Abstract
Self-emulsifying drug delivery systems (SEDDS) are mixtures of oils, surfactants, and cosurfactants, which are emulsified in aqueous media under conditions of gentle stirring and digestive motility that would be encountered in the gastrointestinal tract. We found that SEDDS could efficiently improve oral absorption of the sparingly soluble drugs by rapid self-emulsification and subsequently dispersion in the absorption sites. Ginkgo biloba extract (GBE) has become a widely used herbal remedy for increasing cognitive function in elderly people. The main purpose of our work is to prepare SEDDS for improving oral absorption of GBE. Pseudoternary phase diagrams were constructed to identify the efficient self-emulsification region, and particle size distributions of resultant emulsions were determined. The optimized formulation for bioavailability assessment consisted of 45% Tween 80-Cremophor EL35 (1:1, w/w), 10% 1, 2-propanediol, and 45% ethyl oleate. The mean droplet size distribution of the optimized SEDDS was ∼100 nm when diluted with 500-fold volume of the distilled water. The in vitro dissolution rates of the active components of GBE SEDDS form were significantly faster than those of the GBE tablets. After single oral administration of 800 mg GBE as SEDDS or tablets to fasted dogs, the relative bioavailability of SEDDS for bilabolide and ginkgolide A and B was 162.1, 154.6, and 155.8% compared with the reference tablets, respectively. Our results suggested the potential and promising use of SEDDS for the efficient delivery of the sparingly soluble drugs or traditional Chinese medicines, such as GBE by oral administration.
Self-emulsifying drug delivery systems (SEDDS) are defined as isotropic mixtures of natural or synthetic oils, solid or liquid surfactants, or alternatively, one or more hydrophilic solvents and cosolvents/surfactants (CitationCharman et al. 1992; CitationCraig et al. 1993; CitationGershanik and Benita 2000; CitationGursoy and Benita 2004; CitationShah 1994). On mild agitation followed by dilution in aqueous media, such as gastrointestinal (GI) fluids, these systems can form fine oil-in-water (o/w) emulsions or microemulsions (SMEDDS). Self-emulsifying formulations spread readily in the GI tract, and the digestive motility of the stomach and the intestine provides enough agitation for the spontaneity of emulsion formation (CitationShah 1994). In comparison with sensitive and metastable emulsions, SEDDS are physically stable formulations that are easy to manufacture. Thus, for the sparingly soluble drugs that exhibit dissolution rate-limited absorption, the SEDDS system offers a way to improve the rate and extent of oral absorption and to elicit the more reproducible oral absorption behavior (CitationGursoy and Benita 2004).
Ginkgo biloba L. is the oldest living tree on earth, dating back to the Paleozoic period, over 225 million years ago. The medicinal use of ginkgo leaf is first mentioned in Chinese medicine in the Ming dynasty in 1436 (CitationFoster 1996). A standardized extract of ginkgo leaf is one of the most clinically tested and frequently prescribed phytomedicines in Europe and has been one of the 10 best-selling herbal dietary supplements in the USA for about 6 years (CitationBlumenthal 2001; CitationBlumenthal 1998). The primary active components of GBE include 5–7% terpene lactones (ginkgolides and bilobalide) and 22–27% ginkgo flavonol glycosides (e.g., the flavones quercetin, kaempferol, and isorhamnetin) (CitationKressmann 2002a).Ginkgo biloba extract (GBE) is approved in Germany for the treatment of cerebral insufficiency (memory loss that occurs with conditions such as Alzheimer's disease, vascular or multiinfarct dementia, and other conditions), tinnitus (ringing in the ears), vertigo, and intermittent claudication (poor circulation to the lower legs). In the USA, ginkgo is used widely as a complementary therapy for Alzheimer's disease and vascular dementia (CitationOken et al. 1998).
The dissolution and bioavailability of the active components from the oral solid preparations of different Ginkgo biloba brands were significantly different and irreproducible, due to the lower solubility and varying dissolution of its active components (CitationKressmann 2002a, Citation2002b). In the present study, the SEDDS formulation of GBE was accordingly developed to improve dissolution and oral absorption and to acquire the reproducible blood-time profiles of the active components of GBE.
