Abstract
The in vitro dissolution, swelling, and erosion behavior of monolithic matrix systems containing the well-known hydrophilic polymer, hydroxypropylmethylcellulose, and a combination of chitosan and polycarbophil in the form of an interpolyelectrolyte complex were compared in this study. The two different types of matrix systems showed both a combination of swelling and erosion as the drug release mechanism. Kinetic analysis of the in vitro release profiles of water-soluble drugs from the matrix tablets illustrated that those containing the chitosan-polycarbophil complex exhibited higher mean dissolution time values and therefore slower drug release compared with the other matrix systems. The analysis also indicated that zero-order release kinetics were approached for some of the formulations containing the chitosan-polycarbophil complex, while this could not be achieved for those containing hydroxypropylmethylcellulose.
Hydroxylpropylmethylcellulose (HPMC), approved by the Food and Drug Administration (FDA) of the United States of America as an excipient, is one of the most popular hydrophilic carrier materials used to produce oral drug sustained release systems (Colombo Citation1993; Siepmann and Peppas Citation2001; Jamzad and Fassihi Citation2006). HPMC is a water-soluble polymer and it is generally recognized that drug release from HPMC based matrices occurs by means of drug diffusion through the swelling gel layer and by surface erosion of this layer (Ford et al. Citation1987; Rao, Devi, and Buri Citation1990; Colombo Citation1993; Tahara, Yamamoto, Nishihata, Citation1995; Reynolds et al. Citation1998; Siepmann et al. Citation1999; Siepmann and Peppas Citation2001). The major advantages of HPMC-based matrix drug delivery systems with a combination of drug release mechanisms (i.e., swelling and erosion) are 3-fold; minimal burst release effects; the drug release rate approaches a constant value; and the possibility of predicting the effect of the device design parameters (e.g., shape, size, and composition) on the drug release rate (Colombo Citation1993; Siepmann and Peppas Citation2001).
Chitosan is a deacetylated derivative of the natural polysaccharide, chitin, and is positively charged in acidic environments with pH values below 6.5. It is relatively inexpensive, digestible, and biocompatible and therefore is regarded as a promising material for the production of drug delivery systems (Paul and Sharma Citation2000). Chitosan has been successfully used to form complexes with natural anionic polymers such as carboxymethylcellulose, alginic acid, dextran sulfate, carboxymethyl dextran, heparin, carrageenan, pectin, methacrylic acid copolymers, (Eudragit® polymers) and xanthan (Dumitriu and Chornet Citation1998; Berger et al. Citation2004; Sankalia et al. Citation2007; Margulis and Moustafine Citation2006). Polycarbophil is an acrylic acid polymer cross-linked with divinyl glycol that swells in water up to 1000 times its original volume to form a gel (Hosmani Citation2006).
Interpolyelectrolyte complexes (IPECs) are formed as precipitates by mixing two oppositely charged polymers in an aqueous solution. IPECs have emerged as an important class of polymer carriers, which have started to play a significant role in creating novel oral drug delivery systems (Peppas and Khare Citation1993; Berger et al. Citation2004). Various IPECs have been used in polymeric based controlled drug release systems (Peppas and Khare Citation1993; Garcia and Ghaly Citation1996; Lorenzo-Lamoza et al. Citation1998; Sopprinath and Aminabhavi Citation2002; Chen et al. Citation2004; Nam, Watanabe, Ishihara, Citation2004; Moustafine et al. Citation2005).
Modified release drug delivery systems intended for the oral administration route are mainly manufactured in the form of matrices. These matrix systems are prepared by compressing a mixture of materials that include a drug dispersed in a polymer mass, and very often soluble or insoluble fillers also are added (Colombo Citation1993). Various polymers with different structural designs, including linear, branched, star-like, and comb-like, as well as combinations of polymers have been used to improve drug delivery from matrix systems such as physical mixtures (e.g., polymer blends and interpenetrating networks) and chemically linked copolymers (Pillai and Panchagnula Citation2001).
In this study, the in vitro drug release, swelling, and erosion properties of compressed matrix drug delivery systems containing a chitosan-polycarbophil IPEC were compared with those that contain HPMC as the polymer component.
