Abstract
The elastic liposomes bearing acyclovir sodium were prepared for its enhanced transdermal delivery by conventional rotary evaporation method and characterized for various parameters such as vesicle shape and surface morphology, size and size distribution, entrapment efficiency, elasticity, polydispersity index, turbidity and in vitro release pattern. Permeability studies of acyclovir sodium incorporated in elastic liposomes were performed across artificial membranes and rat skin. Skin permeation potential of the developed formulation was assessed using confocal laser scanning microscopy, that revealed an enhanced permeation of the formulation to the deeper layers of the skin (up to 160 μm) following channel like pathways. Skin permeation profile of elastic liposomal formulation bearing acyclovir sodium was observed and the investigations revealed an enhanced transdermal flux (6.21 ± 1.8 μg/cm2/hr) and decreased lag time (0.6 hr) for acyclovir sodium. The obtained flux was nearly 2.0 and 6.3 times higher than conventional liposomal formulation bearing acyclovir sodium and plain drug solution, respectively (p < 0.01). The elastic liposomal formulation for transdermal delivery of acyclovir sodium provides better transdermal flux, higher entrapment efficiency, ability as a self-penetration enhancer and effectiveness for transdermal delivery as compared with conventional liposomes. In vivo studies showed that on transdermal application of elastic liposomes, the concentration of acyclovir sodium in plasma was found to be 105 ± 9.4 ng/ml after 24 hr which is about 4.2 times compared with conventional liposomes. Thus it is concluded that the elastic liposomes may be promising vehicles for the transdermal delivery of acyclovir sodium.
Acyclovir is being used in the treatment of infections including cutaneous herpes, genital herpes, chicken pox, varicella zoster infections, and herpes keratitis but available therapies have a number of limitations. The oral absorption is dose dependent and highly variable with a bioavailability ranging from 10 to 30% (Miranda and Blum Citation1983). Nevertheless, the oral route is preferred to parenteral administration because of the risk of local toxicity at the injection site. The mean plasma half life (t1/2) of acyclovir is 3.25 hr. Hence, repeated administration of high dose (200 mg; 5 times daily for 10 days) is required for the effective management of herpes simplex virus (HSV) infections (Bryson et al. Citation1983). On topical application the absorption of drug is very slow and needs a permeation enhancer, whereas bolus rapid injection causes renal precipitation of the drug. Furthermore, oral acyclovir has been associated with nausea, diarrhea, rash, or headache. Looking at these problems, an attempt has been made to develop a transdermal formulation of acyclovir sodium for prolonged systemic delivery.
To overcome the problem of poor skin permeability, several researchers have used on the elastic vesicles or transferosomes for transdermal delivery of insulin (Cevc et al. Citation1998), propranolol (Mishra et al. Citation2006), norgesterol (Jain et al. Citation2003b), and melatonin (Dubey et al. Citation2006). In recent years, there has been an increasing interest in the development of novel vesicular approaches for effective transdermal delivery. Vesicular carriers, i.e., elastic liposomes, conventional liposomes, and niosomes, elicit response by different mechanisms. Few of the lipids directly lower the skin permeability barrier that resides primarily in the stratum corneum. Hence specially designed lipid vesicles could be a better module for transcutaneous delivery of drugs. The most recent development in vesicle design for transcutaneous bioactive delivery is the use of elastic liposomes. This liposomes differ from conventional liposomes and niosomes by their characteristic fluid membrane with high elasticity. In elastic liposomes, elasticity is stress-controlled, owing to the composition dependence of the membrane bending energy (Cevc and Blume Citation1992).
Elastic liposomes passage through the normally confined pores of the stratum corneum is governed by the basic principles of elastomechanics (Cevc et al. Citation1996; Gompper and Kroll, Citation1995). This provides a rationale for self-optimizing and self-repairing bioactive carriers enabling elastic liposomes to squeeze themselves through intercellular regions of stratum corneum under the influence of transdermal water gradient (Paul et al. Citation1998; Cevc et al. Citation1995; Cevc Citation1996). The objectives of the present study were to design a transdermal delivery system of acyclovir sodium using elastic liposomes to overcome the problems associated with conventional therapies and to study in vivo effectiveness for sustained action. Also, the efficacy of elastic liposomes was compared with conventional liposomes in vivo.
