Abstract
This study evaluated three chitosan-N-acetyl cysteine (CAC) conjugates of increasing molecular mass as a valuable tool to improve the absorption of drugs by assessing its permeation enhancing effect regarding the active P-gp substrate rhodamine-123 in comparison to the trans- and paracellular marker FD 4 both in rat intestine and Caco 2 monolayers. Additional LDH and MTT cytotoxicity tests have attested a non-toxic profile to CAC, which can consequently be seen as a safe and promising novel drug carrier with the ability to enhance drug absorption and to inhibit P-gp efflux transporters.
INTRODUCTION
The bioavailability of many orally administered drugs is often limited by insufficient paracellular absorption from the gastrointestinal tract. This absorption barrier is formed by epithelial cells, which are interconnected by tight junctions (Zhou and Po Citation1991). The latter are small membrane proteins located on the apical domain of the cell membrane where they accomplish a gate function. In recent years, a large number of permeation enhancers, influencing the opening mechanism of tight junctions, have been identified in order to increase the oral bioavailability of various drugs (Guggi and Bernkop-Schnürch Citation2005). Chitosan and polyacrylates have received a lot of attention, however, thiolated polymers have shown to further improve the permeation enhancing effect while remaining concentrated at the area of drug absorption due to distinct mucoadhesive properties (Bernkop-Schnürch et al. Citation2003). Clausen and colleagues (Citation2002) were able to demonstrate that sulfhydryl groups immobilized on common polymers are responsible for the significantly enhanced paracellular transport by interfering with the permeation mediator glutathione.
Surprisingly, former studies showed that thiolated polymeric excipients are also able to interfere with the main active efflux transporter P-glycoprotein (P-gp), expressed on the apical membrane of intestinal epithelia in humans and rodents (Ho et al. Citation2003). The effectiveness of chitosan-thiobutylamidine on the P-gp activity was assessed both in vitro and in vivo, revealing a strongly improved transmucosal transport of the P-gp substrate rhodamine-123 in presence of thiolated chitosan, as a result of P-gp efflux pump inhibition (Werle and Hoffer Citation2005). Due to their high molecular mass, polymeric excipients are not absorbed after oral administration. Thus systemic toxic side effects can be excluded. Polymeric efflux inhibitors combine both the ability to act as drug carrier and to control the fate of drugs by affecting absorption, distribution, and elimination processes. So far, polyoxylates, pluronic block copolymers, dendrimers and polysaccharides have been identified as effective polymeric efflux inhibitors (Hugger et al. Citation2002; Kabanov et al. Citation2002; D'Emanuele et al. Citation2004).
Based on these findings, it was the aim of this study to investigate the permeation-enhancing and P-gp inhibitory effect of chitosan-N-acetyl cysteine conjugates. Rhodamine-123 (Rho-123) used as specific P-gp substrate was compared with fluoresceine-labeled dextran (FD 4). Three chitosans differing by their molecular mass were chosen to evaluate a possible influence of the molecular mass on the transepithelial transport in the Ussing chamber, while the P-gp inhibitory effect of the most promising candidate was additionally investigated in Caco 2 monolayers. The cytotoxicity potential of the proposed polymeric drug carrier was assayed by LDH and MTT test, to fulfill the requirements posed by the strategic design of non-toxic P-pg inhibiting polymeric excipients.
MATERIALS AND METHODS
Materials
Chitosan 150 kDa (low molecular mass, < 200 mPa · s), 400 kDa (middle molecular mass, 200–400 mPa · s) and 600 kDa (high molecular mass, > 400 mPa · s) was obtained from Fluka (Vienna, Austria). N-Acetyl-L-cysteine (puriss > 99%) was purchased from Fluka (Vienna, Austria). Rhodamine-123 (Rho-123) was purchased from Acros Organics (Geel, Belgium). All other chemicals, if not specified separately, were purchased from Sigma–Aldrich (Vienna, Austria). All culture media were obtained from Sigma-Aldrich (Vienna, Austria). Fetal calf serum was purchased from Invitrogen (Lofer, Austria). Ninety-six-well microtiter plates and Corning Costar Transwell-Clear® filters were purchased from Szabo-Scandic (Vienna, Austria).
All salts and chemicals were of analytical grade unless otherwise stated.
Methods
Synthesis and Characterization of Chitosan-N-acetyl cysteine Conjugates
Chitosan-N-acetyl cysteine conjugates were synthesized by a previously described method using three chitosans, differing by their viscosity. In brief, 500 mg of chitosan were dissolved in 1% aqueous hydrochloric acid and adjusted to pH 5 with 1 M NaOH and 4 grams of N-acetyl cysteine were dissolved in demineralised water. The carboxylic acid moieties of N-acetyl cysteine were activated for 20 min by the addition of 1-ethyl-3-(3-dimethylaminopropyl) carbodiimide hydrochloride (EDAC). The pH of the solution adjusted within the range of 4 to 5, was maintained during the whole experiment. Both reagents were mixed and incubated for 6 h under permanent stirring at room temperature. The resulting chitosan-N-actyl cysteine conjugate was isolated in the dark by dialyzing at 10°C, as described previously. Samples were lyophilized at −78°C and 0.08 bar (Virtis Benchtop Freeze Dryer, Bartelt, Graz, Austria).
