Abstract
This work suggests the use of chitosan gel imbued with the photosensitizer Photogem and with the antibiotic Tetraclin as a possible drug delivery system. The results reveal a decrease in the photosensitizer level of toxicity. Besides, the interaction between Photogem® and chitosan gel causes a red shift in the photosensitizer spectrum, increasing its absorption in the therapeutic window (600–700 nm). These characteristics indicate this compound as a promising natural polymer-based photosensitizer carrier for photodynamic therapy. In summary, our results show that pure and doped chitosan gel may have potential application for antimicrobial action, being an excellent alternative when local control of the drug administration, provided by the gel, is required.
1. INTRODUCTION
Chitosan is a hydrophilic biopolymer obtained from chitin, a constituent from the exoskeletons of arthropods, which exhibits several promising biological activities. This bio-polymer presents interesting characteristics, such as nontoxicity, good biocompatibility (CitationHirano and Noishiki 1985), bioabsorbility (CitationMuzzarelli et al. 1988), and biodegradation, and can be used as a film, gel, or solution (CitationShin et al. 2005). It has been demonstrated that chitosan presents antimicrobial and antifungal activity against many microorganisms, being commercially used as a bioinsecticide and as an agent for fruit conservation (CitationChoi et al. 2002). In addition, it has been demonstrated to be hemostatic (CitationRao and Sharma 1997) and bacteriostatic (CitationTarsi et al. 1997; CitationJe and Kim 2006). This material has been used as a bioadhesive and permeable agent, which allows its application for drug delivery (CitationTakeuchi et al. 2003; CitationNeedleman et al. 1998), being specially proposed as an antibiotics releaser bacterial reduction in oral applications (CitationLiu et al. 2004; CitationDecker et al. 2005). Moreover, chitosan polymer has shown to be a promising biomaterial when used for bone repair (CitationShin et al. 2005; CitationLee et al. 2002; CitationMuzzarelli and Muzzarelli 2002; CitationPark et al. 2003) and tissue regeneration (CitationGerentes et al. 2002; CitationKuo et al. 2006; CitationPang et al. 2005), prompting recent interest in antibacterial and immunostimulative effect (CitationMoon et al. 2007).
Several controlled drug delivery systems have been developed to enhance the drug efficacy and minimize the side effects (CitationChen et al. 2005). The application of chitosan gel, for example, can provide a long stay in the target tissues, adequate drug penetration, high efficacy, and acceptability (CitationIkinci et al. 2002; CitationEl-Samaligy et al. 2006). Besides being an interesting option as a local drug delivery system, the antimicrobial action of the chitosan gel and its molecular interaction with the drugs can further enhance the bactericidal effect (CitationLiu et al. 2004). Considering all the interesting characteristics of chitosan previously mentioned, we propose in this study the use of chitosan gel not only to deliver drug for antimicrobial agents (antibiotics), but also to deliver photosensitizers with less dark toxicity for photodynamic therapy (PDT). We present here the formulation of pure chitosan gel as well as doped with Tetracycline (Tetraclin) and Photogem, drugs commonly and successfully used in antibiotics and PDT treatments, respectively. Tetracycline (Tetraclin) will be used in this study as a control of bacterial reduction action. The antimicrobial activity of those compounds, whose molecular structures are shown in , were studied using the disk diffusion method in Staphylococcus aureus, an opportunist pathogen found in the microbiota of mucous membrane (buccal, nasal, and aural) and human skin, capable of causing serious infection when penetrated into the human organism. The possible advantages of such approach are discussed in this paper.
