -TEA at 2.5 mg/day.
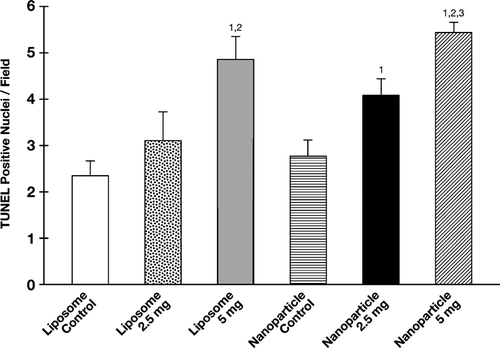
Cellular Infiltration in Tumor Tissue
Number of immune cells infiltrating tumors based on analyses of H&E stained tumor sections and immunohistochemical analyses of tumor sections stained specifically for B and T lymphocytes from all control and treatment groups were low, with no significant differences among control and treatment groups (data not shown). Of special note, there was no difference in number of intratumoral B and T lymphocytes in tumor sections from liposome and nanoparticle control groups.
Oral Deliver No Effect on Body Weights
Although the mean body weights of mice receiving nanoparticle formulations were slightly less than body weights of mice receiving liposomes, there were no significant differences in the mean body weights of mice among all the control and treatment groups (data not shown).
DISCUSSION
Previous studies in our lab showed that α -TEA, a novel acetic acid analog of vitamin E (RRR-α -tocopherol), when formulated in liposomes and delivered by either aerosol at 7.5 mg/mouse/day or gavage at 6.4 mg/mouse/day had marked antitumor efficacy and no evidence of toxicity in BALB-c mouse mammary tumor model (Lawson et al. Citation2003; Citation2004). To conserve the amount of drug we used and to make it clinically friendly for future administration practice, we chose gavage method in this study. Two levels of α -TEA, low (2.5 mg/mouse/day) and high (5 mg/mouse/day), were used for this comparison of liposome versus nanoparticle formulation effectiveness. The high dose α -TEA/liposome formulation was expected to produce a superior anticancer efficacy than the low dose α -TEA/liposome formulation. Effects of α -TEA formulated in nanoparticles were unknown, but based on the literature, expectations were that nanoparticle formulations would be as effective and perhaps more effective as an anticancer agent than α-TEA formulated in liposomes.
This study used two control groups: liposome control group and nanoparticle control group. Since previous work in our lab showed that the tumor volume of the liposome control group was not significantly different than the tumor volume in untreated controls (Lawson et al. Citation2003), an untreated group was not included in this study. Since there was a substantial antitumor effect by the control nondrug-containing nanoparticles, the liposome control group was used for comparing the anticancer efficacy of all treatment groups.
Data showed α -TEA high dose formulated into either liposome or nanoparticle to be effective in reducing tumor volume when evaluated in comparison to liposome or nanoparticle controls, respectively. Low dose α -TEA formulated in liposomes or nanoparticles was significantly less effective than high dose α-TEA formulated by the two methods in reducing tumor burden when compared with their respective controls. Both low and high dose α -TEA formulated in either liposomes or nanoparticles significantly reduced the average number of visible macroscopic lung metastases. High dose α -TEA formulated in liposomes as well as both low and high dose α -TEA formulated in nanoparticles significantly reduced the total numbers of lung and lymph node micrometastatic tumor foci. Low dose α -TEA formulated in liposomes reduced the mean number of lymph node metastatic lesions but not significantly different from control.
Surprisingly, the nanoparticle control group significantly reduced tumor burden in comparison to the liposome control group, with the reduction in tumor volume being similar to the tumor volume reduction observed in the liposome high dose group and nanoparticle low dose group. Furthermore, the nanoparticle control group significantly reduced the total number of lung and lymph node micrometastatic lesions in comparison to the liposome control group. However, the number of mice with visible tumors as well as the average number of visible lung tumors in the nanoparticle control group were not significantly reduced in comparison to the liposome control group. Thus, a substantial amount of the anticancer properties of the α -TEA low and high nanoparticle groups may reflect the anticancer properties of the nanoparticle vehicle.
This is the first report of which we are aware that shows that PLGA polymer nanoparticles alone delivered by gavage can significantly reduce tumor burden and lung and lymph node micrometastases. Although we do not know the mechanisms whereby PLGA polymers reduce tumor burden, it is possible that the PLGA polymer delivered at higher levels via gavage delivery versus smaller amounts via IV delivery may (i) exhibit tumor cell specific toxicity or may (ii) induce enhancement of immune killing of tumor cells. Regarding toxicity, based on body weights, none of the α -TEA treatment or control groups showed any overt toxicity. It is important to note that both chemicals used in the nanoparticle formulation, namely, PLGA and surfactant Tween 80, have FDA approval for use in drug delivery systems (Feng et al. Citation2004; Panyam et al. Citation2002; Shive and Anderson Citation1997). PLGA undergoes hydrolysis, forming biologically compatible and metabolizable moieties (lactic acid and glycolic acid) that are eventually removed from the body by the citric acid cycle (Shive and Anderson Citation1997). Tween 80 is a food-grade surfactant commonly used in dietary supplements, flavoring agents, whipped toppings and shortenings (Abriola and Pennell Citation1994). Therefore, nanoparticles made from these materials were expected to be nontoxic.