MATERIALS AND METHODS
GBE was supplied by Zhejiang Conba Pharm. Co., (Lanxi, Zhejiang, China) and the internal standard, daidzein, by Shanxi Huike Botanical Development Co., (Xi'an, Shanxi, China). Bilabolide (BB), ginkgolide A (GG-A), ginkgolide B (GG-B), and ginkgolide C (GG-C) were isolated and characterized by Hefei Tuofeng Development Co. (Hefei, Anhui, China). HPLC-grade methanol, diethyl ether, dichloromethane, and isopropanol were secured from Concord Tech. (Tianjin, China). Glycerol and 1, 2-propanediol were purchased from Tianjin Bodi Chemical Co. (Tianjin, China). Tween 80 was from Beijing Yili Fine Chemical Co. (Beijing, China). Cremophor EL35 was a gift from BASF Corp. (Mount Olive, NJ, USA). Miglyol 812 was form Condea Chemie GmbH (Witten, Germany). All other reagents were of analytical grade. Distilled water, prepared from deionized water, was used throughout the study.
The GBE tablets were prepared using the same GBE raw material. GBE tablets were prepared by direct compression, each tablet consisting of 40 mg GBE, 160 mg starch, and 200 mg Avicel PH102. The disintegration time of GBE tablets was less than 20 min.
Solubility of GBE
An excess amount of GBE was added to various oils, surfactants, and cosurfactants and mixed by vortexing. The mixture was then kept at 25°C in an air oscillator for 7 days to reach equilibrium. The equilibrated sample was centrifuged at 15000 r/min for 10 min to remove the undissolved GBE. The supernatant was collected and diluted with methanol for quantification of the flavone glycosides by HPLC and the terpene lactones by liquid chromatography with electrospray ionization mass spectrometric detection (LC-ESI-MS).
The determination of the flavone glycosides by HPLC as described by CitationYang et al. (2004) was adopted and modified in the present study. Briefly, 3 ml of the filtered samples were dissolved in 12 ml of methanol-25% HCl (4:1, v/v) and refluxing heated in a boiling water bath for 30 min to hydrolyze the glycosides. Then the mixed solution was transferred into 25 ml of volumetric flask and diluted to the scale using methanol. The resulting aglycones, quercetin, kaempferol, and isorhamnetin, were separate on an RP-C18 column (DiamondsilTM, 200 × 4.6 mm, 5 μm; Dikma, Beijing) using methanol-0.4% phosphoric acid (50:50, v/v) as mobile phase. The detection was performed at 360 nm for quercetin, kempferol, and isorhammetin.
Because of the calculative conversion to flavonol glycosides with an average molecular weight of MR = 756.7, the total content of the flavone glycosides were calculated by the resulting content of corresponding aglycone multiplied by 2.51. Calibration curves (six concentrations) were linear over the range of 0.2–2 μg/ml for the quercetin, kempferol, and isorhamnetin. The coefficient of determination was > 0.999 in all cases. Mean accuracy and precision (3-day validation) were < 6 and < 9%, respectively. The lower limit of quantification (LLOQ) was confirmed to be ∼0.2 μg/ml for quercetin, kempferol, and isorhamnetin. Accuracy and precision at LLOQ were < 7 and 10%, respectively. A recovery of between 94 and 107% was calculated for the three substances.
The amount of the terpene lactones was determined by LC-ESI-MS. Optimum chromatographic separation was achieved on a 150 × 3.9 mm, particle size 5 μm Symmetry C18 column (Waters Corp., Milford, MA, USA) at a column temperature of 25°C. The mobile phase consisted of methanol and water (60:40, v/v) at a flow rate of 0.3 mL/min and the injection volume was 20 μl. The chromatographic system consisted of a Waters 1525 pump controlled by Masslynx 4.0 software (Waters Corp.). A ZQ 2000 micromass spectrometer (Waters Corp.) fitted with a Z-Spray ion interface was used for all analyses. Ionization was achieved using electrospray in the negative ionization mode.
The following parameters were optimized for the analysis of terpene lactones: capillary voltages, 3.0 kV; cone voltages, 30 V; source temperature, 105°C; and desolvation gas (nitrogen) heated to 180°C and delivered at a flow rate of 350 L/hr. Quantification was performed using selected ion recording (SIR) of m/z 325 for BB, m/z 407 for GG-A, m/z 423 for GG-B, m/z 439 for GG-C. Calibration curves (six concentrations) were linear over the range of 0.1–5.0 μg/ml for BB, GG-A, GG-B, and GG-C. The coefficient of determination was > 0.999 in all cases. Mean accuracy and precision (3-day validation) were < 7 and < 12%, respectively. The LLOQ of the method was 0.1 μg/ml for BB, GG-A, GG-B, and GG-C. Accuracy and precision at LLOQ were < 6% and 12%, respectively.
