Abstract
Insulin stability during microencapsulation and subsequent release is essential for retaining its biological activity. The successful delivery of insulin relies on the proper selection of stabilizers in addition to other parameters. Attempts were made to address the problem with a few combination of stabilizers for maintaining the integrity of insulin during formulation and delivery. Insulin loaded nanoparticles with different stabilizers such as pluronic F68, trehalose, and sodium bicarbonate were prepared by the double emulsion evaporation method using two different copolymer ratios of poly(DL-lactide-co-glycolide) (50:50 and 85:15). The presence of stabilizers in the nanoparticles resulted in an increase in the particle size but a reduction of encapsulation efficiency. Insulin release rate was comparatively higher for the batches containing stabilizers when compared with controls for both the copolymer ratios. Also the presence of stabilizers resulted in sustained release of insulin resulting in prolonged reduction of blood glucose levels in streptozotocin induced diabetic rats. From the in vitro and in vivo studies, we concluded that a combination of stabilizers results in beneficial effects without compromising the advantages of delivery systems.
Conventional treatment of diabetes by multiple daily administration of subcutaneous insulin injection does not maintain normoglycemia adequately, as the pharmacokinetics following exogenous administration of insulin does not match with the physiological profiles of insulin secretion. The resulting hyperglycemia is responsible for many of the macro- and microvascular complications of diabetes namely retinopathy, neuropathy, and cardiovascular complications. Improved glycemic control in type 1 diabetes can be achieved by using the delivery systems, that can prolong and control the release of insulin.
Biodegradable polymers such as poly (DL-lactide-co-glycolide) (PLGA) have been successfully used for formulating microspheres/nanoparticles to deliver proteins and peptides at controlled rates over a prolonged period of time (Crotts et al.Citation1998). The maintenance of insulin stability during microencapsulation and subsequent release is essential for retaining its biological activity. Insulin is a hydrophilic, unstable peptide susceptible to both chemical and physical degradation. During the formulation of microemulsions, usually proteins tend to migrate to the interface between the aqueous and organic phase where they may unfold resulting in conformational changes. It was also found to undergo aggregation and subsequent loss of activity following encapsulation by w/o/w solvent evaporation technique (Wang et al. Citation1998).
Also exposure to various formulation processes such as elevated temperature, low pH, organic solvents, and agitation result in biologically inactive linear aggregates (fibrils) of insulin. The acidic degradation products of PLGA can interact with positively charged proteins leading to blockade in its release and also expose a low pH to the entrapped drug forming water, insoluble noncovalently bound protein aggregates. This low pH environment is the cause of deamidation at asparaginase A21 of insulin moiety (Morlock et al. Citation1997). Thus insulin instability has become a strong obstacle in the development of suitable controlled delivery systems.
To overcome these problems, various stabilizers have been used to preserve the integrity of the peptide. The additions of block copolymers of polyethylene-polypropylene glycols such as poloxamers have been shown to stabilize the proteins effectively. Among the different poloxamers, poloxamer 188 was found to be more efficient in preventing the insulin aggregation in solution. In addition, poloxamers stabilize primary emulsion and reduce protein-polymer interactions (Nilhant et al. Citation1994). During the secondary drying phase, the removal of water molecules also contributes to the protein aggregation (Gribbon et al. Citation1996). Addition of the carbohydrate trehalose overcomes these problems and prevents secondary structure changes of the protein.
The low pH microenvironment (< 3) generated by the degradation of PLGA polymers which triggers the unfolding of proteins was neutralized by incorporation of salts such as magnesium hydroxide, preventing the protein aggregation (Zhu et al. Citation2000). Based on this hypothesis, sodium bicarbonate was used for the neutralization of the acidic environment. The objective of our present work was to develop insulin-loaded nanoparticles using a combination of different stabilizers such as pluronic F68, trehalose, and sodium bicarbonate to improve the insulin stability while optimizing nanoparticles characteristics. The effects of the stabilizers on nanoparticles properties such as morphology, encapsulation efficiency, and in vitro release profile were investigated along with the in vivo reduction of blood glucose levels in diabetic rats.
