Abstract
It has been demonstrated that spray-drying is a powerful method to prepare dry powders for pulmonary delivery. This paper prepared dispersible dry powders based on chitosan and mannitol containing honokiol nanoparticles as model drug. The results showed that the prepared microparticles are almost spherical and have appropriate aerodynamic properties for pulmonary delivery (aerodynamic diameters was between 2.8–3.3 μm and tapped density ranging from 0.14–0. 18 g/cm3). Moreover, surface morphology and aerodynamic properties of the powders were strongly affected by the content of mannitol. Fourier transform infra-red (FTIR) spectrum of powders indicated that the honokiol nanoparticles were successfully incorporated into microparticles. In vitro drug release profile was also observed. The content of mannitol in powders significantly influenced the release rate of honokiol from matrices.
Introduction
At present, many drugs in clinic have to be administered by injection because of its poor oral bioavailability. However, injection route is not convenient for the patients due to poor compliance, pain, and etc. In the search for needle-free delivery, the administration of therapeutic drugs by inhalation is a promising alternative that has gained considerable interest over the past few years (CitationSmith, 1997; CitationBosquillon et al., 2004). The lung was an attractive route for drug delivery because it was an efficient portal to the bloodstream, and it also could be the target organ. Besides, the lung could offer large absorptive surface area in the range of 35–140 m2, and thin (0.2 μm) and highly vascularized epithelium, which could lead to high bioavailability (CitationShoyele and Cawthorne, 2006). Moreover, inhalation is well accepted by the general population because of the convenience and good compliance (CitationByron, 1990).
Preparation of dry powder formulations for inhalation is an attractive and appreciated proposal because solubility and stability issues of drugs can be resolved. Furthermore, a dry powder aerosol offers the capacity to provide a wide range of single dose per inhalation. The success of inhaled particles mostly depends on their size and density, and especially aerodynamic diameter (dae) (CitationGrenha et al., 2005). Generally, the powder with aerodynamic diameter ranging from 1–5 μm could guarantee a maximum deposition in the deep lung. Particles size smaller than 1 μm failed to deposit in lung because it was easily breathed out again, and particle sizes larger than 5 μm also failed to deposit in the lung because they could not pass through the upper airways and cannot reach the deep lung (CitationBroadhead et al.,1992; CitationBosquillon et al., 2001).
Micronization is usually adopted to reduce the particle size of powder to less than 5 μm. Traditional method of micronization was using a ball or jet mill to produce powder. Whereas milling is a destructive technique with disadvantages such as lack of control over parameters such as morphology, size and surface properties of milled particles associated with high energy input can promote chemical degradation of drugs (CitationShoyele and Cawthorne, 2006; CitationJohnson, 1997; CitationIrngartinger et al., 2004). Recently, spray-drying as a successful process that provided great control over particle size, particle morphology, and powder density, has been explored to prepare protein/chemical drug-contained powder in a simple step from solutions. CitationNiven et al. (1994) has successfully prepared micronized powders containing recombinant human granulocyte colony-stimulating factor (rhG-CSF) with bioactivity detained. And the powders were tested for pulmonary absorption. The insulin powders formulated with mannitol, glycine, and sodium citrate as excipients were tested in clinical trials (CitationBosquillon et al., 2004; CitationSkyler et al., 2001). Spray-drying is normally performed by the atomization of a suspension, which is sprayed into a hot drying medium (normally air). The formulation of powders including the drug as well as the excipients could affect the physicochemical stability and/or the aerodynamic properties of dry powder, thus changing the depositive site (upper airway, bronchi, alveoli, etc.) of the powder in vivo. Formulation strategies to prolong the drug’s retention time in the lung can be useful to improve or retard absorption, minimizing the biodistribution throughout the systemic circulation, thus influencing the therapeutic effects and reducing side effects (CitationVentura et al., 2008). Chitosan and mannitol as excipients were approved by the Food and Drug Administration (FDA) for aerosol. Chitosan (CS), as a semi-crystalline aminopolysaccharide prepared by deacetylation of chitin, has been widely used in the biomedical field owing to its favorable properties such as non-toxicity, good biocompatibility, biodegradability, etc. (CitationBosquillon et al., 2001; CitationAlpar et al., 2005; CitationYamamoto et al., 2005). Moreover, by means of a reduction of mucociliary clearance and the opening of tight intercellular junctions, chitosan is able to increase drug bioavailability after nasal administration (CitationVasir et al., 2003).
