Abstract
Two different types of cationized microspheres based on a native cationic gelatin (NGMS) and aminated gelatin with ethylendiamine (CGMS) were investigated for the controlled release of three model acidic peptide/protein drugs with different molecular weights (MWs) and isoelectric points (IEPs). Recombinant human (rh)-insulin (MW: 5.8 kDa, IEP: 5.3), bovine milk lactoalbumin, BMLA (MW: 14 kDa, IEP: 4.3), and bovine serum albumin (BSA MW: 67 kDa, IEP: 4.9) were used as model acidic peptide/protein drugs. The in vitro release profiles of these acidic peptide/protein drugs from NGMS and CGMS were compared and different periods of cross-linking were obtained. The slower release of these acidic peptide/protein drugs from CGMS compared with those from NGMS with cross-linking for 48 hr. was caused by the suppression of burst release during the initial phase. The degree of suppression of burst release of the three peptide/protein drugs during the initial phase by CGMS was in the following order: (rh)-insulin > BMLA > BSA. The release of insulin with a lower molecular weight from CGMS was particularly suppressed compared with the other two drugs with higher molecular weights in the initial phase. The control of the release rate of acidic peptide/protein drugs from gelatin microsphere can be achieved by amination of gelatin. Therefore, CGMS is useful for the controlled release of acidic peptide/ protein drugs.
Gelatin is a denatured, biocompatible, and biodegradable protein obtained from collagen and an excellent material for medical use. It has been used clinically as an excipient for drugs and implanted formulations (Tabata and Ikada Citation1998). Furthermore, its physicochemical characteristics can be modified by chemical treatment. Acidic and basic gelatins are denatured by the alkaline and acidic treatment of collagen, respectively. This treatment affects the electrical nature of the collagen, yielding a series of gelatins with different isoelectric points (IEP). Anionic and cationic gelatin microspheres were prepared using acidic gelatin (IEP = 5.0) and basic gelatin (IEP = 9.0), respectively.
Peptide/protein drugs have a relatively short half-life (t1/2) following injection and a poor bioavailability following oral administration. We have been investigating drug delivery systems to improve the bioavailability and controlled release of peptide/protein drugs using microspheres based on acidic gelatin and basic gelatin for a number of years (Tabata et al. Citation1999; Morimoto et al. Citation2000, Citation2001). We reported previously that the release profiles of protein/peptide drugs from anionic and cationic gelatin microspheres can be explained by an ionic interaction (Tabata et al. Citation1999). Therefore, an incorporated acidic peptide drug, such as bovine insulin (IEP = 5.3), with an IEP < 7.0, is released in a sustained manner from positively charged gelatin microspheres, whereas basic peptides, such as b-FGF (IEP = 9.6) and salmon calcitonin (IEP = 8.2), with an IEP > 7.0, are released initially from positively charged gelatin microspheres (Tabata et al. Citation1999; Morimoto et al. Citation2001). We also reported that gelatin microspheres enhance the bioavailability of calcitonin after pulmonary, nasal, and intramuacular administration in rats (Morimoto et al. Citation2000, Citation2001) and that the release rate of insulin from positively charged gelatin microspheres can be sustained by increasing the cross-linking density of gelatin under both in vitro and in vivo conditions (Iwanaga et al. Citation2003).
Furthermore, we reported the controlled release of 125I-plasmid DNA from a cationized hydrogel based on aminated gelatin with ethylendiamine (Fukunaka et al. Citation2002). When cationized hydrogels incorporating 125I-plasmid DNA were implanted into the femoral muscle of mice, the plasmid DNA radioactivity remaining decreased with time and the retention period was prolonged. We also described injectable cationized microspheres based on aminated gelatin with ethylendiamine to prolong the action of insulin in mice (Morimoto et al. Citation2005). We reported that cationized microspheres based on aminated gelatin with ethylendiamine were able to enhance the nasal delivery of insulin (Wang, Tabata, and Morimoto Citation2006).