2. MATERIALS AND METHODS
2.1 Chitosan
Chitosan, obtained from shrimp chitin (an N-acetylgluco- samine polymer), was provided by Cyrbe do Brasil Corporation. It has an average molecular weight of 500,000 g/mol, as determined by viscometry, and a 75% degree of deacetylation, as obtained from 1HNMR measurements (CitationSignini and Campana 1999). It was purified twice by solubilization in a 1% aqueous acetic acid solution, filtered with filter paper, precipitated with a 1% aqueous sodium hydroxide solution, and washed extensively with deionized water until neutral pH was reached. Hydrochloroauric acid was purchased from Aldrich and used without further purification. All glassware was thoroughly cleaned using detergent, aqua regia, and copious rinsing with deionized water (CitationSantos et al. 2004).
2.2 Microorganism
The culture medium agar Mueller–Hinton, selective for S. aureus, was poured into Petri dishes (100 × 20 mm). After the agar solidified, the plates were wrapped and kept at 4°C for 3 days before the experiments.
S. aureus strain (ATCC 25923) employed in this work was suspended in the tryptic soy broth (Difco) for 24 h at approximately 37°C. For the adjustment of inoculum density, cell numbers were estimated through Mc Farland standard being at approximately 108 microorganisms/ml. Bacteria cultivation was carried out in laminar flux, in which 200 μl of Staphylococcus aureus broth was added to each Petri dish. This microbial suspension was uniformly spread on the agar with using a sterile Drigalski loop.
2.3 Disk Diffusion Method
The antimicrobial properties of the chitosan gels and solutions studied here were tested with the disk diffusion method, as standardized by the National Committee for Clinical Laboratory Standards (CitationOdland et al. 2000). This experiment was carried out with the following compounds: (1) chitosan gel (CG), (2) chitosan gel doped with tetracycline (Tetraclin–Teuto) (CG+T), (3) chitosan gel doped with Photogem (CG+P), (4) tetracycline solution (T), and (5) Photogem solution (P). All compounds were prepared previous to each test, according to the subsequent description. Initially, aqueous solutions of hydrochloride tetracycline 100 mg/ml and Photogem 5 mg/l were produced and light protected. These solutions were made using Mili-Q water. A chitosan (Cyrbe do Brasil) slurry was prepared adding 0.25 g of chitosan, purified as previous described by CitationSantos et al. (2004), into 9 ml of 0.16 mol/l ascorbic acid aqueous solution. The chitosan gel doped with the drugs were prepared by adding 9 ml of the solutions mentioned above to the chitosan slurry followed by manually stirring by using a glass stripe. This procedure results in gels with satisfactory aggregation and easy manipulation.
Four sterile absorber paper disks (5 mm diameter) were placed on the agar plates inoculated with the S. aureus. These disks were positioned equidistantly from each other, to avoid superposition of the inhibition halos. Using an automatic pipette, 2 μl of compounds previously described were applied on top of each disk. The plates were kept at room temperature for 10 min to allow the material diffusion. Subsequently, samples were grown aerobically at 37°C up to 48 hr. Inhibition zones around the studied compounds were measured after 24 and 48 hr of incubation. A disk without any compound was employed as a control group for our experiment. For each group, 10 repetitions were carried out, in order to provide statistical analyses of the obtained results.
2.4 Spectroscopy
The ultraviolet-visible absorption spectra of Photogem in solution and chitosan gel were recorded from 400 to 800 nm using quartz cuvets on a Cary 17 spectrophotometer.
3. RESULT AND DISCUSSION
The diameter of the inhibition zone was based on lack of bacterial growth, measured after 48 h. However, after 24 h the observed inhibition halo diameters had already reached their final values. The diameters of the halos, representing the bacteria inhibition, were measured using a pachymeter. When halos with no circular symmetry were observed, the diameter was taken as being the average between the largest and smallest axis.
presents the statistical study of the inhibition halos' diameters using ANOVA and TUKEY with p < 0.01, for the experimental groups C, T, CG + T, and CG.