The amount of free thiol groups on modified chitosan conjugates were quantified photometrically with Ellman's reagent. The total amount of sulfhydryl groups fixed on the conjugates is represented by the summation of reduced thiol groups and oxidized thiol moieties in form of disulfide bonds. The latest were measured after reduction with sodium borohydride. Both tests were performed according to test procedures described previously by our group (Bernkop-Schnürch et al. Citation2004).
Cell Culture
The human colon cancer cell line Caco 2 was studied between passages 80 and 90. Caco 2 cells were maintained at 37°C in an atmosphere of 95% O2, 5% CO2 and 90% relative humidity in minimum essential medium (MEM) supplemented with 20% (v/v) fetal calf serum, 26 mM NaHCO3, 200 mM L-glutamine, penicilline (100 IU/ml) and streptomycin (100 μ g/ml).
Caco 2 cells were seeded on 75 cm2 plastic flasks (Corning Costar, Szabo Scandic, Vienna, Austria) by 0.5 × 106 cells, and the medium was completely changed every other day with modified Eagle's medium (MEM) supplemented with 20% of fetal calf serum. Caco 2 reached confluence at 7 d. Before use, cells were seeded on 12-well microtiter plates for cytotoxicity studies and incubated for 24 h. For transport studies, 1.5 × 106 cells were seeded on 12 mm diameter Transwell-Clear® dishes. The cells were differentiated for 21 d in the complete medium supplemented with penicillin (100 IU/ml) and streptomycin (100 μ g/ml). Medium was changed every second day. Transepithelial electrical resistance (TEER) measurements were performed to ensure that cells reached confluence.
In Vitro Permeation Studies
Modified Ussing-type chambers were used to simulate the in vitro permeation through gastrointestinal tissue. For permeation studies, non-fasting rats weighing 250 to 300 g were used. After sacrifice, the ileum was immediately excised. The tissue was cut into small strips and luminal contents were removed. Then the tissue was mounted in the modified Ussing chamber and preincubated for 20 min with artificial intestinal fluid decribed below. Prior to the experiment, both donor and acceptor chamber, displaying a permeation area of 0.64 cm2, were filled with a freshly prepared incubation medium containing 250 mM NaCl, 2.6 mM MgSO4, 10 mM KCl, 40 mM glucose and 50 mM NaHCO3 buffered with 40 mM HEPES, pH 6.8 to mimic the intestinal fluid. All experiments were performed in an atmosphere of 95% O2 and 5% CO2 to ensure oxygenation and agitation. The temperature within the chambers was maintained at 37 ± 1°C. The corresponding CAC conjugates (0.25% w/v) were dissolved and allowed to swell at 37°C. Rhodamine-123 (Rho-123) and fluoresceinisothiocyanate dextran 4 (FD 4), respectively, were used as model compounds in a final concentration of 0.001% (w/v). These concentrations were added either to the apical compartment for absorptive (AP to BL) or to the basolateral compartment for secretory (BL to AP) transport studies. Polymers were only added on the apical side. Samples of 100 μl were withdrawn from the acceptor compartment every 30 min over a time period of 3 h and immediately replaced by an aliquot of 100 μ l of artificial intestinal fluid equilibrated at 37°C. The amount of permeated model substrate was measured photometrically using a microtitration plate reader (Fluostar Galaxy, Offenburg, Germany). Cumulative corrections were made for previously removed samples.
Caco 2 permeation studies were performed using transwell filters. The transport medium was HEPES buffered saline, pH 6.8. The permeability studies were initiated by adding 1 ml of fresh buffer into the apical compartment. 1.5 ml of fresh buffer was replaced in the basolateral compartment. Rhodamine 123 (0.001%; w/v) and FD 4 (0.001%, w/v) were used as permeation markers. Polymers were swollen in buffer and added in final concentration of 0.25% (w/v). At time zero, donor solution was withdrawn for quantification of initial counts. The monolayers were incubated at 37°C in a controlled atmosphere without shaking for 3 h. At 30-min intervals, 100 μ l samples were removed from each basolateral compartment and immediately replaced with fresh HEPES buffered saline, pH6.8.
Data Analysis
The apparent permeability values (Papp) were calculated according to the following equation:
where dQ/dt is the flux of drug across the monolayer (mol/s), c0 is the initial concentration in the donor compartment (mol/ml), and A is the permeation area expressed as cm2. The apparent permeability values are expressed as cm/s.