MATERIALS AND METHODS
Acyclovir sodium was obtained as a generous gift sample from M/s Cipla Pharmaceticals, (Mumbai, India) Hydrogenated soya phosphatidyl choline (HSPC) was supplied as generous gift sample from LIPOID (Ludwinshafen, Germany). Cholesterol, Sephadex G-50, and Triton X-100 were procured from Sigma Chemical Co. (St. Louis, MO, USA). Span 40, 60, and 80 were purchased from Himedia (Mumbai, India). Rhodamine red-X 1,2-dihexadecanoyl-sn-glycero-3-phosphoethanolamine trimethylammonium salt (RR) was purchased from Molecular Probes (Eugene, OR, USA). Ethanol, chloroform, and xylene were procured from M/s E. Merck (Goa, India). Cellophane membrane (molecular weight cut off, 12000–14000 dalton) was procured from Himedia. All other reagents used in this study were of analytical grade. Double distilled water was used throughout the experiments.
Preparation of Elastic Liposomes
The elastic liposomes were prepared by conventional rotary evaporation method reported by Cevc et al. (Citation1995) with little modifications. Briefly, hydrogenated soya phosphatidyl choline and surfactant (Span 40/60/80) were taken in a round bottom flask and the lipid mixture was dissolved in chloroform:methanol mixture (2:1 v/v). The thin film is casted by evaporating the solvent under reduced pressure in a rotary evaporator (Buchi-type, York Sci. Co., Mumbai, India) and final traces of solvent were removed under vacuum overnight. The dried lipid film was hydrated with saline phosphate buffer PBS (pH 6.5, 0.1ml/mg lipid) containing 1 mg/ml acyclovir sodium at 50–55°C followed by continuous vortexing of the flask for another 1 hr to get multilamellar vesicles. These vesicles were then sonicated by probe sonicator (Soniweld, India) for 5 min at 50–55°C to get the unilamellar vesicles. The sonicated vesicles were extruded through a sandwitch of 100 and 200 nm polycarbonate membranes (Millipore; Sigma Chemicals). To remove any unencapsulated drug, each liposomal preparation was eluted through a chilled Sephadex G-50 minicolumn by centrifugation at 300 g for 3 min. Similarly Rhodamine Red-(0.03% w/v) loaded elastic liposomes were prepared.
Preparation of Conventional Liposomes
Liposomes (control) were prepared by the cast film method (Gupta et al. Citation2007a), which is similar to the method reported for elastic liposomes preparation. Only, surfactants (Span 40/60/80) are replaced with cholesterol keeping PC:CH molar ratio: 7:3 and cholesterol (7:3 molar ratio) were dissolved in minimum quantity of chloroform:methanol mixture (2:1) in a round-bottom flask and thin film casted by evaporating the solvent under reduced pressure in a rotary evaporator (Buchi-type, York Sci. Co.). Final traces of solvent were removed under vacuum overnight. The dried lipid film was hydrated with saline phosphate buffer PBS (pH 6.5, 0.1 ml/mg lipid) containing 1 mg/ml acyclovir sodium at 50–55°C followed by continuous vortexing of the flask for an addition hour to get multilamellar vesicles. These were then sonicated by probe sonicator (Soniweld, India) for 5 min at 50–55°C to get the unilamellar vesicles. The sonicated vesicles were extruded through a sandwich of 100 and 200 nm polycarbonate membranes (Millipore; Sigma Chemicals Co.). To remove any unencapsulated drug, each liposomal preparation was eluted through a chilled Sephadex G-50 minicolumn by centrifugation at 300 g for 3 minutes.
