Abstract
The aim of the present study was to utilize the sol-gel method to synthesize different forms of xerogel matrices for drugs and to investigate how the synthesis conditions and solubility of drugs influence the change of the profile of drug release and the structure of the matrices. Silica xerogels doped with drugs were prepared by the sol-gel method from a hydrolyzed tetraethoxysilane (TEOS) solution containing two model compounds: diclofenac diethylamine, (DD)—a water-soluble drug or ibuprofen, (IB)—a water insoluble drug. Two procedures were used for the synthesis of sol-gel derived materials: one-step procedure (the sol-gel reaction was carried out under acidic or basic conditions) and the two-step procedure (first, hydrolysis of TEOS was carried out under acidic conditions, and then condensation of silanol groups was carried out under basic conditions) in order to obtain samples with altered microstructures. In vitro release studies of drugs revealed a similar release profile in two steps: an initial diffusion-controlled release followed by a slower release rate. In all the cases studied, the released amount of DD was higher and the released time was shorter compared with IB for the same type of matrices. The released amount of drugs from two-step prepared xerogels was always lower than that from one-step base-catalyzed xerogels. One-step acid-catalyzed xerogels proved unsuitable as the carriers for the examined drugs.
INTRODUCTION
Oxides obtained via the sol-gel method can be applied in an assortment of ways, both in medicine and pharmaceutical business, as biocatalysts, biosensors, immunodiagnostics, and drug delivery systems (CitationDave et al. 1994; CitationWu, Lin, and Chan 1994; CitationBlyth, Poynter, and Russel 1996; CitationSieminska and Zerda 1996; CitationAhola et al. 2000; CitationLi et al. 2002; CitationNguyen et al. 2002; CitationRadin et al. 2002; CitationCzarnobaj and MLukasiak 2004; Citationde Gaetano et al. 2005; CitationCzarnobaj, Prokopowicz, and MLukasiak 2006; CitationCzarnobaj and Lukasiak (2007). Sol-gel derived silica xerogels are non-toxic and biocompatible in vivo. These materials cause no adverse tissue reactions and degrade in the body to silicic acid, i.e. Si(OH)4, which is eliminated through the kidneys (CitationKortesuo et al. 2000).
The sol-gel procedure is highly efficient due to the low temperature of the chemical process, high homogeneity, and products purity, which enable inserting some biologically active substances into the preparation.
The sol-gel process involves metal alkoxide precursors (e.g. TEOS—Si(OC2H5)4), ethanol and water. The hydrolysis and condensation of the liquid alkoxide leads to the formation of the solid oxide network (sol-gel transition) (CitationSchmidt 1988; CitationRo and Chung 1991).
Additives with therapeutic properties may be introduced to xerogels in the sol-gel process either at the stage of the formation of sol, or upon the formation of porous xerogel by superficial adsorption (CitationKlein 1993). The first method is particularly interesting, as not many other matrices offer such possibilities. Doping some admixture to a liquid sol and gelating it results in the occludation of a molecule in the formed bonds of the Si-O-Si network. A consequence of such an initiated immobilization mechanism is the fact that molecules of variable shapes, sizes, and electric charges may be trapped.
The type of catalytic agent (acid or base or both of them) determines the shape of polymer structure. The acid catalyst promotes hydrolysis and end-of-chain condensation, leading to the formation of a monolithic, microporous polymer with a small field of the surface proper and an average pore size less than 2 nm, whereas the basic catalyst facilitates the production of complex agglomerate consisting of branched colloidal particles, which results in a delivery of a mesoporous material (an average pore size in the range 2–50 nm), with high adsorptive capabilities. Two-step acid/base catalysis provides gels with properties intermediate to those of single acid or base catalyzed gels (CitationBuckley and Greenblatt 1994; CitationLivage and Sanchez 1994).