Preparation of Self-Emulsifying Formulation
The GBE contents in the marketed formulations are 40 or 80 mg. A series of self-emulsifying formulations were prepared in each of the formulations () with varying concentrations of oil (5–65%), surfactant (30–70%), and consurfactant (5–25%). In the SEDDS, the content of GBE was fixed constant (12.5% w/w of the vehicle). Components of SEDDS (oil, surfactant, cosurfactant, and GBE) were accurately weighed into glass beaker and heated at 40°C in an air-oscillator until GBE dissolved. At the optimized formulation, the fill volume of a size 1 capsule containing 40 mg GBE was used for dissolution and bioavailability studies.
TABLE 1 Vehicle compositions of the designed SEDDS formulations
Visual Observations and Droplet Size Determination
A visual test to assess the self-emulsification properties reported by Craig and Kommuru et al. was modified and adopted in the present study (CitationCraig et al. 1995; CitationKommuru et al. 2001). Briefly, formulation (0.2 ml) was introduced into 100 ml of water in a glass beaker at 37°C, and the contents were blended gently by a magnetic stir bar. The tendency to emulsify spontaneously and also the progress of emulsion droplets were observed. The tendency to form an emulsion was judged as “good” when droplets spread easily in water and formed a fine emulsion that was clear or slightly opaque in appearance. It was judged “bad” when the corresponding performance was poor or there was poor emulsion formation of mixtures layered before adding to water. A phase diagram was constructed to identify the good self-emulsifying region. All studies were repeated in duplicate, with similar observations being made between repeats.
The droplet size and distribution of the resultant emulsions was determined using a LS230 particle size analyzer (Beckman Coulter, USA). The particle size analyzer can measure sizes from 40 nm to 2000 μm.
Dissolution Test
The dissolution test was performed in apparatus (ZRS-8G, Tianjin University Radio Factory, China) according to the third method of Chinese Pharmacopoeia dissolution procedure, as described previously (CitationTang et al. 2006a). Briefly, self-prepared tablets and SEDDS capsules of GBE were put into a sinker. This sinker was loaded with 100 ml of water at 37 ± 0.5°C with paddle speed of 100 r/min. Each sample (4 ml) was withdrawn at 5, 10, 20, 45, and 60 min and then an equal volume of temperature-equilibrated blank media was added into the beaker. The samples were filtered and 3 ml was used for determination of the amount of flavone glycosides to compare the dissolution behavior of the active components of the SEDDS capsules or tablets. In addition, SEDDS capsules or tablets dissolved in water served as reference, respectively, of 100% dissolution control. The quantification procedure of the flavone glycosides by HPLC and the terpene lactones by LC-ESI-MS is described above.
Animal Studies
Experimental protocols were approved by the Animal Care Committee of Shenyang Pharmaceutical University. Six healthy dogs were obtained from the Laboratory Animal Center of Shenyang Pharmaceutical University (Shenyang, Liaoning, China). Their weight ranged from 20 to 28 kg. Randomized, open label, two-period cross-over, single-dose experiment with a washout period of 1 week was designed. GBE SEDDS or tablets was given to six fasted dogs by intragastric administration at the dosage of 800 mg. Blood samples (∼3 ml) were collected into heparinized tubes at 0, 0.5, 0.75, 1, 1.25, 1.5, 2, 3, 4, 5, 6, 8, and 10 hr after drug administration. The plasma samples were separated by centrifugation at 3000 × g for 10 min and stored at −20°C until analysis. The concentration of ginkgolides and bilobalide was determined by LC-ESI-MS (CitationTang et al. 2006b). LC-ESI-MS conditions were as described above. Quantification was performed using selected ion recording of m/z 325 for BB, m/z 407 for GG-A, m/z 423 for GG-B, m/z 439 for GG-C, and m/z 253 for daidzein.