Vesicle Morphology and Size Analysis
Elastic liposomal formulations were visualized under transmission electron microscope (Philips CM12, Eindhoven, Netherlands), with an accelerating voltage of 100 kV. A drop of the sample was placed onto a carbon-coated copper grid to leave a thin film. Before the film dried on the grid, it was negatively stained with 1% phosphotungastic acid. A drop of the staining solution was added onto the film and excess of the solution was drained off with a filter paper. The grid was allowed to dry thoroughly in air and samples were viewed under a transmission electron microscope. The vesicles size was determined by dynamic light scattering method (DLS) in a multimodal mode using a computerized inspection system (Malvern Zetamaster, ZEM 5002, Malvern, UK). For vesicles size measurement, vesicular suspension was mixed with the appropriate medium (PBS pH 6.5) and the measurements were conducted in triplicate.
Entrapment Efficiency
For determination of entrapment efficiency, the unentrapped drug in elastic liposomal formulation was separated using the Sephadex G-50 minicolumn centrifugation method (Fry et al. Citation1978; Sorensen et al. Citation1977; Gupta et al. Citation2007b). The separated liposomes were than distrupted using 0.1% Triton X-100 and analyzed for drug content at 254 nm after suitable dilutions using GBC spectrophotometer.
Turbidity and Elasticity Measurements
The turbidity of different elastic liposomal formulations was determined against PBS (pH 6.5) as blank using Nephelometer (Superfit, Mumbai, India).
Elasticity measurement was performed according to the method reported by Vanden Bergh et al. (Citation2001). Briefly, vesicles were extruded through the polycarbonate filter (Millipore, USA) of 50 nm pore diameter using a stainless steel pressure holder of 25 mm diameter filters with 200 ml capacity barrel at 2.5 bar pressure for 10 min. Vesicular size and volume of extruded suspension were compared before and after the extrusion. The experiments were repeated three times.
Storage-Physical Stability of Elastic Liposomes
The ability of vesicles to retain the drug was assessed by keeping the elastic liposomal suspensions in sealed vials (10 ml capacity) after flushing with nitrogen at different time periods (10, 20, 40, 60, 80 and 120 days). Samples were withdrawn periodically and analyzed for residual drug content. The initial drug content was considered as 100%.
In vitro Skin Permeation Studies
The permeation of acyclovir sodium from the elastic liposomes through hairless rat skin was determined using Franz-diffusion cell (Crown Glass Co. NJ, USA) (Jain et al. Citation2005). Hairless rat abdominal skin was clamped between the donor and receptor compartment of diffusion cell (1 cm2). The membrane was carefully mounted onto the diffusion cell with the stratum corneum side facing the donor compartment and dermal side bathed in receptor media. The elastic liposomes and conventional liposomes bearing acyclovir sodium were placed into the donor compartment over the skin, while the receptor compartment (10 ml) was filled with phosphate buffer saline (PBS, pH 6.5) containing 20% PEG 400.
Temperature of the cell assembly was maintained at 37± 1°C and contents of the receptor compartment were stirred using a magnetic stirrer. Samples (1 ml each) were collected from receptor compartment periodically for 24 hr and the cell was refilled immediately with equal volumes of fresh media. The amount of drug permeated was determined by HPLC method (Boulieu et al. Citation1997). The cumulative amount of drug permeated per unit area was plotted as a function of time, the steady-state permeation rate (Jss), and lag time (LT, h) were calculated from the slope and X-intercept of the linear portion, respectively. The enhancement ratio (ER) was calculated from following equation
Confocal Laser Scanning Microscopy
Depth and mechanism of skin penetration of RR-loaded elastic liposomes and conventional liposmes was investigated using confocal laser scanning microscopy (CLSM). The probe-loaded vesicles were first passed through the Sephadex G-50 mini-column to separate the unentrapped probe. Thereafter formulation was applied nonocculsively for 8 hr to the dorsal skin of 5–6-week, old nude albino rat (Sprague–Dawley strain). Treated animals were kept in a separate cage and maintained under laboratory conditions. Food and water were allowed ad libitum. All investigations were performed as approved by the Institutional ethical committee of Dr. H. S. Gour University, Sagar, India.