In this paper, Diclofenac, a water-soluble drug, and Ibuprofen, a water-insoluble drug, are chosen as the model drugs. Both the drugs belong to Non-Steroidal Anti-Inflammatory Drugs (NSAIDs). They are characterized with a strong anti-inflammatory and analgesic effect. They are administered in various pain conditions, including those which accompany rheumatic diseases. These drugs also proved efficient in relieving pains accompanying metastases to the bones (CitationFang et al. 1999; CitationKramar, Turk, and Vrečer 2003; CitationHeikkil et al. 2007; CitationYi and Zhang 2007).
The present study describes trapping of drugs into sol-gel processed silica xerogels during the matrix formation. Such a method of synthesis produces drug molecules that are dispersed homogeneously in solid matrix.
The delivery of the drug through a xerogel will lead to improved therapeutic activity, better protection against degradation, a change in pharmacokinetics, better control of bio-distribution, and decreased toxicity because of a slower, more constant drug release rate (CitationAhola et al. 2001; CitationRadin et al. 2002).
Two procedures were used for the synthesis of sol-gel derived materials. In a one-step procedure, the sol-gel reaction was carried out under acidic or basic conditions. In a two-step procedure, first, hydrolysis of TEOS was carried out under acidic conditions, and then condensation of silanol groups was carried out under basic conditions.
The effects of synthesis conditions and solubility of drug on the microstructure of the matrix, the degradation of the matrix and the release of the drug are described in detail.
MATERIALS AND METHODS
Xerogel Preparation
One-Step Sol-Gel Process Using Acidic or Basic Catalyst
Silica gels were prepared by means of the hydrolysis and polycondensation of tetraethoxysilane (TEOS; Fluka) with different catalysts (30% hydrochloric acid; Fluka, pHsol = 4 or 25% ammonia solution; Fluka, pHsol = 9), in ethanol (96% EtOH; POCh Gliwice) solution, at room temperature. The alcoholic solution of drug was added into the silica solution after 1 hr hydrolysis. First the beaker content was mixed for 30 min, then was covered with a parafilm and left for gelation. The sol was allowed to gel for 6–9 days and was subsequently dried.
Two-Step Sol-Gel Process
Tetraethoxysilane (TEOS; Fluka), ethanol, drug, water and 30% hydrochloric acid were mixed and stirred to get uniform sols. The sols were hydrolyzed for 1 hr at room temperature before 25% ammonia solution was added. First the beaker content was mixed for 30 min, then was covered with a parafilm and left for gelation. The sol was allowed to gel for 0.5 hr and was subsequently dried. presents the types of the obtained xerogels and the sols composition.
TABLE 1 Composition of the precursors used in the experimentsFootnotea
Materials Characterization
All prepared xerogels were characterized using the BET technique based on nitrogen gas adsorption (ASAP 2405N, Micromeritics Instrument Corporation), FTIR spectroscopy (Jasco FT/IR – 410 spectrometer) and X-ray diffraction (XRD) (Philips X-Pert difractometer) analysis. The morphology of xerogels was observed using a Hitachi Scanning Electron Microscope (SEM) S-2500 (Tokyo, Japan).
Different conditions of synthesis resulted in changes in the porosity of the silica xerogels. Porosity of the samples was quantified by surface area and average pore size measurements. These studies were performed from silica xerogels not containing drugs.
Specific surface area and pore size of the xerogels were measured using the BET technique based on nitrogen gas adsorption. Before measurement, the samples were crushed and degassed at 200°C. The specific surface area was calculated from the BET equation. Average pore size was calculated by the BJH method based on the desorption branch of the isotherm.
The chemical composition of silica xerogels was determined with the FT/IR analysis. The spectra were measured over a range of 4000–400 cm− 1 with an instrument resolution of 4 cm− 1. Each individual spectrum was an average of 36 scans. In this measurement, the pulverized silica xerogels were mixed homogeneously with KBr powder and the background noise was corrected with pure KBr data.
XRD measurements were carried out on crushed samples of xerogels using a diffractometer with Cu Kα radiation at 40 kV and 30 mA, at room temperature.