Efflux ratios were calculated from Papp values by R = secretory Papp/absorptive Papp. All data presented are the mean values ± SD of six different experiments.
TEER values of filter-grown cells were measured using the Millicell-ERS epithelial voltohmmeter (Millipore Co., Bedford, MA) at various time intervals. The TEER values were calculated according to the following equation:
where Rtotal is the measured resistance, Rfilter w/o cells is the resistance of inserts alone, and A is the surface area. A significant decrease in TEER values compared with the control was taken as indicator for the loss of monolayer integrity. After 21 d of culture, control cells in MEM, pH 7.4, displayed a mean TEER value of 495 ± 21 Ω cm2.
Cell Death Measurement
The MTT test according to Mosmann and colleagues (Citation1983) was performed to assay the cellular proliferation and survival of Caco 2 cells exposed to CAC solutions. Controls were formed of unmodified chitosan, as a positive control 1 ml of Triton X was used and cells only formed the negative control. The amount of yellow MTT (3-(4,5-dimethylthiazol-2-yl)-2,5-diphenyltetrazolium bromide) oxidized to purple formazan is measured photometrically at a wavelength of 570 nm with background subtraction of 650 nm. The oxidation takes place only when mitochondrial reductase enzymes are active, and thus conversion is directly related to the number of viable cells. All experiments were run six times. Polymer solutions were prepared in MEM without phenol red at pH 7.4. To exclude cytotoxic effects due to changes in osmolarity and pH of the polymer solutions in comparison to cell culture medium, physiologically well tolerated values were adjusted. Before use, Caco 2 cells were seeded and incubated in 12-well microtiter plates for 24 h. The culture medium was replaced by 1 ml of polymer solutions solved in MEM without phenol red and incubated overnight. Subsequently polymer solutions were aspirated and 1 ml of MTT reagent was added. The production of purple formazan inside cells was allowed to proceed for 3 hours. After incubation time, unreacted dye was removed by aspiration and the insoluble formazan crystals were dissolved in 1 mol acidic isopropanol (0.04 M HCl in absolute isopropanol). The dye solution was centrifuged at 13,000 rpm for 2 min and measured by a Beckmann DU-600 spectrophotometer. The relative cell viability (%) related to control wells containing cell culture medium without polymer was calculated by [A] test/ [A] control × 100.
In order to evaluate the toxicity of CAC conjugates, a colorimetric assay for the quantification of cell death and cell lysis, based on the measurement of lactate dehydrogenase (LDH) activity released from the cytosol of damaged cells into the supernatant was used (Decker and Lohmannmatthes Citation1988; Legrand et al. Citation1992). Cells were exposed over a time period of 3 h to different concentrations of control and test polymers. Working solutions of 400 kDa CAC conjugate were obtained by dilution in MEM without phenol red, pH 7.4, to give final concentrations of 0.25 and 0.5%. Control solutions were formed of unmodified chitosan in the same final concentrations as described formerly for the test solutions, more precisely 0.25% and 0.5% polymer in MEM without phenol red, pH 7.4. The negative control was fresh MEM, pH 7.4, and the positive control was a 2% Triton® X100 HEPES (25 mM) at pH 7.4.
Confluent Caco 2 monolayers, cultured on plastic 12-well plates (1 × 105 cells/well) in MEM containing 20% FCS were incubated with 1 ml of each working solution for 3 h in a controlled atmosphere (37°C, 95% O2, 5% CO2). Supernatant was withdrawn at time points 0, 30, 60, 120, and 180 min, samples were centrifuged at 250 g for 4 minutes and stored at 4°C before analysis using the cytotoxicity detection kit by Sigma. The lactat dehydrogenase assay solution was prepared at time of use by mixing equal amounts of LDH assay substrate, co-factor, and dye solutions. The enzymatic reaction was allowed to proceed at room temperature for 30 minutes under light protection and stopped with 1/10 volume of 1 M hydrochloric acid added to each well. Absorbance was measured photometrically at a wavelength of 492 nm using a Fluostar Galaxy microplate reader (BMG Labtech, Vienna, Austria). Background was measured at 690 nm and subtract from the primary wavelength measurement. To determine the percentage cytotoxicity, the average absorbance values of the triplicates were calculated and from each of these the absorbance values obtained in the background control was subtracted. The resulting values were substituted in the following equation:
Statistical Analysis
Results are expressed as mean ± S.D. Significance was calculated using the Student t-test with p < 0.05. Origin Version 7.0 was used for calculations.