MATERIALS AND METHODS
Insulin USP-Hu. Recom. Cryst (1 mg = 27.5 IU) was purchased from Serologicals Corporation (Norcross, GA, USA). Poly (DL-lactide-co-glycolide) (85:15, 50:50), Pluronic F68, sodium azide, trehalose, and streptozotocin were obtained from Sigma-Aldrich (St. Louis, MO, USA). Sodium bicarbonate, polyvinyl alcohol (PVA), sodium hydroxide, disodium hydrogen phosphate, and sodium dihydrogen phosphate were of analytical grade (Loba Chemie, Mumbai, India). Triflouroacetic acid (TFA) was from E-Merck (Mumbai, India). Dichloromethane and acetonitrile were of HPLC grade (Qualigens Fine Chemicals, Mumbai, India).
Preparation of Insulin-Loaded Nanoparticles
Insulin-loaded nanoparticles were prepared by a modified double emulsion-solvent evaporation technique (Rosa et al. Citation2000). Briefly, 0.2 ml of aqueous phase containing insulin (5% and 12% theoretical loading) dissolved in 0.01M hydrochloric acid, 10 mM trehalose, 10% sodium carbonate, and 1% pluronic were added to the internal aqeous phase. The primary emulsion formed was emulsified with 100 ml of 2% w/v aqueous PVA at 60W for 2 min to form the double emulsion. The emulsion was stirred at room temperature to allow complete evaporation of the organic phase. The nanoparticles were collected by centrifugation, washed with distilled water, and lyophilized. The lyophilized nanoparticles were stored at 4°C in the presence of silica gel.
Morphology and Size
The surface morphology of the nanoparticles was analyzed by scanning electron microscopy (SEM) (Hitachi S-250, Japan). The nanoparticles were mounted on aluminium stubs, coated with gold under vacuum, and observed under SEM. Size analysis was carried out by photon correlation spectroscopy technique using Zeta sizer (3000 HS, Malvern Instruments, UK) after dispersing the nanoparticles in distilled water. Particle size is expressed as mean volume diameter in nm ± SD of three batches.
Insulin Analysis by HPLC
Insulin analysis was carried out by reverse-phase HPLC using a LiChrospher® C8 (250 × 4 mm, 5 μ m) column at ambient temperature at 214 nm (LC10 ATvp UV-visible detector, Shimadzu, Japan) equipped with an integrator (CR 8A, Shimadzu, Japan). The mobile phase was a mixture of an aqueous solution of 0.15% TFA (pH 2.5) and acetonitrile in the ratio of 65:35 run in isocratic mode at a flow rate of 0.7 ml/min.
Encapsulation Efficiency Determination
About 5 mg of insulin-loaded nanoparticles were dissolved in 1 ml of acetonitrile to dissolve PLGA, and insulin was extracted by adding 2 ml of phosphate buffer saline (PBS). The aqueous phase was separated, and filtered through a 0.22 μ nylon membrane filter, and an aliquot 20 μ l was injected for HPLC analysis. The results are expressed as percent ratio of actual and theoretical loading.
In Vitro Release and Stability Studies
Weighed amount (20 mg) of drug-loaded PLGA nanoparticles was suspended in 1 ml PBS containing 0.001% sodium azide as preservative and kept for incubation at 37°C at 25 rpm (Innova 4230, New Brunswick Scientific, USA). At predetermined time intervals, 0.5 ml of sample were withdrawn and fresh PBS added. The samples were filtered and analyzed for insulin content and stability by HPLC using a pure insulin sample as reference. Insulin release is expressed in cumulative percentage.
In Vivo Studies in Rats
The in vivo efficacy of insulin loaded nanoparticles was carried out in streptozotocin induced diabetic rats. The animal experiments were carried out in accordance with the guidelines and protocol was approved by the Institutional Animal Ethics Committee (IAEC, IICT, Hyderabad). Wistar rats of either sex weighing between 150–180 g were used. Streptozotocin (45 mg/kg, intraperitoneal) was administered to render animals diabetic. Blood glucose levels were monitored daily for 3 weeks for all the rats until the induction and stabilization of diabetic state. Diabetic animals were divided into 6 groups of 3 animals each and were administered subcutaneously with nanoparticles at insulin dose of 5 IU/kg. Blood samples were collected at 0, 2, 4, 8, 12, 24, 48, 60, and 72 hr after dosing. Serum was separated immediately and blood glucose levels were determined by glucose oxidase method using auto blood analyzer (Express Plus, Bayer Corporation, USA). The percent reduction of blood glucose levels was calculated from the zero hour reading.