The morphology of xerogels was observed using a scanning electron microscope. Prior to microscopy, the specimens were sputter coated with gold (Edwards, Model s150B Sputter Coater). Subsequently, the specimens were photographed and the microstructure of the silica xerogels was examined with a scanning electron microscope.
The time of gelation and textural properties of the gels are listed in .
TABLE 2 Gelation time, specific surface area, and average pore size of prepared xerogelsFootnotea
Study of In Vitro Release
The release of drugs were determined by ultraviolet spectroscopy, using a Hewlett Packard 8452A UV–VIS spectrophotometer.
Silica xerogel samples were soaked in 20 ml of simulated body fluid (SBF) as the dissolution medium (CitationKokubo et al. 1990). SBF was prepared by dissolving reagent grade NaCl (136.8 mM), NaHCO3 (4.2 mM), KCl (3.0 mM), K2HPO4· 3H2O (1.0 mM), MgCl2· 6H2O (1.5 mM), CaCl2· 2H2O (2.5 mM), Na2SO4 (0.5 mM) (from POCh Co., Poland, analytical grade purity) in redistilled water and the solution was buffered with tris(hydroxymethyl)aminomethane [TRIZMA] (from Sigma) and hydrochloric acid to pH 7.40.
The concentration of drug in SBF was measured by taking 10 ml of the solution at selected intervals based on the drug release. The tested solution was transferred back into the original vials for further release experiments.
The analysis was carried out by measuring the absorbance values at the maximum absorbance of Diclofenac at a wavelength λ = 276 nm and at the maximum absorbance of Ibuprofen at a wavelength λ = 273 nm. The experiment was finished when the difference between the subsequent values of the solution absorbance was smaller than 0.01 absorbance units. The measurements of each sample were repeated three times.
The following Peppas Power low equation (1) was used to test the release mechanism of drugs:
where the constant k depends upon the diffusion constant, the porosity and tortuosity of the matrix, the solubility of the drug in the solvent used, and the initial drug content within the matrix, and where Mt/M∞ is the released quantity of the drug and t represents the exposure time. The diffusional exponent (n) was calculated from the slope of the log(Mt/M∞) versus log time plots. A slope of 0.5 indicates a diffusional square root of time dependence and a slope of 1.0 indicates zero-order release kinetics (CitationBöttcher, Slowik, and Süβ 1998; CitationCosta and Sousa Lobo 2001).
Degradation of the Xerogels
Degradation of the matrices in SBF solution was evaluated by the spectrophotometric method based on the formation of silic-molybdenic blue complex (λmax = 814 nm) to determine the dissolved Si(OH)4 (MLukasiak and Czarnobaj 2002).
Silica xerogel samples were soaked in SBF solution (0.5 g/50 ml). The intervals were selected based on the silica xerogel matrix degradation rate. The solutions were analyzed daily. The SBF solution was replaced following each analysis.
The dissolved Si(OH)4 reacted with ammonium molybdate (5%) in pH = 1 to form silic-molybdenic acid. Then, 0.25% Mohr's salt (hydrated ammonium iron(II) sulphate) was added to the silic-molybdenic acid solution to obtain silic-molybdenic blue complex. Subsequently, the absorbance value at the maximum absorbance of silic-molybdenic blue complex was measured at wavelength λmax = 814 nm.
RESULTS AND DISCUSSION
Materials Characterization
After gelation the gels were dried by air and small glassy pieces were obtained. shows a monolith of an acid-catalyzed silica xerogel () that is crack-free and optically transparent, alongside monoliths of base-catalyzed silica xerogels and two-step prepared xerogels ( and , respectively) that are cracked and translucent. Low pH of sol stabilizes the resultant silica xerogels, increasing their mechanical strength. High pH of sol is the main reason for cracking of the solid sample. The bigger pores for silica xerogels prepared with a basic catalyst resulted in increased light scattering, and thus translucence.