RESULTS
Characterization of the Chitosan-N-acetyl Cysteine Conjugate
N-acetyl cysteine, known as mucolytic agent with beneficial effects in disorders aligned to oxidative stress, was covalently linked to three chitosans differing by their molecular mass. The lyophilized product of all conjugates showed white, odorless cotton like structure. A detailed characterisation of CAC including mucoadhesive properties, biodegradability and swelling behavior has been performed in a study previously published by our group (Schmitz et al. 2006). The isolated conjugates displayed the following specifications: 353.7 μ Mol ± 29.8 μ Mol thiol groups per gram polymer for 150 kDa CAC, 325.5 ± 41.8 μ Mol thiol groups per gram polymer for 400 kDa CAC conjugate and 298.16 μ Mol ± 37.4 μ Mol thiol groups per gram polymer for 600 kDa CAC conjugate, where 54.2%, 43.1%, and 47.6%, respectively, of the thiol groups were oxidized to disulfide bonds during the coupling reaction.
Effect of Chitosan-N-acetyl Cysteine Conjugates on Drug Permeation in the Ussing-Type Chamber Model
Within this study, the effect of CAC conjugates of increasing molecular mass on the transepithelial transport of FD 4 and of Rho-123—a specific P-gp substrate—was evaluated.
Permeation studies with Rho-123 outlined in in absence of CAC conjugates showed a significantly better transport in the secretory than in the absorptive direction. As shown in , the secretory Papp was 2.4-fold of the absorptive Papp at 37°C. These profiles can be explained by a combination of active and passive transport, whereas the active P-gp efflux transport activity from basolateral to apical is responsible for an extrusion of Rho-123 out of the cell.
FIG. 1 Transepithelial transport of Rho-123 on freshly excised rat intestine in the Ussing-type chamber model in the absorptive (▵) and secretory (▴) direction in absence of polymer in comparison to the permeation profiles of Rho-123 in the absorptive direction in presence of 150 kDa CAC (♦), 400 kDa CAC (▪) and 600 kDa CAC (×). Transport data are means (± SD, n = 3) of the percentage of the total dose of Rho-123 applied to the apical or basolateral side of the mucosa.
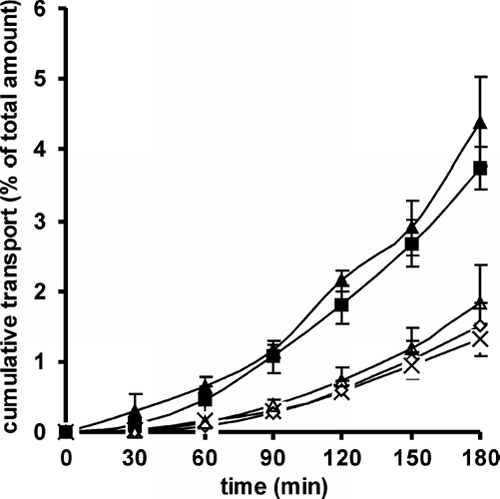
TABLE 1 Comparison of the absorptive and secretory apparent permeability coefficient (Papp) of Rho-123 and FD 4 and resulting efflux ratio (secretory Papp/ absorptive Papp) and improvement ratio (Papp control/ Papp test polymer) in the presence of indicated test polymers (means ± SD, n = 3–5) in the Ussing chamber model and Caco 2 model.
Depending on their molecular mass CAC conjugates had a variable influence on the permeation profile of Rho-123 when tested on rat intestine. 150 kDa and 600 kDa CAC conjugates exhibited a minor permeation enhancing effect for Rho-123. Data were almost identical to the values obtained for the apical to basolateral direction in absence of polymer. According to the Student t test, with p < 0.05, only the values at time point t = 180 minutes were significantly different. However, the 400 kDa CAC conjugate showed a significant permeation enhancement, in comparison to control and the two other conjugates. The apparent permeation enhancing effect, which can be explained by an inhibition of P-gp efflux transporter, was in the same range as the epithelial transport observed in the secretory direction.
shows the permeation profiles of FD 4 performed on rat intestine in the Ussing-type chamber model. In analogy to the permeation behavior of Rho-123, the basal to apical transport of FD 4 was predominant, being 4.1-fold higher than in the absorptive direction. The weakest permeation of FD 4 was observed in presence of 150 kDa CAC conjugate. The 400 kDa and 600 kDa conjugates, however, showed a permeation enhancing effect which was 2.5-fold improved for the 400 kDa and 1.8-fold for the 600 kDa CAC conjugate. The observed transport values for all three CAC conjugates suggest that the viscosity is an important parameter for the permeation of FD 4.
FIG. 2 Transepithelial transport of FD 4 on freshly excised rat intestine in the Ussing-type chamber model in the absorptive (▵) and secretory (▴) direction in absence of polymer in comparison to the permeation profiles of FD 4 in the absorptive direction in presence of 150 kDa CAC (♦), 400 kDa CAC (▪) and 600 kDa CAC (χ). Transport data are means (± SD, n = 3) of the percentage of the total dose of FD 4 applied to the apical or basolateral side of the mucosa.