TABLE 1 Means inhibition halos' diameters (d), standard deviation (SD), and standard error (SE) of selected groups: C, T, CG + T, and CG
In it is shown the inhibition halos' average diameters for the compounds T, CG+T, and CG (data from ). The control group (blank sample) exhibited no inhibition halo. In contrast, the chitosan gel (CG) shows a considerable halo, around 12 mm, revealing the efficiency of the chitosan as an antimicrobial agent for S. aureus. Previous studies have reported comparable results using different types of bacteria. According to CitationLiu et al. (2004) and CitationDidenko et al. (2005) chitosan possesses reactive positively charged amino groups that interact with the bacterial cell membranes, resulting in the leakage of intracellular constituents and alteration of cell permeability, which leads to the microorganism death (CitationLiu et al. 2004; CitationJe and Kim 2006; CitationMoon et al. 2007). The results for tetracycline in solution (T) and in the chitosan gel (CG + T) present the highest diameters for the inhibition zone, around 32 mm. This result reveals that the association of chitosan with tetracycline did not affect the efficiency of the antibiotic, as demonstrated by similar halo growths observed in . The absence of any accumulative effect of the chitosan gel on the tetracycline bacteriostatic effect is probably related to the faster action of tetracycline's, once only the chitosan gel also presented significant bacterial reduction, as shown in . Besides providing antimicrobial action, the chitosan gel can also be applied as a tetracycline delivery system for easier administration, with potential application in Periodontology.
FIG. 2. Average diameter of the inhibition zone for tetracycline in solution (T), chitosan gel doped with tetracycline the (CG + T), and chitosan gel (CG). The first data illustrates the absence of inhibition observed for the control group. The error bars are given by the confidence intervals of statistical test.
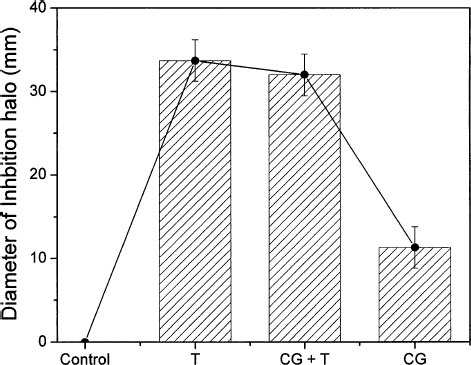
TABLE 2 Means inhibition halos' diameters (d), standard deviation (SD), and standard error (SE) of selected groups: C, P, CG + P, and CG
In order to further study the promising use of chitosan as a local drug delivery system, specifically for photodynamic therapy, we produced gels imbued with Photogem. presents the statistical study of the inhibition halos' diameters using ANOVA and TUKEY with p < 0.01, for the experimental groups C, T, CG + T, and CG.
In we present the results of the chitosan gel as a vehicle for the photoactive drug (Photogem). presents the average diameter of the inhibition zone for Photogem in solution (P), chitosan gel doped with Photogem (CG + P) and chitosan gel (CG). It can be seen that Photogem solution (P), in the concentration employed here, acts against bacteria growth forming an inhibition halo of 20 mm, even in the dark. However, when Photogem® is associated with chitosan (CG + P) the bacterial reduction efficiency decreased, presenting an inhibition halo of 10 mm. This effect can probably be associated with the interaction between chitosan and Photogem, which is a porphyrin whose acid groups are well known to interact with chitosan, hindering the Photogem® toxicity. Furthermore, as can be seen by comparing the results achieved in for chitosan doped with Photogem (CG + P) and chitosan Gel (CG), the bactericidal action of CG is preserved when Photogem is added. The availability of increasing the photosensitizer content in the chitosan gel without raising the dark toxicity level is a desirable feature for PDT applications, in which the main antimicrobial action must happen only when the photosensitizer is activated by proper light irradiation, resulting in a cytotoxic effect that causes damage to the microorganism or target-cell (CitationWood et al. 1999; CitationWainwright 1998; CitationHamblin and Hasan 2004; CitationPfitzner et al. 2004; CitationRovaldi et al. 2000).