The rat was sacrificed by heart puncture; dorsal skin was excised and washed with distilled water. The excised skin was then placed on aluminium foil and the dermal side of the skin was gently teased off to remove any adhering fat and/or subcutaneous tissue. The skin was sectioned into pieces of 1 mm2 size each and evaluated for depth of probe penetration. The full skin thickness was optically scanned at different increments through the z-axis of a CLS microscope (LSM 510 with an attached universal Zeiss epifluoroscence microscope). Optical excitations were carried out at 488 nm using Argon laser beam and fluorescence emission was detected at 560 nm for RR.
Plasma Concentration Studies
Eighteen albino rats (Sprague–Dawley strain), 5–6 weeks old, weighing 80–100 g were divided into 3 groups having 6 rats in each group. First group served as control, received the topical application of plain drug solution, second and third groups received elastic and conventional liposomal formulations bearing acyclovir sodium, respectively. Fully 200 μl of all formulations were applied at the dorsal side of rats to the area of 1.0 cm2. At specific time intervals rats were sacrificed and blood was collected from cardiac puncture in heparinized centrifuge tubes. The collected blood samples were centrifuged at 5000 rpm for 8 min. Serum was harvested from supernatant. To 150 μl of serum, equal volume of 10% (v/v) aqueous trichloroacetic acid was added and mixed by vortexing for 30 sec. The mixture was then centrifuged at 5000 rpm for 5 min and supernatant was filtered through 0.45 μm membrane filter. Next, 50 μl aliquot was injected to HPLC column (Boulieu et al. Citation1997).
The HPLC system (Shimadzu LC10 Ai, Chiyoda-ku, Tokyo, Japan) was operated in a binary mode with a photodiode array detector and a communication bus module. The analysis was performed at 254 nm with a shimpack, reverse phase C18, 250 mm × 34.5 mm × 5 mm column maintained at 25°C (column oven) using a mobile phase of 0.02 M potassium dihydrogen phosphate, pH 3.5 (99%), and acetonitrile (1%) pumped at a flow rate of 1.5 mL/min. The retention time of the drug was found to be 7.8 ± 0.1 min.
Statistical Analysis
Data are expressed as the mean standard deviation (SD) of the mean and statistical analysis was carried out employing analysis of variance (ANOVA). Differences were considered statistically significant at p < 0.01.
RESULTS AND DISCUSSION
Preparation and Characterization of Elastic Liposomes
Elastic liposomes were prepared using the rotary evaporation method reported by Cevc et al. (Citation1995) using lipid and surfactant. Transmission electron microscopy was performed to study the shape and lamellarity of the vesicles that revealed the elastic liposomes are unilamellar and spherical in shape (). The mean vesicle size, encapsulation efficiency, turbidity, and polydispersity index of elastic liposomes bearing acyclovir sodium are presented in . The mean diameter of acyclovir sodium loaded elastic liposomal formulations was found to range 96 ± 2.0–155 ± 1.3 nm. A low polydispersity index of less than 0.1 was obtained for all the formulations and indicated a narrow size distribution of the elastic liposomal suspension and consequently a homogeneous distribution ().
TABLE 1 Composition and characterization of elastic liposomal formulations
Entrapment efficiency is the percentage fraction of the total drug incorporated into the elastic liposomes. The maximum entrapment efficiency obtained was 66.4 ± 0.42% for acyclovir sodium loaded elastic liposomal formulation (TF-S803). It is observed that with increased surfactant concentration in the lipid components of the vesicles, the entrapment efficiency of the acyclovir sodium decreased (). This is due to the possible coexistence of mixed micelles and vesicles at higher concentration of surfactant, with the consequence of lower drug entrapment in mixed micelles. The formation of micellar structure at higher concentration of surfactant is an established fact. The studies by Lasch et al. (Citation1990) and Lopez et al. (Citation1998) prove this fact.