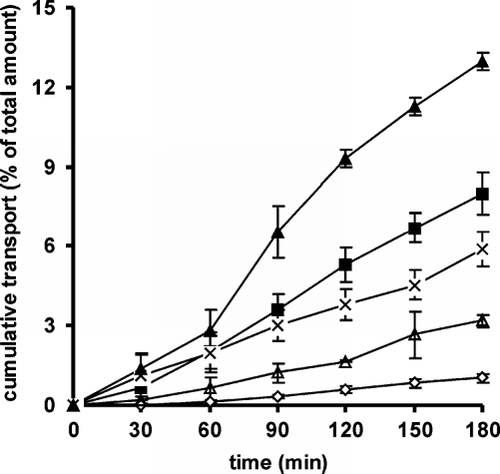
Effect of 400 kDa Chitosan-N-acetyl Cysteine Conjugates on Drug Permeation in the Caco 2 Cell Model
The influence of 400 kDa CAC conjugate on the transepithelial permeation of Rho-123 in the absorptive and secretory direction is shown in .
FIG. 3 In vitro permeation profile of Rhodamine 123 (Rho-123) performed on Caco 2 cell monolayers in absence (▪) and in presence (□) of 400 kDa CAC in the absorptive direction in comparison to the permeation profiles in absence (▴) and in presence (▵) of 400 kDa CAC in the secretory direction. Transport data are means (± SD, n = 3) of the percentage of the total dose of Rho-123 applied to the apical or basolateral side of the monolayers.
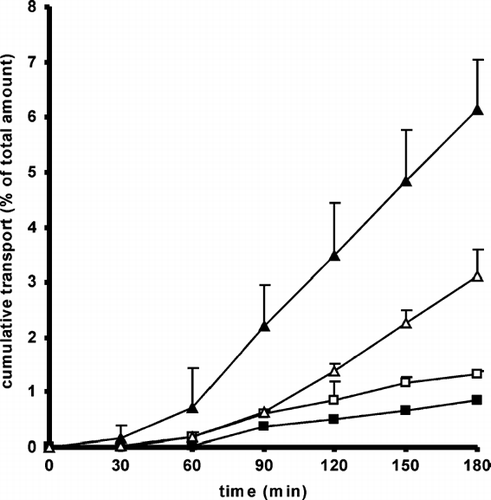
Taken into account that Rho-123 is a P-gp efflux transporter substrate, the transport rate of Rho-123 from basolateral to apical was improved in comparison to that from apical to basolateral. In absence of polymer, we observed a 7.2-fold higher transport in the secretory direction. Papp values are shown in . The permeation enhancing effect of 400 kDa CAC conjugate in the absorptive direction was 1.5-fold higher than control. However, the efflux profile of Rho-123 in the secretory direction was significantly changed in presence of CAC, being decreased 1.9-fold.
The permeation profiles of FD 4, performed in Caco 2 cell monolayers have shown that in comparison to the Ussing-type chamber model, only a low amount of the total delivered dose of FD 4 permeated through the monolayers. 400 kDa CAC conjugate had no significant permeation enhancing effect. Transport rates were almost identical in both the absorptive and the secretory direction in absence or presence of thiolated polymer. Efflux ratio for FD 4 was 4.8 in absence and 5.5 in presence of polymer.
Results of Cytotoxicity Studies
The safety of CAC conjugate, supposed to be used as pharmaceutical excipient, was assayed by standard cytotoxicity tests. Cell viability in face of the proposed thiolated chitosan was measured over 24 hours using the MTT test. Additionally, the LDH test was applied to quantify the effect of CAC conjugate on cell death and cell lysis.
Results of the MTT test are outlined in .
FIG. 4 Cell proliferation measured by MTT assay. The relative cell viability in percent in presence of 400 kDa CAC was calculated in relation to control formed of cell culture medium only. Data are means of at least three experiments ± standard deviation.
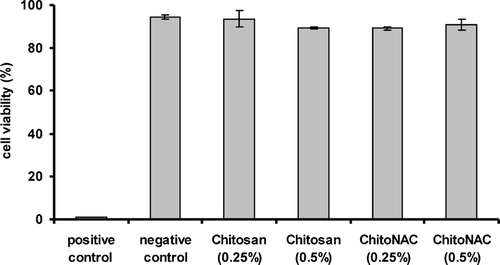
Triton X, used as positive control had a deadening effect on the cells, so that approximately 1% of cells remained intact. In contrast, the negative control formed of untreated cells showed the highest viability. Measured values showed consistent cell viability over 90%. The influence of chitosan and CAC conjugate on cell viability was evaluated in 0.25% and 0.5% (w/v) concentrations. Both polymers showed to have no significant effect on the cell viability in comparison to negative control.
The influence of two polymer concentrations was also tested within the LDH test. Results of this study are outlined in . The overall cytotoxicity of all tested compounds was relatively low, measured values were beyond 20% cell toxicity. Results showed slight differences between the 0.25% and the 0.5% concentrations, whereas the higher concentration was more toxic, but in a negligible manner.