In the present study, we prepared two different types of cationized microspheres based on a native cationic gelatin (NGMS) and aminated gelatin with ethylendiamine (CGMS) for controlled release of three acidic peptide/protein drugs with different molecular weights (MWs) and IEPs. The three model acidic peptide/protein drugs were recombinant human (rh)-insulin (MW: 5.8 kDa, IEP: 5.3), bovine milk lactoalbumin (BMLA, MW: 14 kDa, IEP: 4.3), and bovine serum albumin (BSA, MW: 67 kDa, IEP: 4.9). The in vitro release profiles of these three acidic peptide/protein drugs from NGMS and CGMS were compared. Furthermore, the in vitro release profiles of three acidic peptide/protein drugs from CGMS with different periods of cross-linking of gelatin were evaluated.
MATERIALS AND METHODS
Native cationic gelatin (IEP = 9.0; 100 kDa) was kindly supplied by Nitta Gelatin. (Osaka, Japan). Rh-insulin (insulin; 26.0 IU/mg) was purchased from Wako Pure Chem. (Osaka, Japan), BMLA and BSA were from Sigma Chemical. (St Louis, MO, USA). Also, 1, 2-Ethylenediamine and glycine were purchased from Kanto Chemical (Tokyo, Japan). Glutaraldehyde (25% in water solution) and 2, 4, 6-trinitrobenzenesulfonic acid sodium salt (TNBS) were purchased from Nakalai Tesque (Kyoto, Japan). 1-Ethyl-3-(3-dimethylaminopropyl)-carbodi- imide hydrochloride was purchased from Wako Pure Chem. All other chemicals were of reagent grade and used as received.
Synthesis of Aminated Gelatin
Aminated gelatin was synthesized by the reaction of native cationic gelatin with 1, 2-ethylenediamine (Wang et al. Citation2000). Briefly, native cationic gelatin was dissolved in phosphate buffer (pH 5.3), and 1, 2-ethylenediamine, was added under stirring. After adjusting the pH to 5.0 with 5 M HCl, 1-ethyl-3-(3-dimethyl-aminopropyl)-carbodiimide hydrochloride was added. The final concentrations of native gelatin, ethylenediamine, and 1-ethyl-3-(3-dimethyl-aminopropyl)-carbodiimide hydrochloride in the resultant solution were 20, 56, and 10.7 mg/mL, respectively. The resultant solution was incubated at 37°C for 18 hr, followed by dialysis against purified water for 48 hr, and then freeze-dried to obtain the aminated gelatin. The numbers of primary amino groups introduced into the native cationic and aminated gelatin were measured by the TNBS method (Snyder and Sobocinski Citation1975). The amino group contents of the native cationic gelatin and aminated gelatin were 0.33 and 0.64 mmol/g, respectively.
Preparation of Gelatin Microspheres
NGMS and CGMS were prepared by glutaraldehyde cross-linking in the dispersed state of an aqueous gelatin solution in the oil phase by a modification of the method of Tabata and Ikada (Citation1989). Briefly, 1 mL native cationic or aminated gelatin aqueous solution (10 wt%, preheated to 40°C) was added dropwise to 25 mL olive oil and then emulsified by vortex-mixing to yield a water-in-oil emulsion. The emulsion temperature was dropped to 4°C, then 7.5 mL cold acetone was added to the emulsion and stirring was continued for 1 hr to induce gel formation. The resulting microspheres were collected by centrifugation (2500× g, 4°C, 5 min) and washed three times with acetone. The cross-linking of the microspheres was carried out using 0.06% glutaraldehyde in acetone/0.01 M HCl solution (7:3) at 4°C for the indicated time. Following collection by centrifugation (2500× g, 4°C, 5 min), the microspheres were agitated in 10 mM aqueous glycine solution at room temperature for 1 hr to block the residual aldehyde groups of the unreacted glutaraldehyde. The resulting microspheres were finally washed three times with purified water by centrifugation and then freeze-dried.
