Abstract
To improve delivery efficiency of anastrozole, we applied dendrimer-based stealth nanoparticles to encapsulate anastrozole to formulate stealth anastrozole nanoparticles. This work demonstrated that stealth nanoparticles composed of a PAMAM dendrimers core and a PEG layer could encapsulate anastrozole, hence causing improved water solubility of anastrozole. Anastrozole encapsulation depended on concentration of stealth nanoparticles and generation of dendrimer. The extended release of anastrozole was achieved. We envisioned that this highly structurally adaptable stealth nanoparticle could be further biofunctionalized to construct a targeted therapeutic delivery system for breast cancer treatment.
Anastrozole (2,2′-[5-(1H-1,2,4-triazol-1-ylmethyl)-1.3-phenylene] bis(2-methylpropionitrile)), commercially known as Arimidex, is used to treat postmenopausal women who are estrogen-receptor positive and need hormone-sensitive breast cancer treatment (CitationMareck et al. 2006). Anastrozole is commonly administered in the oral pill form. Arimidex pills contain 1 mg of anastrozole and the recommended dosage is 1 mg tablet per day for a treatment period lasting for about 31 months (CitationZidan et al. 2006). The problems associated with oral pill delivery of anti cancer drugs such as anastrozole are that they have low solubility in water, a short half-life, and uncontrolled delivery and release. In particular, anastrozole has an aqueous solubility of 0.5 mg/mL at 25°C and a half-life of ∼50 hr. To improve the treatment effectiveness of anastrozole, higher solubility and prolonged circulation of anastrozole are highly desired. Polymer-based drug delivery systems such as microparticles, microspheres, and micelles are under extensive investigation for enhancing drug efficacy. The first attempt to deliver anastrozole with polymer delivery system was reported recently. Researchers formulated biodegradable poly (d,l-lactic-co-glycolic acid) (PLGA)-based anastrozole microparticles (CitationZidan et al. 2006). They found that the anastrozole microparticles not only gained improved capacity to encapsulate more anastrozole molecules, but also generated exterior surface groups for ligand conjugation. Encapsulated anastrozole by PLGA microparticles achieved prolonged release for 40 days.
In recent studies, nanoscale polyamidoamine (PAMAM) dendrimers have emerged as the new face of drug carriers. PAMAM dendrimer consists of a hydrophobic initiator core and multiple hydrophilic surface groups on the exterior (CitationYang and Kao 2006). The diameter of these dendrimers ranges from 1.5 nm to 14.5 nm, depending on the generation of the dendrimer. Higher generations of dendrimer have greater encapsulation capacity (CitationKojima et al. 2000). By entrapping hydrophobic compounds inside a nanoscale dendrimer, one can dramatically increase solubility and delivery efficiency of poorly water-soluble drugs (CitationNeerman 2007; CitationMilhem et al. 2000). Due to a number of highly functional surface groups, drugs (CitationYang and Lopina 2005, Citation2003, Citation2007), or functional entities of interest (CitationYang and Lopina 2006) can be conjugated to the dendrimer for enhancing drug payload or achieving targeted delivery. Recently stealth dendrimers prepared by attaching poly-ethylene glycol (PEG) chains to the dendrimer surface has proved to be an efficient way to strengthen drug encapsulation capability of dendrimers and confer PEG's unique stealth properties to the nanoparticle () (CitationKojima et al. 2000; CitationSideratou et al. 2001). Further, stealth nanoparticles having a PEG shielding layer have improved in vivo circulating half-life by avoiding phagocytosis, thus promising a long-term drug treatment. Our previous study has demonstrated that PEG length affects drug encapsulation efficiency and can be optimized for drug loading (CitationYang et al. 2004).
FIG. 1. Schematic of a stealth nanoparticle composed of a G3.0 PAMAM dendrimer core and a PEG shielding layer.
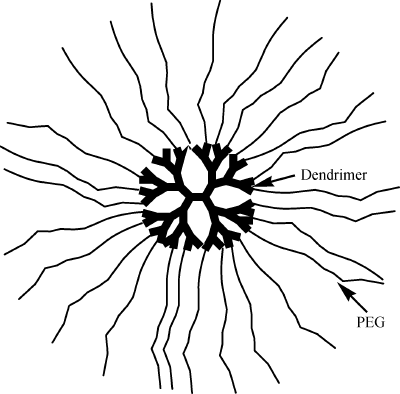
Given the compelling structural advantages of stealth nanoparticles, for the first time we applied stealth dendrimers to formulate anastrozole nanoparticles to improve water solubility and delivery efficiency of anastrozole. We envisioned that this highly structurally adaptable stealth nanoparticle could be further biofunctionalized to construct a targeted therapeutic agent for breast cancer treatment. Nonetheless, drug encapsulation efficiency and release kinetics are critical for such a stealth nanoparticle-based targeted breast cancer delivery system. Therefore, we attempted to demonstrate the feasibility of using stealth nanoparticles to encapsulate and release anastrozole in a controlled manner in this work.