A unique property associated with elastic liposomes is their deformability. This property in combination with sensitivity of elastic liposomes to water gradient across the skin makes this carrier a potential system for topical drug delivery. The horny region of the skin is associated with sparsely distributed pores. These pores act as permeability shunt and locally lower the skin permeability barrier. Further, these pores are potential sites for deformable bodies, which are strongly driven under the effect of transepidermal water gradient.
The main feature of elastic liposomal formulations in comparison with the conventional liposomes and other types of the drug-loaded lipid suspensions is the flexibility of the vesicle membrane and stress-dependent adaptability. The elasticity value for acyclovir sodium loaded elastic liposomal formulations were found to be 7.77 ± 1.2 to 39.33 ± 3.6 (). The results indicate that elasticity of vesicles depends on both surfactant concentration and type. With increase in the surfactant concentration from 5 to 15% w/w, the elasticity value increases from 27.18 ± 3.2 to 39.33 ± 3.6 for formulation TF-S801 and TF-S803 respectively. But with further increase in surfactant concentration, elasticity value of vesicle membranes decreases significantly (from 39.33 ± 3.6 to 9.98 ± 0.9 for formulation TF-S803 and TF-S805 respectively) (p < 0.01). This behavior may be attributed to the formation of mixed micelles at higher concentration of surfactant.
Turbidity of different formulations was determined employing nephalometer. Formulations containing Span-80 exhibited higher values of turbidity, indicating good population of vesicles, in general. Lower values of the turbidity are indicative of the fact that the vesicles might have ruptured or were converted into other structures (). The results of turbidity studies are concordant with Mishra et al. (Citation2006).
Stability (in process as well as on storage) is an important parameter. The formulation stored at 4± 1°C demonstrated a very low drug leakage as compared with formulations stored at 25± 1°C after 120 days storage, suggesting that the refrigerated conditions are well-suited for the developed formulation.
Confocal Laser Microscopy
The CLSM study revealed the characteristic of the formulation supporting the permeation of acyclovir sodium-loaded elastic liposomes to deeper layers of the skin (). RR-loaded elastic liposomal system shows higher depth of penetration (up to 160 μm) and fluorescence intensity 165 AU, whereas conventional liposomes remained confined to few micrometers only (50 μm) and fluorescence intensity 35 AU. As seen from some channel-like pathways in the stratum corneum can be observed. These channel-like structures were highly stained, confirming their role as a permeability shunt, which locally lowers the permeability barrier. These observations of penetration pathways in the form of channels resembled the reports of Van-den Bergh et al. (Citation1999) and Honeywell-Nguyen et al. (Citation2002).
In vitro Skin Permeation Studies
The in vitro skin permeation study was performed using hairless rat skin, and slower skin permeation was observed with respect to the tests with artificial membrane. This was expected, as a consequence of the stronger barrier effect due to the presence of the stratum corneum. Moreover, the same trend was observed as for the drug amount permeated from elastic liposomal formulations bearing acyclovir sodium. The in vitro drug release across the rat skin exhibited 4.93 ± 1.4% drug release in 8 hr that increased to 11.23 ± 0.6% in 24 hr in case of free drug; it was 12.97 ± 0.4% in 8 hr and 21.55 ± 1.6% in 24 hr in acyclovir sodium loaded conventional liposomes. The drug release was further enhanced, i.e., 24.43 ± 1.6 and 39.74 ± 2.3% in 8 hr and 24 hr, respectively, from acyclovir sodium entrapped in elastic liposomes (). The increase in drug release was due to the ultraflexible nature of the elastic liposomes.
FIG. 3 Percentage cumulative drug release from elastic liposomal formulation, conventional liposomal formulation, and plain drug solution through albino rat skin (mean ± S.E., n = 3).