Incorporation of RITC-Peptide/Protein Drugs into Gelatin Microspheres
Peptide/proteins were labeled with rhodamin B isothiocyanate (RITC-peptide/protein drugs) by the method reported by Mossberg and Ericsson (Citation1990) and incorporated into microspheres. Then, 20 μ L RITC-peptide/protein solutions (2.0 mg protein/mL; pH 7.4 phosphate-buffered saline; PBS) were added to the freeze-dried microspheres (NGMS and CGMS) (2.0 mg). Following this, they were left at 4°C for 5 hr to allow the solution to penetrate into the microspheres. Approximately 100% RITC-peptide/protein solution entered the microspheres during the swelling process because the solution volume was less than that which theoretically would impregnate the microspheres. The mean diameter of the microspheres (NGMS and CGMS) incorporating RITC-peptide/protein drugs under swelling conditions in pH 7.4 PBS was determined by microphotographs and was 29.7 ± 1.51 μ m regardless of the period of cross-linking.
Release Profiles of RITC-Peptide/Protein Drugs
The microspheres containing RITC-peptide/protein drugs were added to 1.5 mL PBS (pH 7.4), and this suspension was incubated at 37°C for up to 24 hr. The suspension was centrifuged at a predetermined time for 10,000 rpm and 1 mL supernatant was taken as a sample. The fluorescence intensity of each sample was determined by fluorospectrophotometry (Hitachi F-2000, Tokyo, Japan). The excitation and emission wavelengths were set at 554 and 578 nm, respectively.
Statistical Analysis
The mean values and their standard deviations (S.D.) were calculated in each experiment. Differences between NGMS and CGMS in the in vitro release of RITC-peptide/protein drugs were evaluated by the Mann Whitney U test. Statistical significance was taken as p < 0.05.
RESULTS
shows the microphotographs of CGMS with cross-linking reactions for 6 and 48 hr under swelling conditions in pH 7.4 PBS. CGMS under swelling conditions was spherical regardless of the cross-linking time, although a difference was observed in the surface structure depending on the cross-linking time.
FIG. 1 Microphotographs of CGMS with cross linking for 6 hr (A) and 48 hr (B) under swelling conditions in pH 7.4 PBS.
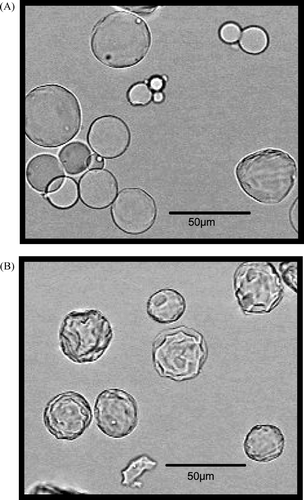
shows the release profiles of RITC-peptide/protein drugs (insulin [A], BMLA [B] and BSA [C]) from CGMS and NGMS with cross linking for 48 h in pH 7.4 PBS at 37°C in vitro. The time profiles of the release of these RITC-peptide/protein drugs from CGMS and NGMS were biphasic. RITC-peptide/protein drugs were released rapidly from NGMS in the initial phase within 2 hr; the amount released was ∼80% at 2 hr. In contrast, the release of three RITC-peptide/protein drugs from CGMS was suppressed significantly compared with that from NGMS. The released amounts of RITC-insulin, RITC-BMLA, and RITC-BSA from CGMS with cross-linking for 48 hr were ∼25%, 60% and 70%, respectively, at 6 hr and then leveled off. The initial release of RITC-insulin with a lower molecular weight from CGMS was particularly suppressed compared with RITC-BMLA and RITC-BSA with higher molecular weights. Therefore, the cationization of the microspheres based on aminated gelatin produces a sustained release of RITC-peptide/protein drugs from the gelatin microspheres.
FIG. 2 Release profiles of RITC-peptide/protein drugs—insulin (A), BMLA (B), and BSA (C)—from native gelatin microsphere (NGMS) and cationic gelatin microspheres (CGMS) with cross-linking for 48 hr in pH 7.4 PBS at 37°C in vitro. NGMS-(ˆ); CGMS-(•). Each value represents the mean ± S.D. of three experiments. In all panels, a significant difference was shown by the Mann Whitney U test (p < 0.05) for each time point.