MATERIALS AND METHODS
Starburst™ PAMAM dendrimer G3.0 and G4.0, methoxy PEG (i.e., MPEG, MW = 6000), triethylamine, 4-nitrophenyl chloroformate, tetrahydrofuran (THF), diethyl ether, and N,N-dimethylformamide (DMF) were all purchased from Sigma-Aldrich (St Louis, MO, USA). Anastrozole was obtained from Chemagis USA (Mountain Lakes, NJ, USA).
Synthesis of PEG 4-Nitrophenyl Carbonate
PEG 4-nitrophenyl carbonate was prepared via the reaction of PEG6000 with 4-nitrophenyl chloroformate following our previous work (CitationYang et al. 2004). First, 2000 mg of MPEG6000 were dissolved in 2 mL of THF. Then, to this solution, 50 μ L of triethylamine (7.2 mmol) and 80.6 mg of 4-nitrophenyl chloroformate (7.2 mmol) were added. The addition of these two components turned the solution into a translucent yellow color. Then, 1 mL of THF was added to the solution to ensure the dissolution of all the solutes. This was followed by stirring for 24 hr at room temperature on a stirring plate. After 24 hr of stirring, the mixture was pipetted evenly into 2-mL centrifuge tubes and centrifuged for 5 min at 10,000 rpm.
Then, the PEG6000 supernatant was added dropwise in a vial containing 20 mL of diethyl ether. The addition of the supernatant to diethyl ether caused a high degree of precipitation. The ether solution with the precipitate was evenly pipetted into 2-ml centrifuge tubes and centrifuged for 5 min at 10,000 rpm. After centrifugation, the supernatant was discarded and the pellet was allowed to air dry overnight. The product obtained was 147.7 mg of PEG6000 4-nitrophenyl carbonate. The same procedure was repeated and another 146.5 mg of PEG6000 4-nitrophenyl carbonate obtained.
Synthesis of PEG-PAMAM Stealth Nanoparticles
MPEG was covalently attached to PAMAM dendrimer to synthesize stealth nanoparticles (CitationYang et al. 2004). Briefly, a 1:1 ratio was maintained between the dendrimer (amine group base) and MPEG6000. Briefly, 2 mL of N,N-dimethylformamide solution was used to dissolve 30 μ L of dendrimer stock solution (contains 0.75 mol G3.0 PAMAM dendrimer) along with 144 mg of PEG6000 4-nitrophenyl carbonate(∼0.75 mol PEG6000). The solution was stirred for 72 hr at room temperature. After the reaction, the solution was added dropwise evenly into 3 vials, each containing 16 mL of diethyl ether. The addition of the solution to diethyl ether caused a high degree of precipitation. After centrifugation, the supernatant was discarded and the pellet was air-dried.
The product obtained was 91.8 mg of PEG-G3.0 stealth nanoparticles. This procedure was repeated using 65.63 μ L of stock solution (containing 0.375 mol G4 PAMAM dendrimer), 144 mg (0.375 mol PEG6000 for 64 amine groups) of PEG6000 4-nitrophenyl carbonate, and 2 mL of N,N-dimethylformamide solution. The product obtained was 92 mg of PEG-G4.0 stealth nanoparticles.
Encapsulation and Release of Anastrozole
Excess amounts of anastrozole were vigorously vortexed with stealth nanoparticles and equilibrated overnight at room temperature in order for stealth nanoparticles to maximally encapsulate anastrozole inside, while maintaining a saturated anastrozole concentration in the bulk aqueous phase. Saturated anastrozole solutions in the presence of stealth nanoparticles were obtained through syringe filtration with a 0.45 μ m PTFE filter. The final concentrations of stealth nanoparticles were 5 mg/mL, 10 mg/mL, and 20 mg/mL.