FIG. 1 SiO2 xerogels made by the sol-gel method: (A) acid-catalyzed xerogel, (B) base-catalyzed xerogel, (c) two-step prepared xerogel.
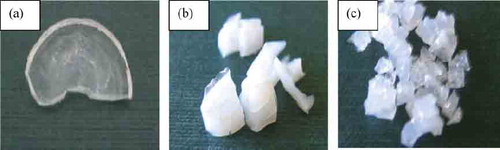
The X-ray diffraction pattern of the gels exhibits broad humps between 15° and 30° 2θ in both spectra, characteristic of the amorphous single-phase nature of the obtained materials ().
The effects of the acidic and basic catalysts on the surface area, and the pore size of matrices are shown in . The base-catalyzed xerogels exhibit the highest surface area and the largest average pore size. Use of the two-step method of synthesis of xerogels decreases both the surface area and the average pore size. For acid-catalyzed xerogels the porosity analysis was not applicable because the surface area and the pore size were too small to be measured with this technique.
shows the scanning electron images of the acid-catalyzed silica xerogel () and base-catalyzed silica xerogel () and two-step prepared silica xerogel (). The SEM showed that the xerogels had a rough surface. The acid-catalyzed silica xerogels showed a dense structure while the two-step prepared silica xerogel and especially the base-catalyzed silica xerogels showed a more globular morphology.
FT-IR spectra of the all samples of silica xerogels are similar (). All spectra are typical for well-polymerized silica and present the peaks at:
3500–3750 cm− 1, associated with H-bonded SiOH stretching vibrations and H-bonded water;
1650 cm− 1, which corresponds to vibrations of molecular water;
1075 cm− 1, 795 cm− 1, 454 cm− 1, which correspond to asymmetric and symmetric stretching vibrations of Si-O-Si and a bending Si-O-Si mode, respectively; and
952 cm− 1, which is related to the vibration of Si-OH bonding (MLasanczka et al. 1999).
FIG. 4 SEM micrographs for (A) acid-catalyzed xerogel, (B) base-catalyzed xerogel, (C) acid/base-catalyzed xerogel.
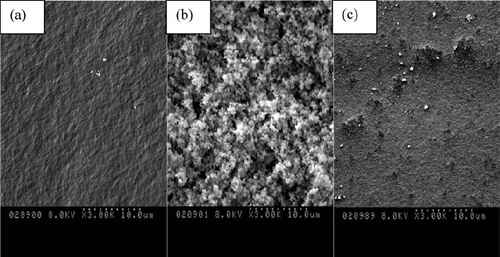
The absence of prominent C–H stretching bands over the 2800–3000 cm−1 region in the spectra suggests that the ethoxy groups were hydrolyzed during sol-gel synthesis.
Drugs Release from Silica Xerogels
For the xerogels obtained in the acid-catalyzed sol-gel route, Diclofenac was released irregularly. The release was 0.7% over 21 days (not shown). For Ibuprofen, in 21 days of test no peak characteristic of IB (at the wavelength λ = 273 nm) was found.
Test results of drug release from the matrix showed that gels obtained from HCl as a catalyst proved rather unsuitable as the carriers of the examined drugs. The most probable reason for this is too small diameter of the matrix pores and a strong occlusion of large-sized drug particles in such gels, which considerably hinder or practically disable water penetration and diffusion of an immobilized molecule from the silica net.
Drugs release profiles from base-catalyzed xerogels and from acid/base-catalyzed xerogels are reported in and .
FIG. 5 Release of Diclofenac and Ibuprofen from silica xerogels obtained in the basic catalysis conditions.
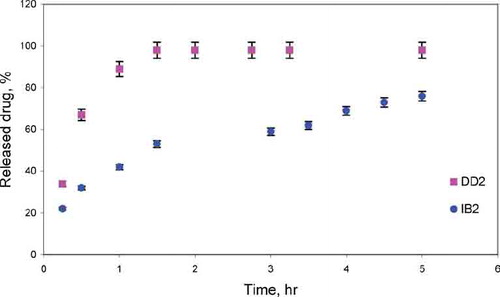
FIG. 6 Release of Diclofenac and Ibuprofen from silica xerogels obtained in the acid/base catalysis conditions.