FIG. 5 Quantification of cell death and cell lysis by lactate dehydrogenase activity measurement. Cytotoxicity was measured over a time period of three hours and expressed in percent. Legend: Stripped bars represent 0.25% unmodified chitosan, black bars 0.5% unmodified chitosan, grey bars represent the 0.25% 400 kDa CAC conjugate and white bars the 0.5% 400 kDa CAC conjugate. Data are means of at least three experiments ± standard deviation.
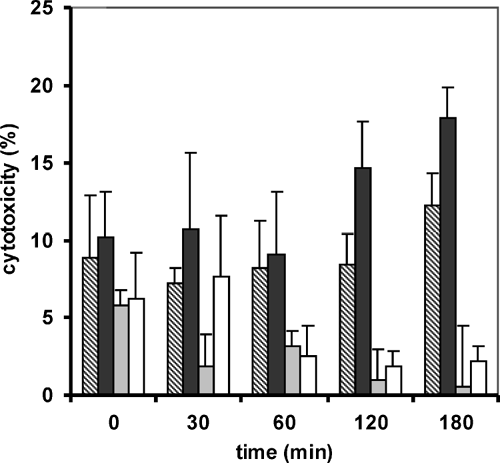
DISCUSSION
The proposed study investigated the relevance of a CAC conjugate as a polymeric P-gp efflux pump inhibitor on freshly excised rat intestine and in a cell culture model. The permeation enhancing properties have been assessed for Rho-123, chosen as specific substrate for apically polarized efflux proteins and for FD 4 as trans- and paracellular marker. Recently, Werle et al. have ascertained the validity of such a test system using chitosan-thiobutylamidine as alternative thiolated polyaminosaccharid for transport studies of Rho-123 in guinea pig intestine, introducing thiomers as potentially interesting candidates for efflux pump inhibition (Werle and Hoffer Citation2005).
In the rat model, the transepithelial transport of the P-gp substrate Rho-123 from the basolateral to the apical side of ileum mounted in the Ussing-type chamber was significantly higher (p < 0.005) than from the apical to basolateral side. A comparable transport ratio for Rho-123 was also observed in the Caco 2 cell model. This gap in permeation rates is seen as a typical indication for the involvement of efflux pumps in transport kinetics (Varma et al. Citation2003). Surprisingly, the efflux of FD 4 in the Ussing-type chamber model and in Caco 2 cell monolayers showed likewise a significantly higher permeation clearance from basal to apical than from apical to basal at 37°C. Tomita and colleagues (Citation1999) were able to give an explanation for the large discrepancy in transport rates, suggesting that under normal conditions, the flux of FD 4 is transported across Caco 2 cell monolayers in a polarized fashion by at least two energy requiring systems, one of which shows substrate specificity for polysaccharide and transcytosis (Tomita et al. Citation1999; Ohkubo et al., Citation2003).
In comparison to controls formed of either Rho-123 or FD 4, the P-gp inhibiting effect of three CAC conjugates differing by their average molecular mass was evaluated in an Ussing-type chamber model. Based on cysteine scanning mutagenesis models, sulfhydryl compounds have been proven to change the membrane structure, resulting in conformational changes of efflux transporter proteins (Demeule et al. Citation2004). Loo et al. were able to demonstrate that bis(diethylthiocarbamoyl)-disulfide (disulfiram), a drug used to treat alcoholism, can potentially reduce P-gp mediated drug resistance by inhibiting P-gp activity via cysteine modification and/or blocking its maturation (Loo and Clarke Citation2000; Loo et al. Citation2003). These tests revealed that the conversion of the drug binding pocket from a high-affinity to a low-affinity state through alterations in the packing of the transmembrane segments is one of the mechanisms responsible for drug efflux mediated by P-glycoprotein. Additionally P-gp is highly dependant on the physical state and microviscosity of the surrounding lipid bilayer. Changes in the membrane microviscosity can inhibit its activity (Regev et al. Citation1999). Based on these findings, it can be assumed that the thiolated polymer introduced within this study is able to inhibit P-gp efflux pumps by changing the molecular conformation of the transporter, expressed finally in a permeation enhancement of the corresponding substrates. Among all three chitosan conjugates tested, however, only 400 kDa CAC conjugate was able to provide a distinct improvement in the transepithelial transport from apical to basolateral. This phenomenon can be explained by the underlying mechanisms involved in the permeation enhancing properties of many mucoadhesive polymers. The molecular mass is a crucial parameter associated to a variety of properties exhibited by polymeric excipients. The main physical mechanism of mucoadhesion, which is related closely to the permeation enhancing characteristics, seems to be based on the interpenetration, or so-called interdiffusion, of the polymeric chains into the highly entangled network of the mucus. Imam