MATERIALS AND METHODS
Chitosan (Warren Chem Specialities, South Africa, deacetylation degree = 91.25%), polycarbophil (Noveon, Cleveland, USA), hydroxylpropylmethylcellulose (Methocel K100M, K100LV Premium, Colorcon Ltd., England), sinomenine hydrochloride (Ankang Chia Tai Pharmaceutical China), ranitidine hydrochloride (Dolder Ltd., Switzerland), microcrystalline cellulose (Avicel®, pH101, FMC corporation NV, Belgium), and sodium carboxymethyl starch (Explotab®, Edward Mendell Co., USA), were purchased. All other chemicals were of analytical grade and used as received.
Preparation of the IPEC
Chitosan (30 g) was dissolved in 1000 ml of a 2% v/v acetic acid solution and polycarbophil (90 g) was dispersed in 3000 ml of a 2% v/v acetic acid solution. The chitosan solution was added slowly to the polycarbophil dispersion under homogenization (5200 rpm, ZKA®, Germany) over a period of 20 min. The mixture was then mechanically stirred for 1 hr at a speed of 1200 rpm (Heidolph RZR2021, Germany). The gel formed was separated by centrifugation for 5 min at 3000 rpm and then washed several times with a 2% v/v acetic acid solution to remove any unreacted polymeric material. The gel was then freeze-dried for a period of 48 hr (Jouan LP3, France) and the lyophilized powder was screened through a 300 μ m sieve.
Characterization of the IPEC
The formation of the IPEC between chitosan and polycarbophil was characterized by means of differential scanning calorimetry (Lu, Chen, and Hamman, Citation2007) and infrared (IR) spectrometry. This characterization was done to prove interaction between the two polymers and to show that the product was not a simple physical mixture of the two polymers. The chitosan-polycarbophil IPEC also was characterized by Fourier transform infrared (FT-IR) spectroscopy. Infrared spectral data of the chitosan-polycarbophil IPEC was obtained on a FTS-175C spectrophotometer (Bio-Rad, USA) using the potassium bromide (KBr) disk method.
Preparation of Matrix Tablets
Monolithic matrix tablets containing the water-soluble model drugs, sinomenine hydrochloride and ranitidine hydrochloride, respectively, were prepared by compressing a mixture of ingredients with varying concentrations of the polymer components as indicated in . The ingredients of the different formulations were premixed manually by stirring them in a 1000 ml glass beaker for 30 min with a spatula. After the addition of 0.05 g magnesium stearate (0.5% w/w), the powder mass was mixed for another 10 min. The powder mixture was then compressed using a rotary tablet compression machine (Cadmach, India) fitted with round, shallow punches to produce matrix-type tablets with a 6 mm diameter.
TABLE 1 Formulations (F1–F12) of the different matrix tablets
Characterization of the Matrix Tablets
Physical Properties
The weight variation test was conducted by weighing 20 randomly selected matrix tablets individually, then calculating the average weight and comparing the individual tablet weights to the average. The specification for weight variation is a 10% variation from the average weight if the average weight < 0.08 g (USP 2006).
The hardness of 10 randomly selected matrix-type tablets of each formulation was determined using a hardness tester (TBH 220 Erweka®, Germany). The force (N) needed to break each tablet was recorded. The thickness of each of 10 randomly selected matrix-type tablets was measured with a vernier calliper (accuracy = 0.02 mm). The thickness of the tablet should be within 5% of the average value.
A friability test was conducted on the matrix-type tablets using an Erweka friabilator (TA3R, Germany). Twenty matrices were randomly selected from each formulation and any loose dust was removed with the aid of a soft brush. The selected tablets were weighed accurately and placed in the drum of the friabilator. The drum was rotated at 25 rpm for 4 min after which the matrices were removed. Any loose dust was removed from the matrices before they were weighed again. The friability maximal limit is 1% (USP 2006) and calculated using the following equation:
where F is the friability, Wbefore is the initial weight of the matrices, and Wafter is the weight of the matrices after test.