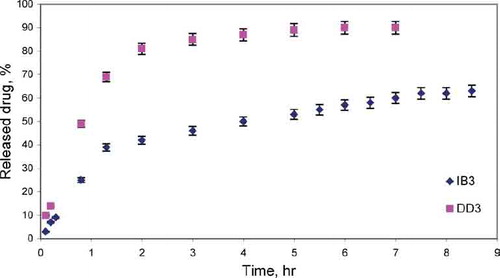
It was observed that the drugs were released in two stages. During the first hours drug (DD/IB) was released quickly, whereas after that time the experiment proceeded more slowly. The release manner also differed in the two stages distinguished: at first the release progressed mainly due to dissolution and diffusion of drug in the superficial layers of xerogels and, therefore, the process proceeded relatively quickly. Later on, drug release became slower. Within the inner part of xerogels, the three-dimensional network of silica effectively immobilized drug molecules, making it difficult for the medicine, due to steric reasons, to pass to the water phase.
Gels obtained in the base-catalyzed sol-gel route and in the two-step sol-gel route, due to the greater porosity than the gels catalyzed with HCl, proved useful matrices for drugs. In the case of xerogels which were not dried the release of the drug was effected almost completely during 1.5–8 hr.
Using the two-step sol-gel method for the synthesis of matrix clearly lowers the release of both Diclophenac and Ibuprofen compared with base-catalyzed one-step processed xerogels.
In all the cases studied, the released amount of DD was higher and the released time was shorter compared with IB from the same of matrices type. For DD the release percent was 90–98%, and release time was 1.5–5 h depending on the matrix type. For IB the release percent was 63–76%, and release time was 5–8h depending on the matrix type.
The release mechanism of drugs from xerogels matrices was estimated using the semi-empirical equation Mt/M∞ = ktn, which describes the release of drugs from various geometrical forms. The release of drug conformed to diffusion controlled release for DD (n = 0.58–0.64) and IB (n = 0.4–0.61) from air-dried xerogels ().
TABLE 3 Release kinetics parameters
The results show that the drugs release can easily be controlled by changing the catalyst. One may expect that the change in the drugs release comes mainly from the changes in the textual properties of the xerogels, and to a lesser extent from water-solubility of drug and from the interactions between DD or IB and the xerogel matrices.
The relatively large pore size of base-catalyzed silica gels may be responsible for the generally higher released amount of drugs from these gels. The lowest released amount of drugs from two-step processed silica gels is probably caused partly by their average pore size.
In the case of two-step processed silica gels, the interactions between the drug and the matrix, especially electrostatic interactions and hydrogen bonding, may play an important role in the release of DD and IB. The two-step prepared silica is reported to have more contribution of silanol groups than the one-step xerogels (CitationCao and Tian 1998; CitationKim et al. 2003). For these drugs-doped xerogels, the released amount is lower because of the hydrogen bonding and electrostatic attraction between DD or IB and many hydroxyl groups of matrix.
IB contains one carboxylic acid group, which can form the stronger bonding with hydroxyl groups via acid-base reaction. Electrostatic interactions may play an important role in the release of DD-ionized drug. These interactions reduce the released amount of drugs and prolong this process.
Study of the Degradation of the Silica Xerogel Matrix
Application of variable catalysts during xerogel synthesis affected the degradation behavior of the silica xerogel matrix in vitro ().
The tests of matrix degradation showed that the degradation proceeds with greater speed in the gels obtained in the conditions of the basic catalysis and two-step method. In comparison with acid-catalyzed xerogels for which the degradation amounted to 0.4% per month, the degradation of base-catalyzed xerogels and acid/base-catalyzed xerogels was over twice as high (0.6–0.7% per month). It might result from the fact that they are characterized by greater porosity and a larger specific surface area, compared to the silica xerogels prepared in an acidic pH. These two factors may also account for the subsequent greater dissolution of the silica xerogel matrix.