et al. showed that the lower the molecular mass is, the higher is the interpenetration but the lower are the cohesive and mucoadhesive properties (Imam et al. Citation2003). The latest are mainly responsible for the development of a permeation enhancing effect based on the thiol groups immobilized on the polymeric backbone of the polymer. According to this, mucoadhesion, cohesiveness and in consequence permeation enhancing properties are improved with increasing molecular mass; however they are limited on the other hand by a decreasing interpenetration. Consequently, the optimal molecular mass of a polymer has to be high enough to provide sufficient cohesive properties, but not too high, in order to guarantee the maximum feasible extent of interpenetration. Based on this evidence, it can be assumed that the poor permeation enhancing effect of 600 kDa CAC conjugate is connected either to an insufficient interpenetration or balanced by P-gp mediated efflux, while inadequate mucoadhesive and cohesive properties prevent a permeation enhancing effect of the 150 kDa CAC derivate. The optimal molecular mass was provided by 400 kDa CAC, which exhibited strong permeation enhancing properties. A plausible cause for those characteristics may be found in the conformational change of the transmembraneous P-gp efflux pump due to thiol groups immobilized on the backbone of the polymeric excipients (Liu and Sharom Citation1996). Possible thiol/disulfide redox reactions have gained a lot of attention as they have been identified to play a significant role in the inhibition of human P-gp transporter proteins (Loo and Clarke Citation2000). An involvement of thiol groups to the inhibition of P-gp efflux was also discussed by Föger and colleagues (Citation2006) who demonstrated that 400 kDa thiolated chitosan is a promising tool for oral administration of P-gp substrates.
The molecular mass dependant permeation was confirmed by transepithelial transport of FD 4 from apical to basal side. While 150 kDa CAC seemed to impede the transport of FD 4, 400 kDa and 600 kDa chitosan conjugates induced a pronounced permeation of FD 4, probably due to a tight and firm contact of the polymer with the mucosa, resulting in a steep concentration gradient of FD 4 at the site of absorption.
Transport rates of Rho-123 in a Caco 2 cell monolayer were increased in presence of 400 kDa CAC conjugate in the absorptive direction. Bearing in mind that in absence of a mucus gel layer mucoadhesive properties cannot be deployed, the diminished transepithelial efflux in the secretory direction can only be explained by an inhibition of the active transporter system. This inhibiting effect may also explain the minor permeation improvement from the apical to the basal side.
In contrast, the permeation profiles of FD 4, a substrate whose efflux is not mediated by the P-gp transporter system, showed that the thiolated conjugate had no influence on the absorption or extrusion of FD 4, confirming the conclusions drawn above. Accordingly, results suggest that the CAC conjugate has no influence on the transport systems identified by Tomita and colleagues (Citation1999) responsible for the FD 4 efflux in Caco 2 monolayers.
With the development of P-gp inhibitors for the treatment of drug-resistant tumors, toxicity has become a major concern. As with any drug, efficacy has to be balanced with toxicity. Thus the polymeric drug carriers must be specific in order to minimize or exclude undesirable effects, not interfere with metabolism of the co-administered agent and allow the agent to be administered at the standard clinical dose. Although thiolated chitosans have proven to be a promising new and safe category of multifunctional polymeric excipients, this study was completed by standard cytotoxicity tests (Bernkop-Schnürch et al. Citation2004). Results showed that CAC conjugate is a non-cytotoxic agent, which is not absorbed from the gastrointestinal tract due to its molecular weight. Consequently systemic toxic side effects can be excluded.
In conclusion, CAC conjugate was identified as toxicological harmlessness inhibitor of the P-gp efflux transporter system, representing an easy accessible promising tool for oral administration of P-gp substrates.
ACKNOWLEDGMENT
This work was supported by Grant no.: BFR 03/019 from the “Ministère de la Culture, de l‘Enseignement Supérieur et de la Recherche (MCESR)-Luxembourg” to Thierry Schmitz.