The release kinetics of anastrozole from stealth nanoparticles at room temperature was measured using UV-visible spectrometry. Solutions containing encapsulated anastrozole by stealth particles (100 μ L) were mixed in with 900 μ L of deionized water in a cuvette to create a 1:10 dilution. The absorbance of anastrozole was determined at a wavelength of 265 nm. The absorbance of the same solution was recorded at 1-min intervals for the first 15 min. Then, absorbance was recorded at 30 min, 1 hr, 12 hr, 1 day, and 2–5 days. Solutions containing nanoparticles of varying concentrations, without anastrozole, were used as blank solutions in the analysis.
RESULTS
We chose low generation Starburst™ G3.0 and G4.0 PAMAM dendrimers as the underlying core to prepare stealth nanoparticles. G3.0 and G4.0 have 32 and 64 surface amine groups, respectively. Both generations have diameters between 3 nm and 5 nm. Methoxy-capped PEG was employed in the synthesis to eliminate the chances of forming loops and cross-links on the dendrimer surface. PEG chains were covalently attached to the dendrimer via carbamate bonds. G3.0- and G4.0-based stealth nanoparticles were prepared following our previous work (CitationYang et al. 2004), from which more details regarding synthesis and characterization of this type of stealth nanoparticle can be found.
PEG-G3.0 stealth nanoparticles displayed concentration-dependent drug encapsulation ability (). Accumulatively released anastrozole in the 1-mL solution containing 20 mg of nanoparticles reached a plateau of 0.45 mg at ∼2 days. Similarly at 2 days, accumulatively released anastrozole reached a plateau of 0.13 mg by 5 mg of PEG-G3.0 nanoparticles and 0.24 mg by 10 mg of PEG-G3.0 nanoparticles, respectively. Extended release of anastrozole by PEG-G4.0 stealth nanoparticles lasted 2 days, after which a constant drug concentration was reached. More specifically, an overall amount of 0.37 mg, 0.57 mg, and 1.21 mg of anastrozole was encapsulated and released by 5 mg, 10 mg, and 20 mg of PEG-G4.0 in 1 mL of water over 5 days, respectively ().
FIG. 2. Accumulative release of anastrozole by various amount of PEG-G3.0 stealth nanoparticles in 1 mL of water.
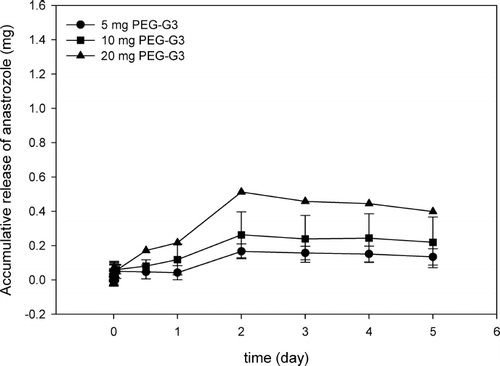
FIG. 3. Accumulative release of anastrozole by various amount of PEG-G4.0 stealth nanoparticles in 1 mL of water.
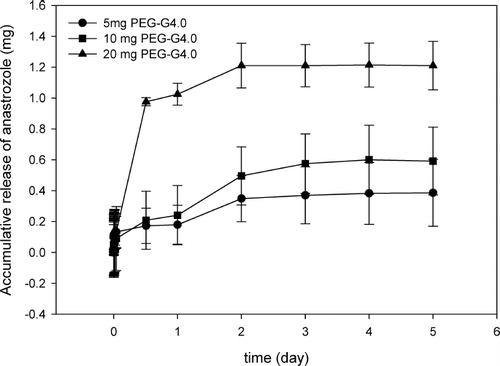
These results confirmed that stealth nanoparticles have the capacity to encapsulate anastrozole molecules inside, and stealth nanoparticles at higher concentrations intend to entrap more anastrozole molecules. In addition to concentration dependency, at the same amount G4.0-based stealth nanoparticles encapsulated more anastrozole than G3.0-based stealth nanoparticles. For example, the amount of encapsulated anastrozole by 20 mg of PEG-G4.0 was 2.7 times that of encapsulated anastrozole by the same amount of PEG-G3.0, indicating higher generation dendrimers have greater capacity to encapsulate anastrozole.