The study of matrix degradation indicates that the low degradation of xerogels (0.4–0.7% per month) also has little effect on the drug release.
CONCLUSIONS
The drug-loaded xerogels were prepared by a different type of sol-gel method and by using a different type of catalyst to investigate the slow release of two representative drugs, viz., DD and IB.
From the results obtained in this study it may be stated that the sol-gel method is a useful tool for trapping medicines such as Diclofenac or Ibuprofen in the pores of silica xerogels. The presented method is repeatable.
The percent of cumulative release of drugs from silica xerogels was strongly catalyst-dependent. From three types of gels tested, base-catalyzed silica xerogels were much more effective than two-step prepared silica xerogels and acid-catalyzed silica xerogels.
In vitro release studies of drugs revealed a similar release profile in two steps: an initial diffusion-controlled release followed by a slower release rate. In all the cases studied, the released amount of DD was higher and the released time was shorter compared with IB from the same matrices type. This may result from water-solubility of DD and from stronger interactions between IB and the xerogel matrices.
Declaration of interest: The author reports no conflicts of interest. The author alone is responsible for the content and writing of the paper.
REFERENCES
- M. Ahola, P. Kortesuo, I. Kangasniemi, J. Kiesvaara, and A. Yli-Urpo. (2000). Silica xerogel carrier material for controlled release of toremifene citrate. Int. J. Pharm 195:219–227.
- M. S. Ahola, E. Sailynoja, M. Raitavuo, and et al (2001). In vitro release of heparin from silica xerogels. Biomaterials 22:2163–2170.
- D. J. Blyth, S. J. Poynter, and D. A. Russel. (1996). Calcium biosensing with a sol-gel immobilized photoprotein. Analyst 121:1975–1978.
- H. Böttcher, P. Slowik, and W. Süβ. (1998). Sol-gel carrier systems for controlled drug delivery. J. Sol-Gel Sci. Tech. 13:277–285.
- A. M. Buckley, and M. Greenblatt. (1994). The sol-gel preparation of silica gels. J. Chem. Educ. 71:599–602.
- G. Cao, and H. Tian. (1998). Synthesis of highly porous organic/inorganic hybrids by ambient presure sol-gel processing. J. Sol-Gel Sci. Tech. 13:305–309.
- P. Costa, and J. M. Sousa Lobo. (2001). Modeling and comparison of dissolution profiles. Eur. J. Pharm. Sci 13:123–133.
- K. Czarnobaj, and J. Łukasiak. (2004). In vitro release of cisplatin from sol-gel processed porous silica xerogels. Drug Del. 11:341–344.
- K. Czarnobaj, and J. Łukasiak. (2007). In vitro release of cisplatin from sol-gel processed organically modified silica xerogels. J. Mater. Sci. Mater. Med. 18:2041–2044.
- K. Czarnobaj, M. Prokopowicz, and J. Łukasiak. (2006). The effect of PEG concentration on the release rate of cisplatin from PEG-modified silica xerogels. Drug Del. 13:339–344.
- B. C. Dave, B. Dunn, J. Selverstone, D. Valentine, and J. I. Zink. (1994). Sol-gel encapsulation methods for biosensors. Anal. Chem. 66:1120A–1127A.
- F. de Gaetano, L. Ambrosio, M. G. Raucci, A. Marotta, and M. Catauro. (2005). Sol-gel processing of drug delivery materials and release kinetics. J. Mater. Sci Mater. Med 16:261–265.
- J. Fang, K. C. Sung, H. Lin, and C. Fang. (1999). Transdermal iontophoretic delivery of diclofenac sodium from various polymer formulations: in vitro and in vivo studies. Int. J. Pharm. 178:83–92.