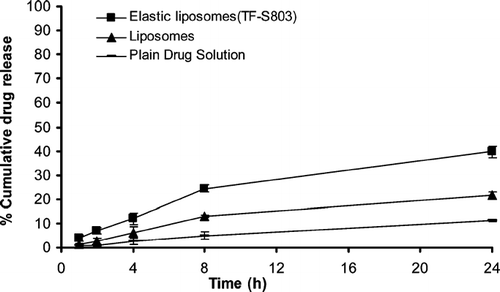
The value of steady-state transdermal flux for different elastic liposomal formulations were observed between 5.73 ± 2.1 and 6.21 ± 1.8 μg/cm2/hr. The maximum flux obtained from TF-S803 was substantially 6.3 times higher than that of plain drug (0.98 ± 1.7 μg/cm2/hr) and nearly 2 times higher than conventional liposomal formulation (3.12 ± 2.1 μg/cm2/hr). Also, a minimum lag time (0.6 hr) was obtained with TF-S803 formulation (). Better transdermal flux and lower lag phase with our formulation was perhaps due to combination of one or more of following mechanisms: (1) increase in thermodynamic activity, (2) increased skin vehicle partitioning of drug, (3) altering the barrier properties by interacting with skin component, and (4) elasticity of vesicle membrane.
TABLE 2 Permeation parameters of acyclovir-loaded formulations across rat skin after 24 hours
The use of elastic liposomes for noninvasive drug delivery is widely reported. Better skin penetration ability of these elastic liposomes is well supported by many authors; Honeywell-Ngoyen et al. (Citation2003) reported the enhanced delivery of pergolide using elastic liposomes; El Maghraby et al. (Citation2000) and El Maghraby et al. (Citation2001) reported better skin permeation ability of elastic liposomes using estradiol and 5-fluorouracil as a model drug; Trotta et al. (Citation2002) reported the elastic liposomes for skin delivery of dipotassium glycyrrhizinate, while Guo et al. (Citation2000) reported the elastic liposomes for systemic delivery of cyclosporin A.
Drug Plasma Profile
The in vivo study of different formulations was carried out by means of plasma drug concentration measurement. Three formulations: plain drug solution, drug-in-conventional liposomes, and drug-in-elastic liposomes were applied on back of the rat skin. The blood concentration was determined for 24 hr. The elastic liposomal formulation-bearing drug and conventional liposomal formulation bearing drug showed the blood level concentration 105 ± 9.4 ng/ml, and 25 ± 1.9 ng/ml, respectively, after 24 hr whereas no drug was found in blood after 24 hr on administration of plain drug solution (). Elastic liposomal formulation-bearing drug showed nearly 4.2 times better blood level concentration than conventional liposomes representing greater permeation.
FIG. 4 Drug plasma concentration profile after transdermal application of different formulations (mean ± S.E. n = 6).
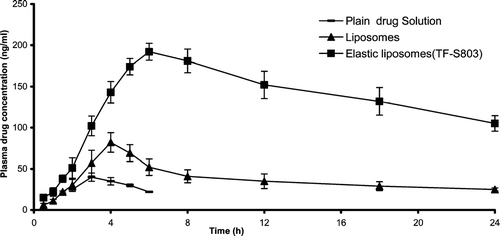
Plasma levels of acyclovir sodium after application of control were low (Cmax. ≈ 40±5.1 ng/ml), whereas those after application of the elastic liposomal formulation bearing acyclovir sodium gradually increased and reached a peak level of 192± 10.3 ng/ml at 6 hr postadministration and maintained for longer times. The area under the curve (AUC0−24h) was found to be 3456±117 ng.h/ml and was much larger than 482 ± 25 ng.h/mL being AUC0−24h of control (). The conventional liposomal formulation bearing acyclovir sodium also showed higher drug level (1394 ± 41 ng.h/ml) in comparison to control, yet lower compared with elastic liposomal formulation bearing acyclovir sodium complex.