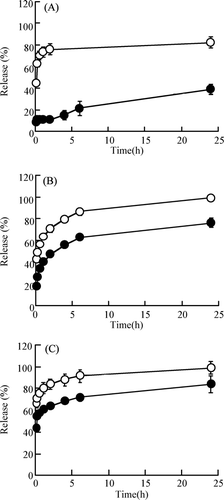
shows the release profiles of RITC-peptide/protein drugs (insulin [A], BMLA [B] and BSA [C]) from CGMS with various periods of cross linking for 6, 24, and 48 hr in pH 7.4 PBS at 37°C in vitro. The release of RITC-insulin from CGMS with cross-linking for 24 and 48 hr was suppressed to a greater degree compared with that of cross-linking for 6 hr. However, the release of RITC-BMLA and RITC-BSA from CGMS was not affected by the period of cross-linking for 6, 24, and 48 hr, respectively.
FIG. 3 Release profiles of RITC-peptide/protein drugs—insulin (A) BMLA (B), and BSA (C)—from cationic gelatin microspheres (CGMS) with cross-linking for 6, 24, 48, hr in pH 7.4 PBS at 37°C in vitro. Cross-linking for 6 (▪), 24 (▴), and 48 hr (•). Each value represents the mean ± S.D. of three experiments. In (A), a significant difference from cross-linking for 24 and 48 hr was shown by cross-linking for 6 hr by the Mann Whitney U test (p < 0.05) for each time point.
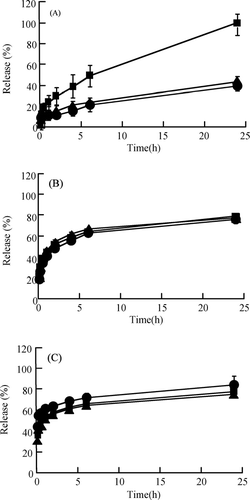
DISCUSSION
Microspheres composed of various kinds of materials have been prepared and used as carriers for the controlled release of peptide/protein drugs (Hahn et al. Citation2004; Leitner et al. Citation2004). Although the drug release rate from microspheres is generally difficult to control, it must be tightly controlled for safe therapy when microspheres are used as carriers for highly potent peptide/protein drugs. Among these carriers, gelatin microspheres are expected to be useful drug carriers because they are biodegradable as well as biocompatible (Nakaoka el al. Citation1996, Tabata and Ikada Citation1998). The cross-linking density, molecular weight, electrical charge, and biodegradation rate of gelatin affects the release rate of peptide/protein drugs from gelatin microspheres. (Tabata et al Citation1999; Morimoto et al Citation2001).
In this study, we investigated the relationship between the electrical charge of gelatin microsphere and the release profile of three acidic peptide/protein drugs, (rh)-insulin (MW: 5.8 kDa, IEP: 5.3) > BMLA (MW: 14 kDa, IEP: 4.3) > BSA (MW: 67 kDa, IEP: 4.9) with different MWs and IEPs using as model drugs. The slower release of these acidic peptide/protein drugs from CGMS compared with those from NGMS was caused by the suppressed burst release in the initial phase under in vitro nondegradation conditions without enzyme. This result suggests that the release of the three peptide/protein drugs from CGMS occurred through simple diffusion of these drugs in the microspheres, and this simple diffusion in CGMS may depend on the electrostatic interaction between these peptide/protein drugs and the aminated gelatin in CGMS.
Since these three peptide/protein drugs with an IEP > 6.0 were negatively charged in pH 7.4 PBS, an electrostatic interaction in CGMS would suppress their release. Furthermore, the cross-linking density in CGMS based on aminated gelatin with an amino group content of 0.64 mmol/g may be higher than that in NGMS based on native cationic gelatin with an amino group content of 0.33 mmol/g. The higher cross-linking density in CGMS may cause the suppressed releases of the three peptide/protein drugs.