FIG. 3. Average diameter of the inhibition zone for Photogem® in solution (P), chitosan gel doped with Photogem® the (CG + P), and chitosan gel (CG). The first data illustrates the absence of inhibition observed for the control group. The error bars are given by the confidence intervals of statistical test.
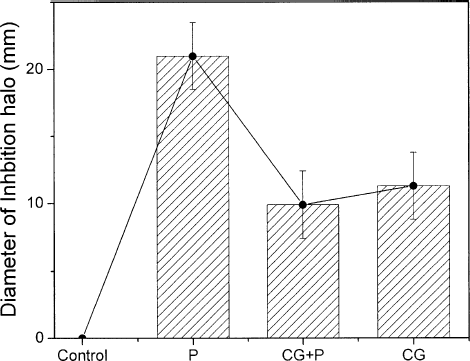
With the aim of verifying the interaction between chitosan and Photogem, the UV-Vis absorption spectra for the Photogem solution (a) and chitosan gel doped with Photogem (b) were obtained as shown in . The two bands observed are due to the Photogem (porphyrin). The band in the ultraviolet region, around 400 nm, corresponds to the B (Soret) band, while the structure around 550 nm is known as the Q band. Both transitions involve π−π* from the porphyrin ring (Kalyanasundaram Citation1992). As expected due to the interaction between the porphyrin acid groups and chitosan, a 50 nm red-shift in the UV-vis absorption spectrum of the doped chitosan gel was observed. Albeit such a shift was observed, it is clear from that the characteristic features (B and Q Band) from the porphyrin are still present, indicating that no compound degradation occurred. Besides decreasing the toxicity as previously mentioned, shows another advantage of using the chitosan gel-Photogem compound. The red-shift caused by the interaction with chitosan increased the Photogem absorption in the 600–700 nm region, in which human tissues are less absorptive. (CitationRogers et al. 2003; CitationIon et al. 1998). Therefore, the compound studied here enhances the photoactive drug light absorption in a suitable way for PDT. No changes in these absorbance spectra were observed by increasing the compounds temperature up to 60°C (results not shown), revealing that no thermal degradation will occur upon laser irradiation.
4. Conclusions
The obtained results demonstrated that chitosan gel pure and prepared with tetracycline present antimicrobial effect. In addition, the chitosan gel/Photogem® association revealed an increase in the photosensitizer absorption in therapeutic window (600–700 nm), allowing the use of light sources with deeper penetration into tissues, which can lead to increasing PDT efficiency. This combination also decreased the photosensitizer toxicity in the dark, another desirable feature for dye release in photodynamic therapy. Furthermore, the gel formulation has additional advantages, when compared with solutions, such as better control and more precision of local drug administration.
ACKNOWLEDGMENTS
Financial support from FAPESP (Fundação de Amparo á Pesquisa do Estado de São Paulo), CNPq (Conselho Nacional de Desenvolvimento Cientifico e Tecnológico) and CAPES (Coordenação de Aperfeiçoamento de Pessoal de Nível Superior) are gratefully acknowledged. We also thank Prof. Dr. Vanderlei Salvador Bagnato for furnishing Photogem® and Prof. Dr. Cleber R. Mendonca and Dr. Leonardo De Boni for helpful discussion.
REFERENCES
- W. R. Chen, M. Korbelik, K. E. Barteis, H. Liu, J. H. Sun, and R. E. Nordquist. (2005). Enhancement of laser cancer treatment by a chitosan-derived immunoadjuvant. Photochem. Photobiol. 81:190–195.
- W. Y. Choi, H. J. Park, D. J. Ahn, J. Lee, and C. Y. Lee. (2002). Wettability of chitosan coating solution on ‘Fuji’ apple skin. J. Food Sci. 67:2668–2672.
- E. M. Decker, C. von Ohle, R. Weiger, I. Wiech, and M. Brecx. (2005). A synergistic chlorhexidine/chitosan combination for improved antiplaque strategies. J. Periodontal Res. 40:373–377.