Swelling and Erosion
Three matrix-type tablets from each formulation were weighed individually before transferring each tablet to a dissolution vessel containing 900 ml phosphate buffer (pH 7.4) at 37.0 ± 0.5°C, which was stirred at 50 rpm with a paddle. At 2-hr intervals up to 12 hr, the tablets were removed from the vessels and gently wiped with a tissue, weighed, and then dried at 60°C until constant weight was achieved. The mean weight was determined for the three tablets (therefore conducted in triplicate) of each formulation both in the wet state just after removal and after drying at each time interval. The data obtained from this experiment were used to calculate the swelling index and percentage mass loss, which gives an indication of matrix erosion.
Swelling Index
The swelling index (or degree of swelling) was calculated according to the following equation:
where SI is the swelling index, Ws and Wd are the swollen and dry matrix weights, respectively, at immersion time t in the buffer solution.
Percentage Matrix Erosion
The percentage matrix erosion was calculated from the weight of the matrices obtained at each time interval of the swelling experiment after drying in relation to the initial dry weight, according to the following equation:
where dry weight (t) is the weight of the matrix after drying when removed from the buffer at time t.
Drug Content
The drug content of the matrix tablets was determined by crushing 10 randomly selected tablets from each formulation in a mortar and pestle. Approximately 40 mg powder from the sinomenine HCl or 5 mg powder from ranitidine HCl containing matrices were weighed accurately and separately transferred into a 200-ml volumetric flask, which was then made up to volume with phosphate buffer solution (pH 7.4). This mixture was stirred for 30 min to allow release of the drug. After filtration through a 0.45 μ m filter membrane, the solution was assayed using ultraviolet (UV) spectrophotometry (Helios a, Thermo®, England) at a wavelength of 265 nm for sinomenine HCl and 313 nm for ranitidine HCl. The assay for drug content was performed in triplicate for each formulation. The percentage drug content of the tablets was calculated by means of the following equation:
where DC is the drug content, Wdrug is the weight of the drug, and Wmt is the weight of the matrix tablet.
Drug Release Profiles
The USP (2006) dissolution apparatus 2 (i.e., paddle) was used to determine the in vitro drug release from the different polymer-based matrix tablets. The dissolution medium (900 ml) consisted of phosphate buffer solution (pH 7.4) at 37 ± 0.5°C with a rotation speed of 50 rpm. Three sinomenine HCl or one ranitidine HCl matrix tablet of each formulation was introduced into each of three dissolution vessels (i.e., in triplicate) in a 6-station dissolution apparatus (TDT-08L, Electrolab®, India). Samples (5 ml) were withdrawn at predetermined time intervals, and 5 ml of preheated dissolution medium was used to replace every withdrawn sample immediately. Sink conditions were maintained throughout the study. Samples were filtered through a 0.45 μ m membrane, drug content in the solution was determined with UV spectrophotometry.
Kinetic Analysis of Drug Release
Drug release from simple swellable and erosion drug delivery systems may be described by the well-known power law expression and is defined by the following equation (Ritger and Peppas Citation1987; Pillay and Fassihi Citation1999):
where Mt is the amount of drug released at time t, M∞ is the overall amount of drug released, K1 is the release constant, n is the release or diffusional exponent, Mt/M∞ is the cumulative drug concentration released at time t (or fractional drug release).
The release exponent (n) is used for the interpretation of the release mechanism from polymeric matrix controlled drug release systems (Peppas Citation1985). For the case of cylindrical tablets, n ≤ 0.45 corresponds to Fickian diffusion release (case I diffusional), 0.45 < n < 0.89 to anomalous (non-Fickian) transport, n = 0.89 to zero-order (case II) release kinetics, and n > 0.89 to supercase II transport (Ritger and Peppas Citation1987). The dissolution data were modelled by using the Power law equation 5 with graph analysis software (Origin® Scientific Graphing and Analysis software, version 7, OriginLab Corporation, USA) using the Gaussian-Newton (Levenberg-Hartely) approach.
Mean Dissolution Time (MDT)
MDT is a statistical moment that describes the cumulative dissolution process and provides a quantitative estimation of the drug release rate. A higher MDT value indicates a slower drug release from the delivery system. It is defined by the following equation (Reppas and Nicolaides Citation2000; Sousa et al. Citation2002):
where MDT is the mean dissolution time, Mt is the amount of the drug released at time t; ti is the time (min) at the midpoint between i and i-1, and M∞ is the overall amount of the drug released.