RESULTS AND DISCUSSION
The successful delivery of insulin relies on the proper selection of stabilizers in addition to other parameters. Insulin loaded nanoparticles with different stabilizers were prepared by the double emulsion evaporation method using two different copolymer ratios of PLGA (50:50 and 85:15). The influence of various stabilizers on the nanoparticle characteristic parameters such as mean particle size, actual loading, encapsulation efficiency, and percent yield are given in .
TABLE 1 Characterization of insulin-loaded nanoparticles prepared by double emulsion-solvent evaporation technique
Surface Morphology and Particle Size
The insulin-loaded nanoparticles were found to be spherical and free flowing as evident from , irrespective of the presence of stabilizers. The nanoparticles size increased on increasing the drug loading from 5% (batch and 1c) to 12% (batch and 1d). Another interesting finding is that the mean particle size of nanoparticles was significantly less in the batches of copolymer ratio 85:15 (batches 2a and 2b) when compared with PLGA 50:50 (1a, 1b), when such other parameters as drug loading and stabilizers were kept constant. No such differences were observed between PLGA 75:25 and 50:50 in a previous report (Witschi et al. Citation1998), whereas an increase in particle size had been reported for an increase in intrinsic polymer viscosity, which was attributed to the difficulty for emulsification of high viscosity solutions (Blanco et al. Citation1998). Also the presence of stabilizers in the nanoparticles resulted in an increase in the particle size for both the copolymer ratio 50:50 (batches 1c, 1e and control 1) and 85:15 (batches 2b, 2c, and control 2). Quaglia et al. (Citation2003) reported similar results for insulin microspheres when additives were used.
FIG. 1 Scanning electron micrograph of insulin loaded nanoparticles (a) Control 1 (PLGA 50:50; 5% theoretical loading) (b) Control 2 (PLGA 85:15; 12% theoretical loading) (c) With stabilizers (Trehalose 10 mM, Pluronic® F68 1% and sodium bicarbonate 10%; PLGA 50:50; 5% theoretical loading) (d) With stabilizers (Trehalose 10 mM, Pluronic® F68 1% and sodium bicarbonate 10%; PLGA 85:15; 12% theoretical loading).
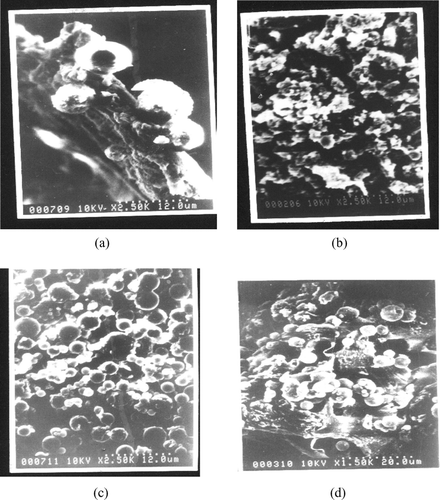
Encapsulation Efficiency
For high target loading, the encapsulation efficiency was decreased as evident from batches 1a, 1c (5% loading), and 1b, and 1d (12% loading). This may be attributed to the presence of very large pores leading to significant drug loss during the preparation (Witschi et al. Citation1998). It is noteworthy that at low drug loading (5%), the encapsulation efficiency was comparatively higher for PLGA 85:15 (batch 1a) when compared with 50:50 (batch 2a) (42.83% and 11.61%, respectively). Our results confirm a previous report (Witschi et al. Citation1998) where similar increase was noticed for PLGA 75:25 than 50:50 at 1% peptide loading. Proteins of good tensoactive properties that can interact with PLGA forming an interfacial stabilizing film at w/o interface are known to have very high encapsulation efficiency (> 90%) as in the case of bovine serum albumin (BSA). Proteins that do not exhibit tensoactive properties are entrapped by their interaction (ionic) with PLGA carboxyl-end groups that limit their entrapment efficiency as evident with lysozymes (Blanco et al. Citation1998).
Our results for the encapsulation efficiency of insulin in PLGA 50:50 (41.92%) are similar to earlier reported values (44%) (Rosa et al. Citation2000). The displacement of insulin from w/o interface by the stabilizers resulted in the reduction of encapsulation efficiency.