An aliquot of 100 μl mobile phase and 100 μl daidzein (10 ng/ml in mobile phase) were added to a 200 μl plasma sample in screw-cap glass tubes. The mixture was vortexed for 30 sec and extracted with 3 ml diethyl ether-dichloromethane-isopropanol (6:3:1, v/v). After vortex-mixing for 2 min and centrifugation at 3500 × g for 10 min, the organic layer was separated and evaporated to dryness at 40°C under a gentle stream of nitrogen. The residue was reconstituted in 100 μl mobile phase followed by vortex-mixing. A 20 μl aliquot of the supernatant was injected onto the LC-MS system. The method showed excellent reproducibility with intraday and interday precision of less than 12.3% (R.S.D.), as well as excellent accuracy with inter and intra-assay accuracy between 97.1 and 106.3%. The lower limit of quantitation of BB, GG-A, and GG-C was 10.0 ng/ml and that of GG-B was 2.5 ng/ml with precision less than 13.2% and accuracy less than 109%.
Pharmacokinetic Data Analysis
The chromatographic data were automatically processed to obtain the peak-area ratios of compound to the internal standard and fitted to a weighed (1/C2) linear regression relationship. The maximum plasma concentration (Cmax) and the time to reach this maximum concentration (Tmax) were determined by visual inspection of the experiment data. The elimination rate constant (Ke) was calculated by applying the least-squares regression technique to the data for the last four points of the plasma concentration-time curve, and the half-life (t1/2) of the drug was obtained by 0.693/Ke.
The data are presented as mean with standard error (SE) for the individual groups. An unpaired Student's t-test was used to determine any significant differences. Differences were considered to be significant at p < 0.05.
RESULTS AND DISCUSSION
Vehicles Selection
Ginkgolides are soluble in acetone, ethanol, methanol, ethyl acetate, tetrahydrofuran, dioxane, acetic acid, trifluoroacetic acid, acetonitrile, pyridine, and dimethyl sulfoxide. They are sparingly soluble in ether and water. The solubility data of bilabolide are not available in the literature but its polarity and solubility appears similar to those of the ginkgolides. A log P value of 1.72 was reported for ginkgolide B (CitationVan Beek 2005). The self-emulsifying formulations consisted of oil, surfactants, cosolvent, and drug, and should be a clear and homogeneous liquid at ambient temperature and should have good solvent properties to allow presentation of the drug in solution (CitationKang et al. 2004). Drug amount loaded in each formulation is a critical factor, dependent on the drug is apparent solubility in a variety of formulation vehicles. In addition, the volume of the formulation should be minimized in to deliver the therapeutic dose of the drug in an encapsulated form.
Vehicles selected for the formulation should have the ability to solubilize the drug to a great extent to obtain a concentrative form of the emulsions. GBE solutions appear brown after dissolution, so the amount of soluble GBE in various vehicles could be estimated by visual observations. GBE had poor solubility in oils and surfactants, so the data of solubility in these vehicles were not determined. GBE had higher solubility in 1, 2-propanediol and glycerol than other vehicles (). The 1, 2-propanediol was finally chosen as the cosolvent because the viscosity of glycerol is too high to disperse. Tween 80 or Tween 80-Cremophor EL 35 (1:1) was chosen as the surfactant because it has good emulsifying efficiency and good miscibility with 1, 2-propanediol.
TABLE 2 Solubility of Ginkgo biloba extracts in various vehicles at 25°C (n = 3, mg/g)
Visual Observations and Droplet Size Analysis
The visual test is a measure of the apparent spontaneity of emulsion formation. A series of SEDDS were prepared and their self-emulsifying properties were observed via naked eyes. A pseudoternary phase diagram was constructed to identify the self-emulsifying regions and also to acquire the optimum levels of oil, surfactant, and cosurfactant. The phase diagrams of the systems containing Tween 80, 1, 2-propanediol, and different oils (Miglyol 812 and Ethyl oleate) are shown in . The phase diagrams of the systems containing Tween 80-Cremophor EL35 (1:1), 1, 2-propanediol, and different oils (Miglyol 812 and ethyl oleate) are shown in . For both systems, the self-emulsifying region is larger when ethyl oleate is used as oil than Miglyol 812 as oil.
FIG. 1 Pseudoternary phase diagrams indicating the efficient self-emulsification region with Miglyol 812 as oil. (A) Tween 80 as surfactant; (B) Tween 80-Cremophor EL 35 (1:1) as the mixed surfactants (Key: The region of efficient self-emulsification is bound by the solid line; and the filled squares represent the composition evaluated.)