Difference and Similarity Factors
The difference factor, f1 is a measure of the relative error between two dissolution curves and the similarity factor f2 is a measure of similarity in the percentage dissolution between two dissolution curves (Moore and Flanner Citation1996). Assuming that the percentage dissolved values for two profiles cannot be higher than 100, the difference factor f1 can have values from 0 (when no difference between the two curves exists) to 100 (when maximum difference exists). With the same assumption holding, the similarity factor f2 can have values from 100 (when no difference between the two curves exists) to 0 (when maximum difference exists) (Moore and Flanner Citation1996; Pillay and Fassihi Citation1999; Reppas and Nicolaides Citation2000).
In this study, they were used to confirm the differences, if any, between the drug release profiles of the water soluble model drugs from the two different polymer based matrix tablets. The difference factor (f1) and similarity factor (f2) are defined by the following equations:
where n is the number of sample withdrawal points, Rt is the percentage of the reference product dissolved at time t, Tt is the percentage of the test product dissolved at time t.
RESULTS AND DISCUSSION
Characterization of the IPEC
The formation of a separate chemical entity that is not just a simple mixture between chitosan and polycarbophil was confirmed previously by means of differential scanning calorimetry (Lu et al. Citation2007) and the formation of the interactions between these two polymers was further confirmed by means of FT-IR spectroscopy. shows the FT-IR spectra of chitosan, polycarbophil, and the product that was formed between these two polymers.
FIG. 1 Infrared spectra of chitosan, polycarbophil, and the IPEC prepared between these two polymers.
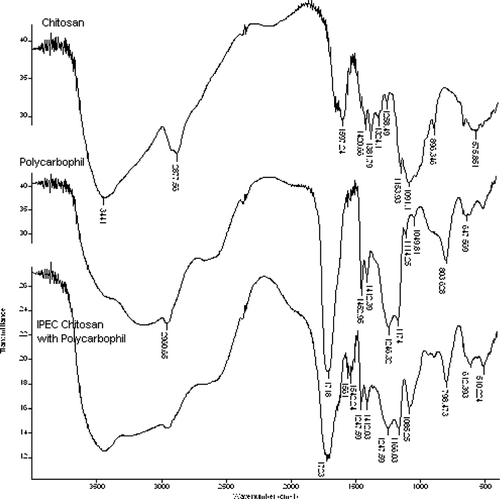
From it is clear that a peak appeared at 1561 cm− 1on the IR spectrum of the chitosan-polycarbophil IPEC, which may be assigned to the carboxylate groups of polycarbophil that formed ionic bonds with the protonated amino groups of chitosan as previously illustrated for the interaction between Eudragit E and Eudragit L (Moustafine et al. Citation2005). This ionic bond seems to be the primary binding force for the formation of an IPEC between chitosan and polycarbophil polymer chains.
Physical Properties and Drug Content of Matrix Tablets
As summarized in , the matrix-type tablets showed good thickness uniformity that varied only slightly between 3.40 ± 0.04 and 3.70 ± 0.06 mm, whereas the weight of the different formulations varied between 75.8 ± 2.3 mg and 87.9 ± 4.0 mg. However, the weight variation within each formulation was very low and complied with the specification of < 10% from the average weight. The tablets also passed the friability test (< 1%) according to USP (2006) specifications. The drug content in all the formulations ranged from 18.81 ± 0.35 to 20.09 ± 0.11% (w/w).
TABLE 2 Physical characteristics and drug content of the different matrix tablets
Swelling and Erosion
illustrates the water uptake profiles of the sinomenine HCl containing matrix formulations, whereas shows that of the ranitidine HCl containing formulations. and show the percentage erosion of these different matrix-type tablets.
FIG. 2 Swelling index profiles of the matrix tablets containing sinomenine HCl (formulations F1–F6) at pH 7.4.
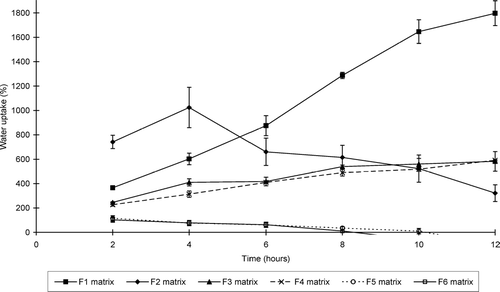
FIG. 3 Swelling index profiles of the matrix tablets containing ranitidine HCl (formulations F7–F12) at pH 7.4.