In Vitro Release and Insulin Stability
The cumulative amount of insulin released from the nanoparticles is given in (PLGA 50:50) and (PLGA 85:15). Insulin release was found to be biphasic, with an initial burst release of drug that usually exists near the surface through the aqueous pores or channels formed during nanoparticles preparation. The second phase involves the degradation of polymer matrix and release of the entrapped peptide through the aqueous channels by diffusion [9]. Also the amount of insulin released from the batches containing additives was comparatively higher than the controls (without additives) for both the copolymer ratios. It is evident from the HPLC chromatograms given in , where insulin peak appears intact in nanoparticles prepared with combination of stabilizers () but insulin degradation can be seen in the control group (). The higher release rate of peptide in presence of stabilizers is due to the reduced insulin-PLGA interaction that destabilize the w/o primary emulsion. This leads to coalescence of the aqueous microdroplets causing more porous structure and higher release rate. Such higher release rates in the presence of surfactants were reported earlier (Rosa et al. Citation2000; Blanco et al. Citation1998; Quaglia et al. Citation2003).
FIG. 2 (a).Influence of surfactants on the in vitro release of insulin from nanoparticles prepared with PLGA 50:50: (◊) 1a, (▪) 1b,control 1(▴) (b). Influence of surfactants on the in vitro release of insulin from nanoparticles prepared with PLGA 85:15: (⧫) 2a, (▪) 2b, (▴) Control 2.
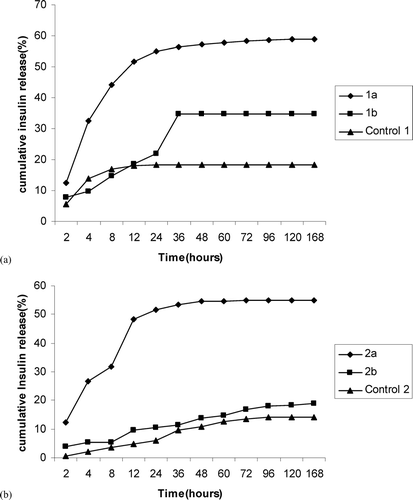
FIG. 3 (3a). HPLC chromatogram of pure insulin sample (peak at 4.594 min) (3b). HPLC chromatogram of insulin loaded nanoparticle with combination of stabilizers. Insulin peak visible at Rt 4.576 min (3c). HPLC chromatogram of insulin loaded nanoparticle without stabilizers (Control 1). Very small peak of insulin at Rt 4.5 min alongwith degradation peaks visible at Rt 6.886 min
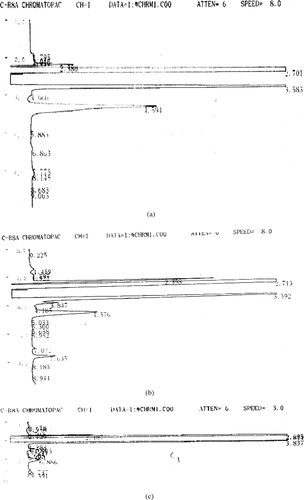
The amount of insulin released was higher for batches containing combination of stabilizers (1a, 2a), irrespective of the PLGA copolymer ratio. The addition of cryoprotectant trehalose and neutralizing sodium bicarbonate which protects the peptide from acidic degradation products of PLGA contributed to the stability of insulin in the release medium. Thus the combination of stabilizers used in this formulation provided greater stability than the individual stabilizers or the control groups.
In Vivo Studies
The results of the in vivo studies are given as mean percent reduction in the blood glucose levels at different time intervals in (PLGA 50:50) and in (PLGA 85:15). In accordance with the in vitro results, the control batches of both the groups resulted in lesser reduction of blood glucose levels for shorter time (up to 24 hr). Batches containing pluronic F68 alone (1e and 2c) and those with sodium bicarbonate and trehalose (1c and 2b) had a sustained effect in the blood glucose reduction. Especially formulations of copolymer ratio 85:15 exhibited a prolonged inhibitory effect on blood glucose levels (up to 72 hr). These results suggest the importance in the selection of appropriate stabilizers and for the first time a combination effect of different stabilizers on the insulin stability during the release from nanoparticles.
FIG. 4 (4a). Mean ± SE percent reduction of blood glucose levels in streptozotocin induced diabetic rats by insulin loaded PLGA (50:50) nanoparticles, (◊)2a, (▪)2c, (⧫) Control 2 (2b). Mean ± SE percent reduction of blood glucose levels in streptozotocin induced diabetic rats by insulin loaded PLGA (85:15) nanoparticles.