- T. Heikkil, J. Salonen, J. Tuura, M. S. Hamdy, G. Mul, N. Kumar, T. Salmi, D. Yu. Murzin, L. Laitinen, A. M. Kauhonen, J. Hirvonen, and V.-P. Lehto. (2007). Mesoporous silica material TUD-1 as a drug delivery system. Int. J. Pharm 331:133–138.
- S. M. Kim, K. Chakrabarti, E. O. Oh, and C. M. Whang. (2003). Effect of the base catalyzed reaction of two/step acid/base catalyzed process on the microstructures and physical properties of poly(dimethylsiloxane)modified silica xerogels. J. Sol-Gel Sci. Tech. 27:149–155.
- L. C. Klein. (1993). Sol-gel optical materials. Annu. Rev. Mater. Sci. 23:437–452.
- T. Kokubo, H. Kushitani, S. Sakka, T. Kitsugi, and T. Yamamuro. (1990). Solutions able to reproduce in vivo surface-structure changes in bioactive glass-ceramic A-W. J. Biomed. Mater. Res. 24:721–731.
- P. Kortesuo, M. Ahola, S. Karlsson, I. Kangasniemi, A. Yli-Urpo, J. Kiervaara, and et al (2000). Silica xerogel as an implantable carrier for controlled drug delivery—evaluation of drug distribution and tissue effects after implantation. Biomaterials 21:193–198.
- A. Kramar, S. Turk, and F. Vreˇer. (2003). Statistical optimisation of diclofenac sustained release pellets coated with polymathacrylic films. Int. J. Pharm. 256:43–52.
- M. Łączka, K. Cholewa-Kowalska, K. Kulgawczyk, M. Klisch, and W. Mozgawa. (1999). Structural examinations of gel-derived materials of the CaO-P2O5-SiO2 system. J. Mol. Struct. 511–512:223–231.
- C.-J. Li, Y.-H. Lin, C.-L. Shih, J.-P. Tsaur, and L.-K. Chan. (2002). Sol-gel encapsulation of lactate dehydrogenase for optical sensing of L-lactate. Biosensors Bioelec. 17:323–330.
- J. Livage, and C. Sanchez. (1994). Sol-gel chemistry. J. Non-Cryst. Solids 45:11–19.
- J. Łukasiak, and K. Czarnobaj. (2002). Evaluation of the usefulness of the spectrophotometric method based on the reaction of formation of silic-molybdenic blue for determination of silica in hydrogenated fat. Roczn. PZH 53:259–265.
- D. T. Nguyen, M. Smit, B. Dunn, and J. I. Zink. (2002). Stabilization of creatine kinase encapsulated in silicate sol-gel materials and unusual temperature effects on its activity. Chem. Mater. 14:4300–4306.
- S. Radin, S. Falaize, M. H. Lee, and P. Ducheyne. (2002). In vitro bioactivity and degradation behavior of silica xerogels intended as controlled release materials. Biomaterials 23:3113–3122.
- J. C. Ro, and I. J. Chung. (1991). Structures and properties of silica gels prepared by sol-gel method. J. Non-Cryst. Solids 130:8–17.
- H. Schmidt. (1988). Chemistry of material preparation by the sol-gel process. J. Non-Cryst. Solids 100:51–64.
- L. Sieminska, and T. W. Zerda. (1996). Diffusion of steroids from sol-gel glass. J. Phys. Chem. 100:4591–4597.
- S. Wu, J. Lin, and S. I. Chan. (1994). Oxidation of dibenzothiophene catalyzed by heme-containing enzymes encapsulated in sol-gel glass. A new form of biocatalysts. Appl. Biochem. Biotechnol 47:11–17.
- J.-Z. Yi, and L.-M. Zhang. (2007). Biodegradable blend films based on two polysaccharide derivatives and their use as ibuprofen-releasing matrices. J. Appl. Polym. Sci. 103:3553–3559.