Nevertheless, the nanoparticle control exhibited significant anticancer efficacy and these anticancer effects were not associated with increased ability to induce apoptosis. Some type of tumor specific toxicity is a possibility that warrants further investigation. Support for the second possible explanation comes from studies showing enhancement of antitumor immunity by PLGA nanoparticles used for delivery of vaccine (Jilek et al. Citation2004; Yoshida and Babensee Citation2004). These studies showed that PLGA when formed into nanoparticles enhanced maturation of monocyte-derived dendritic cells. As antigen-presenting cells, mature dendritic cells could enhance antitumor immunity (Jilek et al. Citation2004; McKenna et al. Citation2005; Yoshida and Babensee Citation2004). To investigate possible immune involvement, immune cell infiltration of tumors from liposome and nanoparticle control groups was evaluated. No detectable differences in intratumoral B or T lymphocyte numbers between the liposome and nanoparticle controls at euthanasia were observed, providing no support for T and B cell involvement at this one time point.
CONCLUSION
α -TEA formulated in either nanoparticles or liposomes at a dosage of 5 mg/day was very effective in inhibiting tumor growth and lung metastases. α -TEA at 2.5 mg/day formulated in nanoparticles was more effective than α -TEA at 2.5 mg/day formulated in liposome in reducing tumor burden and lymph node and lung micrometastatic tumor foci. However, the difference between the two formulations could be attributed to a nanoparticle effect rather than an α -TEA effect since nanoparticles alone significantly reduced tumor burden and metastases. These studies show that oral delivery of α -TEA at an appropriate dosage formulated in either liposomes or nanoparticles to be an effective, clinically relevant means for administrating this hydrophobic drug.
This work was supported by Public Health Service Grant CA59739 and the Foundation for Research (to K. Kline B. G. Sanders) and by the National Institute of Environmental Health Sciences Center Grant ES07784 (of which they both are members).
We thank John Mendenhall (Institute of Cell and Molecular Biology, U.T. Austin, TX), John N. Wright (Institute of Biomedical Engineering, U.T. Austin, TX), and Dr. Ti Cao (Institute of Chemistry and Biochemistry, U.T. Austin, TX) for help and advice in the development and characterization of nanoparticles. We thank Dr. LuZhe Sun (University of Texas Health Science Center at San Antonio, TX) for providing the 66cl-4-GFP murine mammary tumor cell line. We thank Dr. Mark McArthur (University of Texas M.D. Anderson Cancer Center, Department of Veterinary Sciences, Bastrop, TX) for evaluating H&E-stained tumor tissue sections for cellular infiltration. We thank the University of Texas MD Anderson Histology Core Facility (Science Park, Bastrop, TX) for preparation of H&E and immunohistochemically stained tissues. And we thank Dr. Howard Thames, director of the Biostatistics and Data Processing Core (University of Texas M.D. Anderson, Houston, TX) for help with statistical analyses.