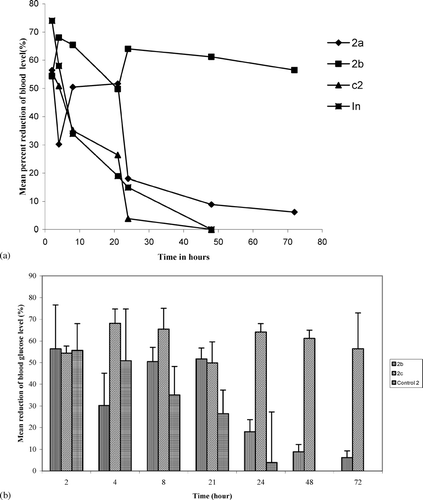
CONCLUSION
A successful sustained release formulation should release the peptide in its native (active) form. To attain this goal, a novel approach has been attempted to maintain the stability and integrity of protein/peptide in PLGA nanoparticles. Different stabilizers and their combinations have been tried for the nondestructive delivery of insulin in nanoparticles. The coencapsulation of stabilizers affected the morphology, encapsulation efficiency, in vitro release and in vivo performance of the insulin loaded nanoparticles. In general, the mean size of the nanoparticles increased due to the presence of stabilizers while there was a reduction in the encapsulation efficiency. The presence of stabilizers led to higher insulin release rates, especially when a combination of stabilizers was used. Also the presence of stabilizers resulted in sustained release of insulin resulting in prolonged reduction of blood glucose levels in streptozotocin induced diabetic rats. From the in vitro and in vivo studies, we concluded that a combination of stabilizers also results in beneficial effects without compromising the advantages of delivery systems.
The authors thank MHRD/AICTE, New Delhi, India, for providing financial assistance in the form of a Junior Research Fellowship. The authors also thank Dr. J.S. Yadav, director of IICT, for his support and encouragement to carry out the work.
REFERENCES
- Blanco D., Alonso M. J. Protein encapsulation and release from poly (lactide-co-glycolide) microspheres: effect of the protein and polymer properties and of the coencapsulation of surfactants. Eur. J. Pharm. Biopharm. 1998; 45: 285–294
- Crotts G., Park T. G. Protein delivery from poly (lactic-co-glycolic acid) biodegradable microspheres: release kinetics and stability studies. J. Microencapsul. 1998; 15: 699–713
- Gribbon E. M., Hatley R. H. M., Gard T., Blair J. A., Kampinga J., Roser J. Trehalose and novel hydrophobic sugar glasses in drug stabilization and delivery. Chemical Aspects of Drug Delivery Systems, D. R. Karsa, R. A. Stephenson. RSC, London 1996; 138–145
- Morlock M., Koll H., Winter G., Kissel T. Microencapsulation of rh-erythropoietin, using biodegradable poly(DL-lactide-co-glycolide): protein stability and the effects of stabilizing excipients. Eur. J. Pharm. Biopharm. 1997; 43: 29–36
- Nilhant N., Schugens Ch., Grandfils Ch., Jerome R., Teyssie Ph. Polylactide microparticles prepared by double emulsion/evaporation technique. I. Effect of primary emulsion stability. Pharm. Res. 1994; 11: 1479–1484
- Quaglia F., Rosa G. D., Granata E., Ungaro F., Fattal E., La Rotonda M. I. Feeding liquid, non-ionic surfactant and cyclodextrin affect the properties of insulin-loaded poly(lactide-co-glycolide) microspheres prepared by spray-drying. J. Control. Rel. 2003; 86: 267–278
- Rosa G. D., Iommelli R., La Rotonda M. I., Miro A., Qualgia F. Influence of the co-encapsulation of different non-ionic surfactants on the properties of PLGA insulin-loaded microspheres. J. Control. Rel. 2000; 69: 283–295
- Wang N., Wu X. S. A novel approach to stabilization of protein drugs in poly (lactic-co-glycolic acid) microspheres using agarose hydrogel. Int. J. Pharm. 1998; 166: 1–14
- Witschi C., Doelker E. Influence of the microencapsulation method and peptide loading on poly (lactic acid) and poly (lactic-co-glycolic acid) degradation during in vitro testing. J. Control. Rel. 1998; 51: 327–341
- Zhu G., Mallery S. R., Schwendeman S. P. Stabilization of proteins encapsulated in injectable poly (lactide-co-glycolide). Nat. Biotech. 2000; 18: 52–57