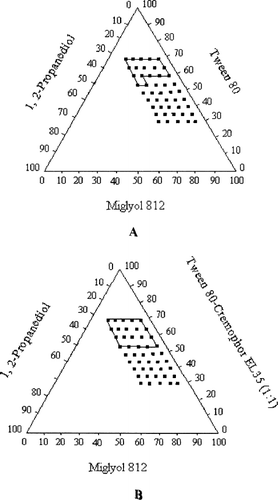
FIG. 2 Pseudoternary phase diagrams indicating the efficient self-emulsification region with ethyl oleate as oil. (A) Tween 80 as surfactant; (B) Tween 80-Cremophor EL 35 (1:1) as the mixed surfactants (Key: The region of efficient self-emulsification is bound by the solid line; and the filled squares represent the composition evaluated.)
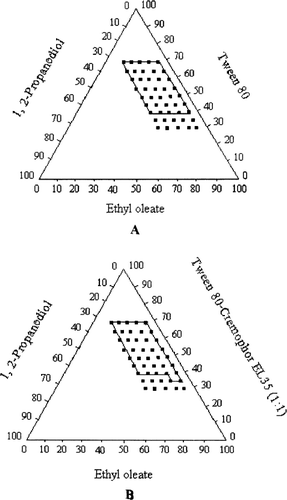
The mixed surfactants, Tween 80-Cremophor EL35 (1:1), increased the self-emulsifying region slightly larger than Tween 80, but it significantly reduced the particle size. Therefore, the mixed surfactants were used as the optimized emulsifier. The result is in accordance with the results previously reported by other authors (CitationLi et al. 2005; CitationEngels et al. 1995). The combined use of Tween 20 and Cremophor EL showed marked advantages over the single use of either surfactant. The microemulsion region was greatly increased in the phase diagram and the oil compositions also were enlarged so that high drug load became possible (CitationLi 2005). The combined use of Tween 80 and soybean lecithin was found to markedly increase the oil level in the microemulsions by 3-fold (CitationEngels et al. 1995).
The results in this study seemed to indicate that mixed surfactant might provide a better hydrophilic-lipophilic balance for self-emulsion process, because it enhances the flexibility of surfactant layer that was formed and also increases the surfactants' ability to partition at higher levels into the oil-water interface (CitationEngels et al. 1995; CitationMoreno et al. 2003; CitationNinham et al. 1984; CitationWarisnoicharoen et al. 2000; CitationWeingarten et al. 1991). In this study, increasing the surfactant concentration (from 30 to 70%) enhanced the spontaneity of the self-emulsification process and decreased the mean droplet size. This may be due to excess penetration of water into the bulk oil causing massive interfacial disruption and ejection of droplets into the bulk aqueous phase (CitationPouton 1997). More surfactant can stabilize the oil-water interface, thus resulting in a decrease in droplet size. Furthermore, the decrease in the mean droplet size reflects the formation of a better close-packed film of the surfactant at the oil-water interface, thereby stabilizing the oil droplets (CitationLevy and Benita 1990).
We observed that increasing the concentration of the cosurfactant, 1, 2-propanediol, within the self-emulsifying region increased the spontaneity of the self-emulsification process. When the cosurfactant is added to the system, it not only can dissolve drug, but also further lowers the interfacial tension between the oil and water interface and influences the interfacial film curvature, which thereby readily deforms around oil droplets (CitationKommuru et al. 2001).
Based on the in vitro self-emulsification properties, the formulation containing Tween 80-Cremophor EL35 (1:1) (45%), 1, 2-propanediol (10%), and ethyl oleate (45%) was selected for the dissolution and bioavailability studies. The mean droplet size distribution of the optimized SEDDS was ∼100 nm when diluted with 500-fold volume of the distilled water.
In Vitro Dissolution Study
Dissolution studies were performed for SEDDS and the conventional self-prepared tablets. The dissolution rates of the active components in GBE from these dosage forms were evaluated in water. The release percentages of the active components of GBE from the SEDDS were significantly higher than those of GBE from the conventional tablets (). This result suggested GBE in the SEDDS from could be rapidly released due to the small droplet size and increased contact surface with dissolution media.
FIG. 3 Dissolution profiles of flavone glycosides, bilobalide, ginkgolide A, ginkgolide B, and ginkgolide C from SEDDS (•) and the conventional tablets (○). (A) Flavone glycosides; (B) bilabolide; (C) ginkgolide A; (D) ginkgolide B; (E) ginkgolide C. Tests were conducted in 100 ml of water at 37 ± 0.5°C with a rotation speed of 100 r/min. Each value is the mean ± SD (n = 3).