DISCUSSION
Anastrozole concentration in water was determined by measuring the absorbance at the wavelength of 265 nm with a UV-Vis spectrophotometer. In the UV-Vis spectroscopy tests, G3.0 and G4.0 PAMAM dendrimers displayed an absorption peak below 300 nm, and stealth nanoparticles displayed an adsorption peak at 280 nm. The presence of stealth nanoparticles interfered with anastrozole's absorption peak at 254 nm, thus making it difficult to quantify the actual concentration of anastrozole in the presence of stealth nanoparticles. We proposed a simple solution to resolve this problem by encapsulating drugs into stealth nanoparticles in saturated anastrozole solutions. As saturated anastrozole solutions without stealth nanoparticles remained a constant concentration due to the saturation effect, increase or decrease in drug concentration was directly attributed to the encapsulation or release by stealth nanoparticles. Since the bulk water phase was saturated with anastrozole, released anastrozole came out of the bulk solution, leading to the concentration deduction, which was instantly monitored by UV-Vis spectroscopy. As a result, the amount of anastrozole being released could be easily calculated based on the absorbance decrease of the solution.
Our work demonstrated the feasibility of using stealth nanoparticles composed of a PAMAM dendrimers core and a PEG layer to encapsulate anastrozole, hence causing improved water solubility of anastrozole. Anastrozole encapsulation depended on concentration of stealth nanoparticles and generation of dendrimer. The extended release of anastrozole was achieved. The highly adaptable stealth dendrimers allow for assembly of multiple functional entities on the surface. This study paved the way for us to explore a new targeted breast cancer drug delivery system based on the formulated stealth anastrozole nanoparticles.
The authors thank Chemagis USA Inc (Mountain Lakes, NJ, USA) for anastrozole donation. This research was supported in part by the A.D. Williams Foundation, the Jeffress Memorial Trust, and a new faculty startup fund from the School of Engineering of Virginia Commonwealth University.
REFERENCES
- C. Kojima, K. Kono, K. Maruyama, and T. Takagishi. (2000). Synthesis of polyamidoamine dendrimers having poly(ethylene glycol) grafts and their ability to encapsulate anticancer drugs. Bioconj. Chem. 11:910–917.
- U. Mareck, H. Geyer, S. Guddat, N. Haenelt, A. Koch, M. Kohler, G. Opfermann, M. Thevis, and W. Schanzer. (2006). Identification of the aromatase inhibitors anastrozole and exemestane in human urine using liquid chromatography/tandem mass spectrometry. Rapid Commun. Mass Spectrom 20:1954–1962.
- O. M. Milhem, C. Myles, N. B. McKeown, D. Attwood, and A. D'Emanuele. (2000). Polyamidoamine Starburst dendrimers as solubility enhancers. Int. J. Pharm. 197:239–241.
- M. F. Neerman. (2007). The efficiency of a PAMAM dendrimer toward the encapsulation of the antileukemic drug 6-mercaptopurine. Anticancer Drugs 18:839–842.
- Z. Sideratou, D. Tsiourvas, and C. M. Paleos. (2001). Solubilization and release properties of PEGylated diaminobutane poly(propylene imine) dendrimers. J. Colloid Interface Sci. 242:272–276.
- H. Yang, and W. J. Kao. (2006). Dendrimers for pharmaceutical and biomedical applications. J. Biomater. Sci. Polym. Ed. 17:3–19.
- H. Yang, and S. T. Lopina. (2005). Extended release of a novel antidepressant, venlafaxine, based on anionic polyamidoamine dendrimers and poly(ethylene glycol)-containing semi-interpenetrating networks. J. Biomed. Mater. Res. 72A:107–114.
- H. Yang, and S. T. Lopina. (2003). Penicillin V-conjugated PEG-PAMAM star polymers. J. Biomater. Sci. Polym. Ed. 14:1043–1056.
- H. Yang, and S. T. Lopina. (2007). Stealth dendrimers for antiarrhythmic quinidine delivery. J. Mater. Sci. Mater. Med. 18:2061–2065.
- H. Yang, and S. T. Lopina. (2006). In vitro enzymatic stability of dendritic peptides. J. Biomed. Mater. Res. 76A:398–407. Part A
- H. Yang, J. J. Morris, and S. T. Lopina. (2004). Polyethylene glycol-polyamidoamine dendritic micelle as solubility enhancer and the effect of the length of polyethylene glycol arms on the solubility of pyrene in water. J. Colloid Interface Sci. 273:148–154.
- A. S. Zidan, O. A. Sammour, M. A. Hammad, N. A. Megrab, M. D. Hussain, M. A. Khan, and M. J. Habib. (2006). Formulation of anastrozole microparticles as biodegradable anticancer drug carriers. AAPS PharmSciTech 7:61.