TABLE 3 Pharmacokinetic parameters of acyclovir
The results of the present investigation showed that the problems associated with the transdermal delivery of acyclovir sodium could be overcome incorporating it into the new ultraflexible drug carrier, elastic liposomes. Elastic liposomes are specially optimized vesicles that can respond to an external stress by rapid and energetically inexpensive shape transformations. Elastic liposomes differ from conventional gel state niosomes and liposomes by their characteristic fluid membrane with high elasticity. The elasticity of these vesicles is attributed to the simultaneous presence of different stabilizing (phospholipids) and destabilizing (surfactant) molecules and their tendency to redistribute in bilayers. Such highly deformable vesicles can thus be used to bring drugs across the biological permeability barriers such as skin.
CONCLUSION
Skin permeation profile of acyclovir sodium through elastic liposomes formulations was observed in the present study. The investigations revealed an enhanced transdermal flux and decreased lag time of acyclovir sodium. Hence, it could be concluded that elastic liposomes may be suitable carrier for transdermal delivery of acyclovir sodium.
The authors extend their gratitude to M/s Lipoid, GmbH, Germany, for supplying HSPC as a gift sample. Yashwant Gupta and Anekant Jain are thankful to the Council of Scientific and Industrial Research and Indian Council of Medical Research, New Delhi, India, for providing financial assistance (SRF). The authors also are grateful to the All India Institute of Medical Sciences, New Delhi, for providing transmission electron microscopy facility.
REFERENCES
- Boulieu R., Gallant C., Silberstein N. Determination of acyclovir in human serum by high-performance liquid chromatography. J. Chromat. 1997; 693: 233–236
- Bryson Y. J., Dillon M., Lovett M. Treatment of first episodes of genital herpes simplex virus infection with oral acyclovir. A randomized double-blind controlled trial in normal subjects. N. Engl. J. Med. 1983; 308: 916–921
- Cevc G., Gebauer D., Stieber J., Schatzlein A., Blume G. Ultraflexible vesicles, elastic liposomes, have an extremely low pore penetration resistance and transport therapeutic of insulin across the intact mammalian skin. Biochim. Biophys. Acta 1998; 1368: 201–215
- Cevc G. Lipid suspensions on the skin. Penetration enhancement, vesicle penetration and transdermal drug delivery. Crit. Rev. Ther. Drug. Carrier Syst. 1996; 13: 257–88
- Cevc G., Blume G. Lipid vesicles penetrate into intact skin owing to the transdermal osmotic gradients and hydration force. Biochim. Biophys. Acta 1992; 1104: 226–232
- Cevc G., Blume G., Schatzlein A., Gebauer D., Paul A. The skin: a pathway for the systemic treatment with patches and lipid-based agent carriers. Adv. Drug Deliv. Rev. 1996; 18: 349–378
- Cevc G., Schatzlein A., Blume G. Transdermal drug carriers: basic properties, optimization and transfer-efficiency in the case of epicutaneously applied peptides. J. Contr. Rel. 1995; 36: 3–16
- Dubey V., Mishra D., Asthana A., Jain N. K. Transdermal delivery of a pineal hormone: Melatonin via elastic liposomes. Biomaterials 2006; 27: 3491–3496
- El Maghraby G. M. M., Williams A. C., Barry B. W. Oestradiol skin delivery from ultradeformable liposomes, refinement of surfactant concentration. Int. J. Pharm. 2000; 196: 63–74
- El Maghraby G. M. M., Williams A. C., Barry B. W. Skin delivery of 5-Fluorouracil from ultradeformable and standard liposomes. J. Pharm. Pharmacol. 2001; 53: 1069–1076