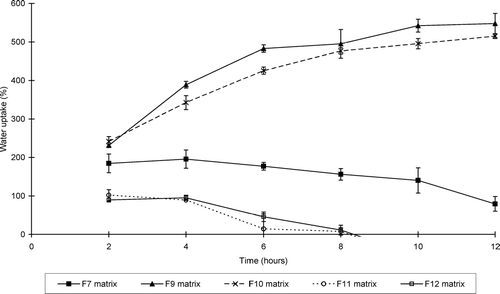
FIG. 4 Percentage erosion of sinomenine HCl-containing matrix tablets (formulations F1–F6) at pH 7.4.
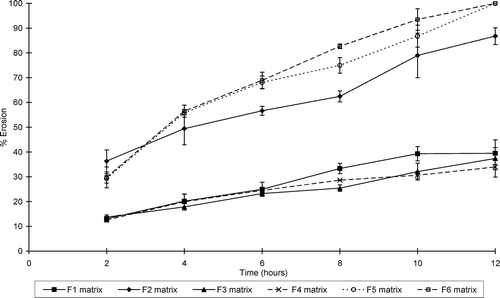
FIG. 5 Percentage erosion of the ranitidine HCl-containing matrix tablets (formulations F7–F12) at pH 7.4.
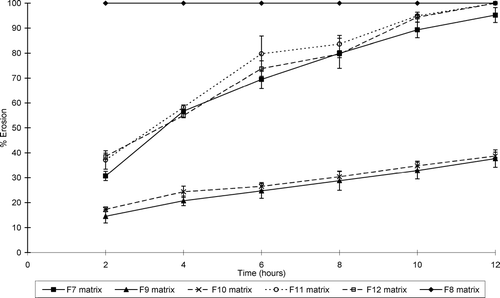
While all the matrix-type tablets showed erosion to some extent, swelling dominated in some of the systems. Water uptake therefore occurred at a faster rate and extent compared to erosion in these matrix-type tablets. Furthermore, the net weight loss of some of the matrix-type tablets does not necessarily mean that swelling did not take place in these systems, but that surface erosion occurred at a faster rate. It is important to note that the ratio of chitosan:polycarbophil (i.e., a ratio of 1:3) used in this study to produce the IPEC is an important factor to ensure erosion in conjunction with swelling in the matrix systems. For example, it has been shown that another ratio of chitosan:polycarbophil (1:1) led to the production of matrices that showed mainly swelling without erosion (Lu et al. Citation2007).
The swelling properties of the matrix systems depended on the concentration of the polymers as well as the type of the model drug and excipients included in the matrix systems. Only three sinomenine HCl containing formulations (i.e., F 1, F 3, and F 4) showed net weight gain or water uptake over the total period of the swelling experiment (i.e., 12 hr), whereas two ranitidine HCl containing formulations (i.e., F 9 and F 10) showed net water uptake over the total time period of the swelling experiment.
The percentage matrix erosion increased dramatically for some formulations, e.g., the erosion of formulation 7 increased from 30.72 ± 1.86% after 2 hr to 95.22 ± 3.00% at 12 hr. As expected, the inclusion of microcrystalline cellulose (Avicel) and sodium carboxymethyl starch (Explotab) in the matrix-type tablet formulations tended to increase both the erosion rate and extent of the matrix systems.
From these results it is clear that the rate and extent of swelling and erosion can be controlled by the composition of the matrix systems. The matrix-type tablets that contained the highest concentration chitosan-polycarbophil IPEC showed the highest swelling properties for those containing sinomenine HCl. In general, the matrix tablets containing HPMC K100LV exhibited lower swelling and higher erosion compared with the chitosan-polycarbophil IPEC containing matrix systems. In addition, the type of HPMC included in the matrix systems played an important role in their swelling and erosion behavior. HPMC with a higher viscosity (K100M) showed less erosion compared with low viscosity HPMC (K100LV).