The degree of suppression of burst release of the three peptide/protein drugs during the initial phase produced by CGMS was in the following order: (rh)-insulin > BMLA > BSA. The release of insulin with a lower molecular weight from CGMS was particularly suppressed compared with the other two drugs with higher molecular weights in the initial phase. The release of insulin from CGMS with a more prolonged period of cross-linking also was more highly suppressed. However, the release of BMLA and BSA from CGMS was not affected by more prolonged periods of cross linking. These results may be due to the localization of these incorporated peptide/protein molecules in CGMS. Insulin with a lower molecular weight may be incorporated into the internal regions of CGMS compared with those of BMLA and BSA with higher molecular weights. On the other hand, the release profiles of these peptide/protein drugs from CGMS in pH 7.4 PBS cannot be explain by the degree of negative charges of these peptide/protein drugs with different IEPs.
In conclusion, the control of the release rate of acidic peptide/protein drugs from gelatin microspheres can be achieved by amination of gelatin. Therefore, CGMS will be a useful method for the controlled release of acidic peptide/protein drugs.
REFERENCES
- Fukunaka Y., Iwanaga K., Morimoto K., Kakemi M., Tabata Y. Controlled release of plasmid DNA from cationized gelatin hydrogel based on hydrogel degradation. J. Control. Rel. 2002; 80: 333–343
- Hahn S. K., Kim S. J., Kim M. J., Kim D. H. Characterization and in vivo study of sustained-release formulation of human growth hormone using sodium hyaluronate. Pharm. Res. 2004; 21: 1374–1381
- Iwanaga K., Yabuta T., Kakemi M., Morimoto K., Tabata Y., Ikada Y. Usefulness of microspheres composed of gelatin with various cross-linking density. J. Microencapsul. 2003; 20: 767–776
- Leitner V. M., Guggi D., Krauland A. H., Bernkop-Schnurch A., Andreas B. S. Nasal delivery of human growth hormone: in vitro and in vivo evaluation of a thiomer/glutathione microparticulate delivery system. J. Control. Rel. 2004; 100: 87–95
- Morimoto K., Kstsumata H., Yabuta T., Iwanaga K., Kakemi M., Tabata Y., Ikada Y. Gelatin microspheres as a pulmonary delivery system: evaluation of salmon calcitonin absorption. J. Pharm. Pharmacol. 2000; 52: 611–617
- Morimoto K., Kstsumata H., Yabuta T., Iwanaga K., Kakemi M., Tabata Y., Ikada Y. Evaluation of gelatin microspheres for nasal and intramuscular administrations of salmon calcitonin. Eur. J. Pharm. Sci. 2001; 13: 179–185
- Morimoto K., Chono S., Kosai T., Seki T., Tabata Y. Design of novel injectable cationic microspheres based on aminated gelatin for prolonged action of insulin. J. Pharm. Pharmacol. 2005; 57: 839–844
- Mossberg K., Ericsson M. Detection of doubly stained fluorescent specimens using confocal microscopy. J. Microscopy 1990; 159: 215–224
- Nankaoka R., Inoue Y., Tabata Y., Ikada Y. Size effect on the antibody production induced by biodegradable microspheres containing antigen. Vaccine 1996; 13: 1251–1256
- Snyder S. L., Sobocinski P. Z. An improved 2,4,6-trinitro-benzensulfonic acid method for the determination of amines. Anal. Biochem. 1975; 64: 284–288
- Tabata Y., Ikada Y. Protein release from gelatin matrices. Adv. Drug Deliv. Rev. 1998; 31: 287–301
- Tabata Y., Ikada Morimoto K., Kstsumata H., Yabuta T., Iwanaga K., Kakemi T. Surfactant-free preparation of biodegradable hydrogel micro-spheres for protein release. J. Bioact. Compat. Polymers 1999; 14: 371–384
- Tabata Y., Ikada Y. Synthesis of gelatin microspheres containing interferon. Pharm. Res. 1989; 6: 422–427
- Wang J., Tauchi Y., Deguchi Y., Morimoto K., Tabata Y. Positively charged gelatin microsphere as gastric mucoadhesive drug delivery system for clearance of H. pylori. Drug Deliv. 2000; 7: 237–243
- Wang J., Tabata Y., Morimoto K. Aminated gelatin microspheres as a nasal delivery system for peptide drugs: evaluation of in vitro release and in vivo insulin absorption in rats. J. Control. Rel. 2006; 113: 31–37