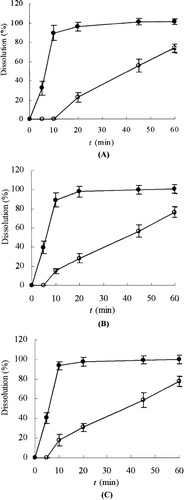
Bioavailability Studies
In view of flavonoids in the GBE preparations, their concentrations are too low to be measured by HPLC or LC-MS after oral single administration of 800 mg GBE to dogs, even after enzymatic hydrolysis of their conjugated metabolites to aglycon. Therefore, we mainly focused on the pharmacokinetic profiles of the terpene lactones (ginkgolides and bilobalide) after oral single administration of GBE to dogs.
The plasma profiles of ginkgolides and bilobalide in dogs following oral administration of the reference tablets and self-emulsifying formulation of GBE at a single dose of 800 mg GBE were determined. The plasma concentration profiles of BB, GG-A, and GG-B for SEDDS presented significantly greater improvement in oral absorption than the conventional tablets (, , ). The main pharmacokinetic parameters for each of the terpene lactones of two formulations are summarized in .
FIG. 4 Bilabolide plasma concentration-time profiles for either SEDDS (•) or tablets (○) following oral administration at a single dose of 800 mg GBE in dogs. Each value is the mean ± SE (n = 6).
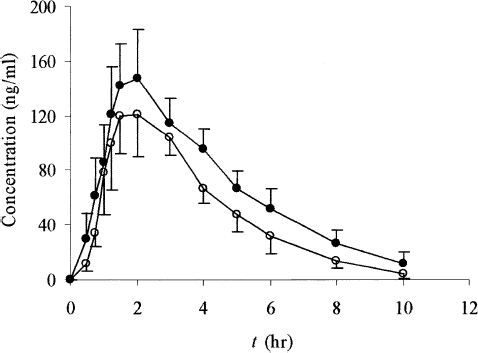
FIG. 5 Ginkgolide A plasma concentration-time profiles of either SEDDS (•) or tablets (○) following oral administration at a single dose of 800 mg GBE in dogs. Each value is the mean ± SE (n = 6).
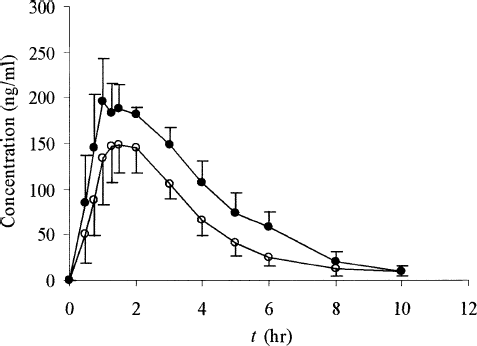
FIG. 6 Ginkgolide B plasma concentration-time profiles of either SEDDS (•) or tablets (○) following oral administration at a single dose of 800 mg GBE in dogs. Each value is the mean ± SE (n = 6).
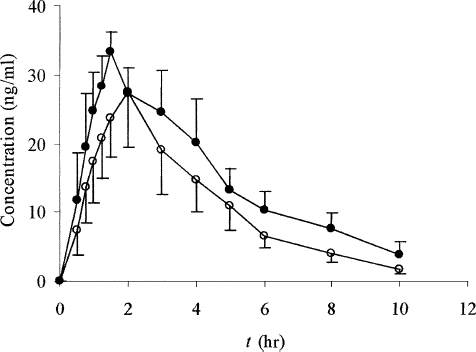
TABLE 3 Main pharmacokinetic parameters of bilabolide, ginkgolide A and ginkgolide B after a single oral dose of Ginkgobiloba extract 800 mg SEDDS and tablets in dogs
The small Tmax values of SEDDS for all three determined components demonstrated the fairly rapid onset compared with the conventional tablets. For bilobalide, the reduction in Tmax values from 2.33 ± 0.75 hr for tablets to 1.58 ± 0.34 hr for SEDDS was statistically significant (p < 0.05). For ginkgolide A, this difference was almost 1 hr (tablet: 2.42 hr; SEDDS: 1.50 hr, p < 0.05), and for ginkgolide B, Tmax values were 2.25 ± 0.88 hr for tablets and 1.46 ± 0.40 hr for SEDDS (p < 0.05). The marked differences also were observed for the Cmax. For bilobalide, the Cmax value was 201.19 ng/ml for SEDDS and 138.99 ng/ml for tablets; for ginkgolide A, it was 252.60 ng/ml versus 165.76 ng/ml; for ginkgolide B it was 44.89 ng/ml versus 31.09 for SEDDS and tablets (p < 0.05), respectively.