- L. V. Didenko, D. V. Gerasimenko, N. D. Konstantinova, T. A. Silkina, I. D. Avdienko, G. E. Bannikova, and V. P. Varlamov. (2005). Ultrastructural study of chitosan effects on Klebsiella and Staphylococci. Bull. Exp. Biol. Med. 140:356–360.
- D. S. dos Santos, P. J. G. Goulet, N. P. W. Pieczonka, O. N. Oliveira, and R. F. Aroca. (2004). Gold nanoparticle embedded, self-sustained chitosan films as substrates for surface-enhanced Raman scattering. Langmuir. 20:10273–10277.
- M. S. El-Samaligy, N. N. Afifi, and E. A. Mahmoud. (2006). Increasing bioavailability of silymarin using a buccal liposomal delivery system: preparation and experimental design investigation. Int. J. Pharm. 308:140–148.
- P. Gerentes, L. Vachoud, J. Doury, and A. Domard. (2002). Study of a chitin-based gel as injectable material in periodontal surgery. Biomaterials. 23:1295–1302.
- M. R. Hamblin, and T. Hasan. (2004). Photodynamic therapy: a new antimicrobial approach to infectious disease?. Photochem. Photobiol. Sci. 3:436–450.
- S. Hirano, and Y. Noishiki. (1985). The Blood compatibility of chitosan and N-acylchitosans. J. Biomed. Mater. Res. 19:413–417.
- G. Ikinci, S. Senel, H. Akincibay, S. Kas, S. Ercis, C. G. Wilson, and A. A. Hincal. (2002). Effect of chitosan on a periodontal pathogen Porphyromonas gingivalis. Int. J. Pharm. 235:121–127.
- R. M. Ion, A. Planner, K. Wiktorowicz, and D. Frackowiak. (1998). The incorporation of various porphyrins into blood cells measured via flow cytometry, absorption and emission spectroscopy. Acta Biochimica Polonica. 45:833–845.
- J. Y. Je, and S. K. Kim. (2006). Antimicrobial action of novel chitin derivative. Biochimica Et Biophysica Acta-General Subjects 1760:104–109.
- K. Kalyanasundaram. 1992. Photochemistry of polypyridine and porphyrincomplexes, London: Academic Press.
- S. M. Kuo, S. J. Chang, T. W. Chen, and T. C. Kuan. (2006). Guided tissue regeneration for using a chitosan membrane: an experimental study in rats. J. Biomed. Mater. Res. A 76A:408–415.
- J. Y. Lee, S. H. Nam, S. Y. Im, Y. J. Park, Y. M. Lee, Y. J. Seol, C. P. Chung, and S. J. Lee. (2002). Enhanced bone formation by controlled growth factor delivery from chitosan-based biomaterials. J. Control. Rel. 78:187–197.
- D. Z. Liu, W. P. Chen, C. P. Lee, S. L. Wu, Y. C. Wang, and T. W. Chung. (2004). Effects of alginate coated on PLGA microspheres for delivery tetracycline hydrochloride to periodontal pockets. J. Microencapsul. 21:643–652.
- H. Liu, Y. M. Du, X. H. Wang, and L. P. Sun. (2004). Chitosan kills bacteria through cell membrane damage. Int. J. Food Microbiol. 95:147–155.
- J. S. Moon, H. K. Kim, H. C. Koo, Y. S. Joo, H. M. Nam, Y. H. Park, and M. I. Kang. (2007). The antibacterial and immunostimulative effect of chitosanoligosaccharides against infection by Staphylococcus aureus isolated from bovine mastitis. Appl. Microbiol. Biotechnol. 75 (5):989–998.
- C. Muzzarelli, and R. A. A. Muzzarelli. (2002). Natural and artificial chitosan-inorganic composites. J. Inorgan. Biochem. 92:89–94.