Drug Release
The in vitro cumulative drug release values (percentage of the initial dose) were plotted as a function of time for sinomenine HCl and ranitidine HCl, respectively, from the different matrix-type tablets and are presented in and . The calculated MDT and drug release kinetic values are presented in .
TABLE 3 Release kinetics and mean dissolution times (MDT) values (formulations F 1–F 12) at pH 7.4
The matrix-type tablets containing the chitosan-polycarbophil IPEC exhibited slower drug release in general (except for formulation F 8 that eroded and released the drug relatively fast) compared with the HPMC-containing matrix systems as indicated by the higher MDT values. Furthermore, the release exponent values (ranging from 0.696 to 0.877) for the matrix systems containing the chitosan-polycarbophil IPEC indicated that the drug release from these systems approached zero-order kinetics (i.e., n = 0.89 for cylindrical tablets). This confirms that the IPEC between chitosan and polycarbophil has similar and in some cases superior properties compared with HPMC as a polymeric carrier material in controlled release matrix systems for water-soluble drugs.
The dissolution profiles obtained from the matrix systems containing the different polymeric excipients proved to be similar only in some instances such as when comparing formulations F 1 and F 3 as well as F 2 and F6, as shown in . Lack of similarity (i.e., f2 < 50) between the drug release profiles of the other formulations, as well as considering that the matrix systems containing the IPEC between chitosan and polycarbophil exhibited higher MDT values with release kinetics approaching zero-order release, indicate that the matrix systems containing the chitosan-polycarbophil IPEC as polymeric excipient performed better in terms of controlled drug release compared with those containing HPMC as polymeric excipient.
TABLE 4 Calculated difference factor (f1) and similarity factor (f2) values for comparison of the dissolution profiles of the matrix-type tablets
CONCLUSION
The IPEC between chitosan and polycarbophil showed excellent potential as an excipient for the production of monolithic matrix systems for controlling the release of water-soluble drugs. The use of this chitosan-polycarbophil IPEC in matrix-type tablets showed slower drug release (i.e., higher MDT values) and better kinetics (i.e., approaching zero-order release) in terms of constant drug release over an extended period of time compared with the well-known hydrophilic HPMC polymer that exhibited Fickian to anomalous release kinetics for the drugs tested in this study. The chitosan-polycarbophil IPEC behaves therefore as an acceptable polymeric excipient in matrix drug delivery systems. It may contribute toward expanding the list of polymeric excipients for this type of modified release drug delivery system.
REFERENCES
- Berger J., Reist M., Mayer J. M., Felt O., Peppas N. A., Gurny R. Structure and interactions in covalently and ionically crosslinked chitosan hydrogels for biomedical applications. Eur. J. Pharm. Biopharm. 2004; 57: 19–34
- Chen S.-C., Wu Y.-C., Mi F.-L., Lin Y.-H., Yu L.-C., Sung H.-W. A novel pH-sensitive hydrogel composed of N,O-carboxymethyl chitosan and alginate cross-linked by genipin for protein drug delivery. J. Control. Rel. 2004; 96: 285–300
- Colombo P. Swelling-controlled release in hydrogel matrices for oral route. Adv. Drug Deliv. Rev. 1993; 11: 37–57
- Dumitriu S., Chornet E. Inclusion and release of proteins from polysaccharide-base polyion complexes. Adv. Drug Deliv. Rev. 1998; 31: 223–246
- Ford J. L., Rubinstein M. H., McCaul F., Hogan J. E., Edgar P. J. Importance of drug type, tablet shape and added diluents on drug release kinetics from hydroxylpropyl methylcellulose matrix tablets. Int. J. Pharm. 1987; 40: 223–234
- Garcia A. M., Ghaly E. S. Preliminary spherical agglomerates of water soluble drug using natural polymer and cross-linking technique. J. Control. Rel. 1996; 40: 179–186