Moreover, for bilabolide, AUC0→10h values of 727.0 ng/ml·hr versus 466.9 ng/ml·hr for SEDDS and tablets, respectively, were found to be statistically significant different (p < 0.05). For ginkgolide A, the AUC0→10h for SEDDS was 839.9 ng/ml·hr and that for the tablets was 565.4 ng/ml·hr (p < 0.05). For ginkgolide B, the AUC0→10h value was 164.7 ng/ml·hr for SEDDS versus 104.6 ng/ml·hr for the tablets (p < 0.05). The relative bioavailabilities of SEDDS to tablets for bilobalide, ginkgolide A, and ginkgolide B were all in the range 154.6–162.1%. These data clearly demonstrated the promising utility of SEDDS in improving the rate and extent of oral absorption of active components in GBE.
CONCLUSIONS
GBE is a widely used herbal remedy for the treatment of cerebral insufficiency (such as Alzheimer's disease) in many countries. The active components of GBE are sparingly soluble, resulting in the dissolution and bioavailability of the active components from the oral solid preparations of different Ginkgo biloba brands significantly different and irreproducible. In the study, the use of SEDDS improved the dissolutions of the active components. Also, SEDDS showed significant greater extent of oral absorption than the conventional tablets based on the in vivo bioavailability studies. The relative bioavailability of SEDDS for bilabolide, ginkgolide A, and B was 162.1, 154.6, and 155.8%, respectively, compared with the reference tablets. In conclusion, for sparingly soluble drugs or traditional Chinese medicines, such as GBE, a significant improvement in reproducibility and bioavailability via oral administration might be achieved with SEDDS.
We are grateful for the financial support by the National High Technology Research and Development Program of China (863 Program, no. 2004AA2Z3250) and by the Education Department of Liaoning Province, no. 2004D266.
REFERENCES
- The Complete German Commission E Monographs—Therapeutic Guide to Herbal Medicines. M. Blumenthal, W. R. Busse, A. Goldberg, J. Gruenwald, T. Hall, C. W. Riggins, R. S. Rister, S. Klein, and R. S. Rister. American Botanical Council, AustinTX, (1998) 136–138. Boston, MA: Integrative Medicine Communication.
- M. Blumenthal. (2001). Herb sales down 15% in mainstream market. Herbal Gram. 51:69.
- S. A. Charman, W. N. Charman, M. C. Rogge, T. D. Wilson, F. J. Dutko, and C. W. Pouton. (1992). Self-emulsifying drug delivery systems: formulation and biopharmaceutic evaluation of an investigational lipophilic compound. Pharm. Res. 9 (1):87–93.
- D. Q. M. Craig, H. S. R. Lievens, K. G. Pitt, and D. E. Storey. (1993). An investigation into the physicochemical properties of self-emulsifying systems using low frequency dielectric spectroscopy, surface tension measurement and particle size analysis. Int. J. Pharm. 96 (1–3):147–155.
- D. Q. M. Craig, S. A. Barker, D. Banning, and S. W. Booth. (1995). Investigation into the mechanisms of self-emulsification using particle size analysis and low frequency dielectric spectroscopy. Int. J. Pharm. 114 (1):103–110.
- T. Engels, T. Förster, and W. V. Rybinsko. (1995). The influence of coemulsifier type on the stability of oil-in-water emulsions. Colloids. Surf. A: Physicochem. Eng. Aspects. 99 (2–3):141–149.
- S. Foster. Ginkgo-ginkgo biloba. American. Botanical. Council, AustinTX, (1996) Botanical Booklet No. 304..
- T. Gershanik, and S. Benita. (2000). Self-dispersing lipid formulations for improving oral absorption of lipophilic drugs. Eur. J. Pharm. Biopharm. 50 (1):179–188.