REFERENCES
- Bernkop-Schnürch A., Kast C. E., Guggi D. Permeation enhancing polymers in oral delivery of hydrophilic macromolecules: thiomer/GSH systems. J. Control. Release 2003; 93: 95–103
- Bernkop-Schnürch A., Hornof M., Guggi D. Thiolated chitosans. Eur. J. Pharm. Biopharm. 2004; 57: 9–17
- Clausen A. E., Kast C. E., Bernkop-Schnürch A. The role of glutathione in the permeation enhancing effect of thiolated polymers. Pharm. Res. 2002; 19: 602–608
- D'Emanuele A., Jevprasesphant R., Penny J., Attwood D. The use of a dendrimer-propranolol prodrug to bypass efflux transporters and enhance oral bioavailability (vol 95 pg 447 2004). J. Control. Release 2004; 96: 513–513
- Decker T., Lohmannmatthes M. L. A Quick and simple method for the quantitation of lactate-dehydrogenase release in measurements of cellular cyto-toxicity and tumor necrosis factor (Tnf) activity. J. Immunol. Methods 1988; 115: 61–69
- Demeule M., Brossard M., Turcotte S., Regina A., Jodoin J., Beliveau R. Diallyl disulfide, a chemopreventive agent in garlic, induces multidrug resistance-associated protein 2 expression. Biochem. Biophys. Res. Commun. 2004; 324: 937–945
- Föger F., Schmitz T., Bernkop-Schnürch A. In vivo evaluation of an oral delivery system for P-gp substrates based on thiolated chitosan. Biomaterials 2006; 27: 4250–4255
- Guggi D., Bernkop-Schnürch A. Improved paracellular uptake by the combination of different types of permeation enhancers. Int. J. Pharm. 2005; 288: 141–150
- Ho G. T., Moodie F. M., Satsangi J. Multidrug resistance 1 gene (P-glycoprotein 170): an important determinant in gastrointestinal disease?. Gut 2003; 52: 759–766
- Hugger E. D., Novak B. L., Burton P. S., Audus K. L., Borchardt R. T. A comparison of commonly used polyethoxylated pharmaceutical excipients on their ability to inhibit P-glycoprotein activity in vitro. J. Pharm. Sci. 2002; 91: 1991–2002
- Imam M. E., Hornof M., Valenta C., Reznicek G., Bernkop-Schnürch A. Evidence for the interpenetration of mucoadhesive polymers into the mucus gel layer. S. T. P. Pharma Sci. 2003; 13: 171–176
- Kabanov A. V., Batrakova E. V., Alakhov V. Y. Pluronic block copolymers for overcoming drug resistance in cancer. Adv. Drug Deliv. Rev. 2002; 54: 759–779
- Legrand C., Bour J. M., Jacob C., Capiaoumont J., Martial A., Marc A., Wudtke M., Kretzmer G., Demangel C., Duval D. Lactate dehydrogenase (LDH) activity of the cultured eukaryotic cells as marker of the number of dead cells in the medium [corrected]. J. Biotechnol. 1992; 25: 231–243
- Liu R., Sharom F. J. Site-directed fluorescence labeling of P-glycoprotein on cysteine residues in the nucleotide binding domains. Biochemistry 1996; 35: 11865–11873
- Loo T. W., Clarke D. M. Drug-stimulated ATPase activity of human P-glycoprotein is blocked by disulfide cross-linking between the nucleotide-binding sites. J. Biol. Chem. 2000; 275: 19435–19438
- Loo T. W., Clarke D. M. Blockage of drug resistance in vitro by disulfiram, a drug used to treat alcoholism. J. Natl. Cancer Inst. 2000; 92: 898–902
- Loo T. W., Bartlett M. C., Clarke D. M. Substrate-induced conformational, changes in the transmembrane segments of human P-glycoprotein-Direct evidence for the substrate-induced fit mechanism for drug binding. J. Biol. Chem. 2003; 278: 13603–13606
- Mosmann T. Rapid colorimetric assay for cellular growth and survival: application to proliferation and cytotoxicity assays. J. Immunol. Methods 1983; 65: 55–63
- Ohkubo R., Tomita M., Hotta Y., Nagira M., Hayashi M. Comparative study of flux of FITC-labeled Dextran 4000 on normal (iso)- and hyper-osmolarity in basal side in caco-2 cell monolayers. Drug Metab. Pharmacokinet. 2003; 18: 404–408
- Regev R., Assaraf Y. G., Eytan G. D. Membrane fluidization by ether, other anesthetics, and certain agents abolishes P-glycoprotein ATPase activity and modulates efflux from multidrug-resistant cells. Eur. J. Biochem. 1999; 259: 18–24
- Schmitz T., Grabovac V., Palmberger T. F., Hoffer M. H., Bernkop-Schnürch A. Synthesis and characterization of a chitosan-N-acetyl cysteine conjugate. Int. J. Pharm. 2008; 347: 79–85
- Tomita M., Hotta Y., Ohkubo R., Awazu S. Polarized transport was observed not in hydrophilic compounds but in dextran in Caco-2 cell monolayers. Biol. Pharm. Bull. 1999; 22: 330–331
- Varma M. V. S., Ashokraj Y., Dey C. S., Panchagnula R. P-glycoprotein inhibitors and their screening: a perspective from bioavailability enhancement. Pharmacol. Res. 2003; 48: 347–359
- Werle M., Hoffer M. Glutathione and thiolated chitosan inhibit multidrug resistance P-glycoprotein activity in excised small intestine. J. Control. Release 2005; 111: 41–46
- Zhou X. H., Po A. L. W. Peptide and protein drugs.1. Therapeutic applications, absorption and parenteral administration. Int. J. Pharm. 1991; 75: 97–115