- Hosmani A. H. Carbopol and its pharmaceutical significance: a review. Pharmainfo 2006; 1–4
- Jamzad S., Fassihi R. Development of a controlled release low dose class II drug-Glipizide. Int. J. Pharm. 2006; 312: 24–32
- Lorenzo-Lamosa M. L., Remuñán-López C., Vila-Jato J. L., Alonso M. J. Design of microencapsulated chitosan microspheres for colonic drug delivery. J. Control. Rel. 1998; 52: 109–118
- Lu Z., Chen W., Hamman J. H. Chitosan-polycarbophil complexes in swellable matrix systems for controlled drug release. Curr. Drug Deliv. 2007; 4(4)257–263
- Margulis E. B., Moustafine R. I. Swellability testing of chitosan/Eudragit® L100–55 interpolyelectrolyte complexes for colonic drug delivery. J. Control. Rel. 2006; 116: e36–e37
- Moore J. W., Flanner H. H. Mathematical comparison of dissolution profiles. Pharm. Tech. 1996; 20: 64–74
- Moustafine R. I., Kabanova T. V., Kemenova V. A., Van den Mooter G. Characteristics of interpolyelectrolyte complexes of Eudragit E100 with Eudragit L100. J. Control. Rel. 2005; 103: 191–198
- Nam K., Watanabe J., Ishihara K. The characteristics of spontaneously forming physically cross-linked hydrogels composed of two water-soluble phospholipid polymers for oral drug delivery carrier I: hydrogel dissolution and insulin release under neutral pH condition. Eur. J. Pharm. Sci. 2004; 23: 261–270
- Paul W., Sharma C. P. Chitosan, a drug carrier for the 21st century: a review. S.T.P. Pharma Sci. 2000; 10: 5–22
- Peppas N. A. Analysis of fickian and non-fickian drug release from polymers. Pharm. Acta Helv. 1985; 60: 110–111
- Peppas N. A., Khare A. R. Preparation, structure and diffusion behaviour of hydrogels in controlled release. Adv. Drug Deliv. Rev. 1993; 11: 1–35
- Pillai O., Panchagnula R. Polymers in drug delivery. Curr. Opin. Chem. Biol. 2001; 5: 447–451
- Pillay V., Fassihi R. A new method for dissolution studies of lipid filled capsules employing nifedipine as a model drug. Pharm. Res. 1999; 16: 333–338
- Rao K. V. R., Padmalatha Devi K., Buri P. Influence of molecular size and water solubility of the solute on its release from swelling and erosion controlled polymeric matrices. J. Control. Rel. 1990; 12: 133–141
- Reppas C., Nicolaides E. Analysis of drug dissolution data. Oral Drug Absorption Prediction and Assessment, J. B. Dressman, H. Lennernäs. Marcel Dekker, New York 2000; 229–254
- Reynolds T. D., Gehrke S. H., Hussain A. S., Shenouda L. S. Polymer erosion and drug release characterization of hydroxylpropyl methylcellulose matrices. J. Pharm. Sci. 1998; 87: 1115–1123
- Ritger P. L., Peppas N. A. A simple equation for description of solute release. II Fickian and anomalous release from swellable devices. J. Control. Rel. 1987; 5: 37–42
- Sankalia M. G., Mashru R. C., Sankalia J. M., Sutariya V. B. Reversed chitosan–alginate polyelectrolyte complex for stability improvement of alpha-amylase: Optimization and physicochemical characterization. Eur. J. Pharm. Biopharm. 2007; 65: 215–232
- Siepmann J., Kranz H., Bodmeier R., Peppas N. A. HPMC matrices for controlled drug delivery: a new model combining diffusion, swelling and dissolution mechanisms and predicting the release kinetics. Pharm. Res. 1999; 16: 1748–1756
- Siepmann J., Peppas N. A. Modelling of drug release from delivery systems based on hydroxylpropyl methylcellulose (HPMC). Adv. Drug Deliv. Rev. 2001; 48: 139–157
- Sopprinath K. S., Aminabhavi T. M. Water transport and drug release study from cross-linked polyacrylamide grafted guar gum hydrogel microspheres for the controlled release application. Eur. J. Pharm. Biopharm. 2002; 53: 87–98
- Sousa J. J., Sousa A., Moura M. J., Podczeck F., Nowton J. M. The influence of core materials and film coating on the drug release from coated pellets. Int. J. Pharm. 2002; 233: 111–122
- Tahara K., Yamamoto K., Nishihata T. Overall mechanism behind matrix sustained release (SR) tablets prepared with hydroxylpropylmethylcellulose 2910. J. Control. Rel. 1995; 35: 59–66