- R. N. Gursoy, and S. Benita. (2004). Self-emulsifying drug delivery systems (SEDDS) for improved oral delivery of lipophilic drugs. Biomed. Pharmacother. 58 (3):173–182.
- B. K. Kang, J. S. Lee, S. K. Chon, S. Y. Jeong, S. H. Yuk, G. Khang, H. B. Lee, and S. H. Cho. (2004). Development of self-microemulsifying drug delivery systems (SMEDDS) for oral bioavailability enhancement of simvastatin in beagle dogs. Int. J. Pharm. 274 (1–2):65–73.
- T. R. Kommuru, B. Gurley, M. A. Khan, and I. K. Reddy. (2001). Self-emulsifying drug delivery systems (SEDDS) of coenzyme Q10: formulation development and bioavailability assessment. Int. J. Pharm. 212 (2):233–246.
- S. Kressmann, W. E. Muller, and H. H. Blume. (2002a). Pharmaceutical quality of different Ginkgo biloba brands. J. Pharm. Pharmacol 54 (5):661–669.
- S. Kressmann, A. Biber, M. Wonnemann, B. Schug, H. H. Blume, and W. E. Muller. (2002b). Influence of pharmaceutical quality on the bioavailability of active components from Ginkgo biloba preparations. J. Pharm. Pharmacol. 54 (11):1507–1514.
- M. Y. Levy, and S Benita. (1990). Drug release from submicronized O/W emulsion: a new in vitro kinetic evaluation model. Int. J. Pharm. 66 (1–3):29–37.
- P. Li, A. Ghosh, R. F. Wagner, S. Krill, Y. M. Joshi, and A. T. Serajuddin. (2005). Effect of combined use of nonionic surfactant on formation of oil-in-water microemulsions. Int. J. Pharm. 288 (1):27–34.
- M. A. Moreno, M. P. Ballesteros, and P. Frutos. (2003). Lecithin-based oil-in-water microemulsions for parenteral use: pseudoternary phase diagrams, characterization and toxicity studies. J. Pharm. Sci. 92 (7):1428–1437.
- B. W. Ninham, S. J. Chen, and D. F. Evans. (1984). Role of oils and other factors in microemulsion design. J. Phys. Chem. 88 (24):5855–5857.
- B. S. Oken, D. M. Storzbach, and J. A. Kaye. (1998). The efficacy of Ginkgo biloba on cognitive function in Alzheimer disease. Arch. Neurol. 55 (11):1409–1415.
- C. W. Pouton. (1997). Formulation of self-emulsifying drug delivery systems. Adv. Drug. Del. Rev. 25 (1):47–58.
- N. H. Shah, M. T. Carvajal, C. I. Patel, M. H. Infeld, and A. W. Malick. (1994). Self-emulsifying drug delivery systems (SEDDS) with polyglycolyzed glycerides for improving in vitro dissolution and oral absorption of lipophilic drugs. Int. J. Pharm. 106 (1):15–23.
- J. L. Tang, J. Sun, F. D. Cui, and Z. G. He. (2006a). Preparation of self-emulsifying drug delivery systems of Ginkgo biloba extracts and in vitro dissolution study. Asian J. Trad. Med. 1 (3–4):138–141.
- J. L. Tang, J. Sun, Y. H. Sun, F. D. Cui, and Z. G. He. (2006b). LC-ESI-MS determination of bilobalide and ginkgolides in canine plasma. Chromatographia 63 (1):53–58.
- T. A. Van Beek. (2005). Ginkgolides and bilobalide: their physical, chromatographic and spectroscopic properties. Bioorg. Med. Chem. 13 (17):5001–5012.
- W. Warisnoicharoen, A. B. Lansley, and M. J. Lawrence. (2000). Nonionic oil-in-water micro-emulsions: the effect of oil type on phase behaviour. Int. J. Pharm. 198 (1):7–27.
- C. Weingarten, N. S. S. Magalhaes, A. Baszkin, S. Benita, and M. Seiller. (1991). Interaction of a nonionic ABA copolymer surfactant with phospholipid monolayers: possible relevance to emulsion stabilization. Int. J. Pharm. 75 (2–3):171–179.
- Y. J. Yang, Q. N. Ping, and Y. M. Song. (2004). Improvement of the dissolution rate of Ginkgo biloba tablets by using solid dispersion methods. Zhong. Gou. Xin. Yao. Za. Zhi. 13 (6):521–524.