- Fry D. W., White J. C., Goldman I. D. Rapid separation of low molecular weight solutes from liposomes without dilution. J Anal Biochem. 1978; 90: 809–815
- Gompper G., Kroll D. M. Driven transport of fluid vesicles through narrow pores. Phys. Rev. 1995; 52: 4198–208
- Guo J., Ping G., Sun G., Jiao C. Lecithin vesicular carrier for transdermal delivery of cyclosporine A. Int. J. Pharm. 2000; 194: 201–207
- Gupta Y., Jain A., Jain P., Jain S. K. Design and development of folate appended liposomes for enhanced delivery of 5-FU to tumour cells. J. Drug Target 2007a; 153: 231–240
- Gupta Y., Jain A., Jain S. K. Transferrin conjugated solid lipid nanoparticles for enhanced delivery of quinine dihydrochloride to the brain. J. Pharm Pharmacol 2007b; 59: 935–940
- Honeywell-Nguyen P. I., DeGraff A. M., Groenink H. W. W., Bowstra J. A. The in vivo and in vitro interactions of elastic and rigid vesicles with human skin. Biochim. Biophys. Acta 2002; 1573: 130–140
- Honeywell-Nguyen P. I., Frederik P. M., Bomans P. H. H., Junginger H. E., Bouwstra J. A. The in vitro transport of pergolide from surfactant based elastic vesicles though human skin: A suggested mechanism of action. J. Contr. Rel. 2003; 86: 145–156
- Jain S., Jain P., Jain N. K. Transfersomes: a novel vesicular carrier for enhanced transdermal delivery: development, characterization and performance evaluation. Drug Dev. Ind. Pharm. 2003a; 29: 1013–1026
- Jain S., Sapre R., Umamaheshwari R. B., Jain N. K. Protransfersomes for effective transdermal delivery of norgestrel preparation and in vitro characterization. Ind. J. Pharm. Sci. 2003; 65: 153–161
- Jain S. K., Chourasia M. K., Masuriha R., Soni V., Jain A, Jain N. K., Gupta Y. Solid lipoid nanoparticles bearing flurbiprofen for transdermal delivery. Drug Deliv 2005; 12: 207–215
- Lasch J., Hoffman J., Amelyaneenka W. G., Klibanov A. A., Torchilin V. P., Binder H., Gawrisch K. Interaction of Triton X-100 and Octyl glycoside with liposomal membranes at sublytic and lytic concentration: spectroscopic studies. Biochim Biophys Acta 1990; 1022: 171–180
- Lopez C., Maza A., Coderch L., Lpez Iglesias L., Wehli E., Parra J. L. Direct formation of mixed micelles in the solubilization of phospholipids liposome by Triton X-100. FEBS Lett. 1998; 426: 314–318
- Miranda P. D., Blum M. R. Pharmacokinetics of acyclovir after intravenous and oral administration. Antimicrob. Chemother 1983; 12: 29–37
- Mishra D., Garg M., Dubey V., Jain S., Jain N. K. Elastic liposomes mediated transdermal delivery of an antihypertensive agant: Propranolol hydrochloride. J. Phar. Sci. 2006; 96: 145–155
- Paul A., Cevc G., Bachhawat B. K. Transdermal immunization with an integral membrane component, gap junction protein, by means of ultradeformable drug carriers, transfersomes. Vaccine 1998; 16: 188–195
- Sorensen E. N., Weisman G., Vidaver G. A. A sephadex column for measuring uptake and loss of low molecular weight solutes from small vesicles. Anal. Biochem. 1977; 82: 376–384
- Trotta M., Peira E., Debernadi F., Gallarate M. Elastic liposomes for skin delivery of potassium glycyrrhizinate. Int. J. Pharm. 2002; 241: 319–327
- Vanden bergh B. A. I., Vroom J., Gerritsen H., Junginger H. E., Bowstra J. A. Interactions of elastic and rigid vesicles with human skin in vitro: electron microscopy and two-photon excitation microscopy. Biochim. Biophys. Acta 1999; 146: 155–173
- Vanden Bergh B. A. I., Wertz P. W., Junginger H. E., Bouwstra J. A. Elasticity of vesicles assessed by electron spin resonance, electron microscopy, and extrusion measurement. Int. J. Pharm. 2001; 217: 13–24