REFERENCES
- Abriola L. M., Pennell K. D. Surfactant Flushing Research to Remove Organic Liquids from Aquifers. United States Environmental Protection Agency. National Center for Environmental Publications and Information, Washingtan, DC 1994
- Anderson K., Lawson K. A., Simmons-Menchaca M., Sun L., Sanders B. G., Kline K. Alpha-TEA plus cisplatin reduces human cisplatin-resistant ovarian cancer cell tumor burden and metastasis. Exp. Biol. Med. 2004a; 229: 1169–1176
- Anderson K., Simmons-Menchaca M., Lawson K. A., Atkinson J., Sanders B. G., Kline K. Differential response of human ovarian cancer cells to induction of apoptosis by vitamin E succinate and vitamin E analogue, alpha-TEA. Cancer Res. 2004b; 64: 4263–4269
- Clarke R. Issues in experimental design and endpoint analysis in the study of experimental cytotoxic agents in vivo in breast cancer and other models. Breast Cancer Res Treat. 1997; 46: 255–278
- Davda J., Labhasetwar V. Characterization of nanoparticle uptake by endothelial cells. Int. J. Pharm. 2002; 233: 51–59
- De S., Robinson D. H. Particle size and temperature effect on the physical stability of PLGA nanospheres and microspheres containing Bodipy. AAPS PharmSciTech 2004; 5: e53
- Dexter D. L., Kowalski H. M., Blazar B. A., Fligiel Z., Vogel R., Heppner G. H. Heterogeneity of tumor cells from a single mouse mammary tumor. Cancer Res. 1978; 38: 3174–3181
- Feng S. S., Mu L., Win K. Y., Huang G. Nanoparticles of biodegradable polymers for clinical administration of paclitaxel. Curr. Med. Chem. 2004; 11: 413–424
- Hofheinz R. D., Gnad-Vogt S. U., Beyer U., Hochhaus A. Liposomal encapsulated anti-cancer drugs. Anticancer Drugs 2005; 16: 691–707
- Jain R. A. The manufacturing techniques of various drug loaded biodegradable poly(lactide-co-glycolide) (PLGA) devices. Biomaterials 2000; 21: 2475–2490
- Jilek S., Ulrich M., Merkle H. P., Walter E. Composition and surface charge of DNA-loaded microparticles determine maturation and cytokine secretion in human dendritic cells. Pharm. Res. 2004; 21: 1240–1247
- Lawson K. A., Anderson K., Menchaca M., Atkinson J., Sun L., Knight V., Gilbert B. E., Conti C., Sanders B. G., Kline K. Novel vitamin E analogue decreases syngeneic mouse mammary tumor burden and reduces lung metastasis. Mol Cancer Ther. 2003; 2: 437–444
- Lawson K. A., Anderson K., Simmons-Menchaca M., Atkinson J., Sun L., Sanders B. G., Kline K. Comparison of vitamin E derivatives alpha-TEA and VES in reduction of mouse mammary tumor burden and metastasis. Exp. Biol. Med. 2004; 229: 954–963
- Lee L. J. Polymer nano-engineering for biomedical applications. Ann. Biomed. Eng. 2006; 34: 75–88
- Lin S. Y., Chen K. S., Teng H. H., Li M. J. In vitro degradation and dissolution behaviours of microspheres prepared by three low molecular weight polyesters. J Microencapsul. 2000; 17: 577–586
- McKenna K., Beignon A. S., Bhardwaj N. Plasmacytoid dendritic cells: linking innate and adaptive immunity. J. Virol. 2005; 79: 17–27
- Miller B. E., Roi L. D., Howard L. M., Miller F. R. Quantitative selectivity of contact-mediated intercellular communication in a metastatic mouse mammary tumor line. Cancer Res. 1983; 43: 4102–4107
- Panyam J., Labhasetwar V. Biodegradable nanoparticles for drug and gene delivery to cells and tissue. Adv Drug Deliv Rev. 2003a; 55: 329–347
- Panyam J., Labhasetwar V. Dynamics of endocytosis and exocytosis of poly(D,L-lactide-co-glycolide) nanoparticles in vascular smooth muscle cells. Pharm. Res. 2003b; 20: 212–220
- Panyam J., Zhou W. Z., Prabha S., Sahoo S. K., Labhasetwar V. Rapid endo-lysosomal escape of poly(DL-lactide-co-glycolide) nanoparticles: implications for drug and gene delivery. FASEB J. 2002; 16: 1217–1226
- Shive M. S., Anderson J. M. Biodegradation and biocompatibility of PLA and PLGA microspheres. Adv. Drug Deliv. Rev. 1997; 28: 5–24
- Sinha J., Raay B., Das N., Medda S., Garai S., Mahato S. B., Basu M. K. Bacopasaponin C: critical evaluation of anti-leishmanial properties in various delivery modes. Drug Deliv. 2002; 9: 55–62
- Smigal C., Jemal A., Ward E., Cokkinides V., Smith R., Howe H. L., Thun M. Trends in breast cancer by race and ethnicity: update 2006. CA Cancer J. Clin. 2006; 56: 168–183
- Tirmenstein M. A., Watson B. W., Haar N. C., Fariss M. W. Sensitive method for measuring tissue alpha-tocopherol and alpha-tocopheryloxybutyric acid by high-performance liquid chromatography with fluorometric detection. J. Chromatogr. B Biomed. Sci. Appl. 1998; 707: 308–311
- Yoshida M., Babensee J. E. Poly(lactic-co-glycolic acid) enhances maturation of human monocyte-derived dendritic cells. J. Biomed. Mater. Res. A. 2004; 71: 45–54
- Zamboni W. C. Liposomal, nanoparticle, and conjugated formulations of anticancer agents. Clin. Cancer Res. 2005; 11: 8230–8234
- Zhang S., Lawson K. A., Simmons-Menchaca M., Sun L., Sanders B. G., Kline K. Vitamin E analog alpha-TEA and celecoxib alone and together reduce human MDA-MB-435-FL-GFP breast cancer burden and metastasis in nude mice. Breast Cancer Res. Treat. 2004; 87: 111–121