- R. Muzzarelli, V. Baldassarre, F. Conti, P. Ferrara, G. Biagini, G. Gazzanelli, and V. Vasi. (1988). Biological-activity of chitosan—ultrastructural-study. Biomaterials 9:247–252.
- I. G. Needleman, G. P. Martin, and F. C. Smales. (1998). Characterisation of bioadhesives for periodontal and oral mucosal drug delivery. J. Clinical Periodontol. 25:74–82.
- B. A. Odland, M. E. Erwin, and R. N. Jones. (2000). Quality control guidelines for disk diffusion and broth microdilution antimicrobial susceptibility tests with seven drugs for veterinary applications. J. Clinical Microbiol. 38:453–455.
- E. K. Pang, J. W. Paik, S. K. Kim, U. W. Jung, C. S. Kim, K. S. Cho, C. K. Kim, and S. H. Choi. (2005). Effects of chitosan on human periodontal ligament fibroblasts in vitro and on bone formation in rat calvarial defects. J. Periodontol. 76:1526–1533.
- J. S. Park, S. H. Choi, I. S. Moon, K. S. Cho, J. K. Chai, and C. K. Kim. (2003). Eight-week histological analysis on the effect of chitosan on surgically created one-wall intrabony defects in beagle dogs. J. Clinical Periodontol. 30:443–453.
- A. Pfitzner, B. W. Sigusch, V. Albrecht, and E. Glockmann. (2004). Killing of periodontopathogenic bacteria by photodynamic therapy. J. Periodontol. 75:1343–1349.
- S. B. Rao, and C. P. Sharma. (1997). Use of chitosan as a biomaterial: studies on its safety and hemostatic potential. J. Biomed. Mater. Res. 34:21–28.
- J. E. Rogers, K. A. Nguyen, D. C. Hufnagle, D. G. McLean, W. J. Su, K. M. Gossett, A. R. Burke, S. A. Vinogradov, R. Pachter, and P. A. Fleitz. (2003). Observation and interpretation of annulated porphyrins: studies on the photophysical properties of meso-tetraphenylmetalloporphyrins. J. Physical Chem. A 107:11331–11339.
- C. R. Rovaldi, A. Pievsky, N. A. Sole, P. M. Friden, D. M. Rothstein, and P. Spacciapoli. (2000). Photoactive porphyrin derivative with broadspectrum activity against oral pathogens in vitro. Antimicrob. Agents Chemotherapy 44:3364–3367.
- S. Y. Shin, H. N. Park, K. H. Kim, M. H. Lee, Y. S. Choi, Y. J. Park, Y. M. Lee, Y Ku, I. C. Rhyu, S. B. Han, S. J. Lee, and C. P. Chung. (2005). Biological evaluation of chitosan nanofiber membrane for guided bone regeneration. J. Periodontol. 76:1778–1784.
- R. Signini, and S. P. Campana. (1999). On the preparation and characterization of chitosan hydrochloride. Polym. Bull. 42:159–166.
- H. Takeuchi, Y. Matsui, H. Yamamoto, and Y. Kawashima. (2003). Mucoadhesive properties of carbopol or chitosan-coated liposomes and their effectivess in the oral administration of calcitonin to rats. J. Control. Rel. 86:235–242.
- R. Tarsi, R. A. A. Muzzarelli, C. A. Guzman, and C. Pruzzo. (1997). Inhibition of Streptococcus mutans adsorption to hydroxyapatite by low-molecular-weight chitosans. J. Dental Res. 76:665–672.
- M. Wainwright. (1998). Photodynamic antimicrobial chemotherapy (PACT). J. Antimicrob. Chemotherapy. 42:13–28.
- S. Wood, B. Nattress, J. Kirkham, R. Shore, S. Brookes, J. Griffiths, and C. Robinson. (1999). An in vitro study of the use of photodynamic therapy for the treatment of natural oral plaque biofilms formed in vivo. J. Photochem. Photobiol. 50:1–7.