Nanoparticles have been proposed as valuable vehicles for efficient drug transport to the lung epithelium while avoiding the mucociliary clearance and phagocytic mechanisms. However, nanoparticles without using any excipients for pulmonary delivery had intrinsic disadvantages such as easy aggregation during storage in the form of powder, and could be easily breathed out again due to their small size below 1 μm (Griese and Reinhardt, 1998; Dickinson et al., 2001; Clark, 2002; Makino et al., 2003). In this paper, we report the preparation and characterization of dry powders containing honokiol nanoparticles and used chitosan and mannitol as excipients. Here, we selected honokiol as model hydrophobic drug to prepare honokiol nanoparticles enhancing the solubility and stability of honokiol. Meanwhile, we demonstrated that selecting chitosan and mannitol as excipients could modify the aerodynamic property of powders and tailor the rate of drug release, hence could offer the opportunity for pulmonary delivery of honokiol nanoparticles.
Materials and methods
Materials
Chitosan (with 92% degree of deacetylation (DD) and a viscosity of 55 mpa.s (1% in 1% acetic acid, 20°C)) and Poloxamer F127 were supplied by Sigma (USA). Mannitol solution (20%, w/v) was purchased from Sichuan KeLun Pharmaceutical Co. Ltd (Chengdu, China). Ethyl acetate (Et Ac) was obtained from KeLong Chemicals (Chengdu, China). Honokiol was purified in our library by HSCCC (CitationChen et al., 2007). All other chemicals used in this work were of analytical reagent grade. Deionized distilled water from Milli-Q water system was used to prepare the aqueous solutions.
Preparation of microparticles
Honokiol nanoparticles loaded chitosan/mannitol microparticles were prepared by the spray-drying method. Honokiol nanoparticles were prepared by emulsion solvent evaporation method developed in our lab (CitationGou et al., 2008). Briefly, chitosan was dissolved in 1% acetic acid solution at a concentration of 10 mg/ml. Then 10 ml of honokiol nanoparticles suspension (15 mg/ml) and different amounts of 20% mannitol solution (10, 20, 30, and 40 ml) were added to 100 ml of chitosan/acetic acid solution, respectively. Spray-drying of these solutions was performed in a laboratory-scale spray-dryer (Büchi® Mini Spray dryer, B-290, Switzerland). The conditions of the spray-drying process were: feed rate 3.2 mg/ml, nozzle diameter 0. 7 mm, aspirator pressure 90% and inlet temperature 140°C. These condition resulted in an outlet temperature of 65–75°C. The spray-dried powders were collected and stored at ambient temperature for further analysis.
Physical and chemical characterization of the spray-dried powders
Spray-drying yield and drug content
The yields of spray-dried powders were calculated by gravimetry. Honokiol content of the prepared powders was determined by high-performance liquid chromatography (HPLC), and the result was the mean of three test runs.
Morphological analysis
The morphological characterization of spray powders was performed by scanning electron microscopy (JSM-5900LV, JEOL, Japan). Powder samples were placed at cabinet drier for 24 hr before observation. Then samples were sputtered with gold before examination.
The moisture of prepared powders
The moisture of obtained powders was determined by the oven drying method (CitationRattes and Oliveira, 2007). Powder samples with anticipated weight were placed in a drying and sterilization oven (DZF-6050, Shanghai YiHeng Technology Co. Ltd, China) at 105°C, and weighed by an electronic balance (AUW-120D, Shimadzu, Japan) until the constant weight. The moisture of powders was detected from the weight loss on drying. Measurements were performed in triplicate.
Particle size, powder density, and primary aerodynamic diameter
The particle size of prepared powders was determined by laser diffraction (BT-2002 Laser Particle Size Analyzer, Dandong Bettersize Instruments Ltd, China). Approximately 2 mg of prepared powders were suspended in a mixture of cyclohexane and Tween20 under ultrasound in order to disperse the particles before detection. Each sample was measured in triplicate. The data obtained were expressed as the volume weighted mean particle size.
The bulk density of powders was detected using 0.5g of powder and a 10 ml-graduated cylinder. The tapped density of powders was determined by tapped density measurements on the same amount samples in a 10 ml-test tube after mechanical tapping until constant volume was obtained, according to a previously described method (CitationGrenha et al., 2005). Measurements were performed in triplicate. Carr’s Index values of each spray-dried powder were derived from bulk density and tapped density data, as presented in equation (CitationSmith, 1997). Carr’s Index could be adopted to evaluate the flow properties of powders. A value less than 25% indicates a fluid powder, whereas a value greater than 25% indicates a cohesive powder (CitationDe Villiers, 2005; CitationLearoyd et al., 2008).
Aerodynamic diameter (dae) of the particle is a key parameter determining the aerosol performance of an inhaled powder (CitationChan, 2006). For solid spherical particles, dae is simply equal to the physical diameter (d) multiplied by the square root of the particle density (ρ)
This means that, for a given density, small particles have lower aerodynamic diameter contrasted to larger particles.
Fourier transform infra-red (FTIR) measurements
FTIR (KBr) spectra were taken at room temperature using a NICOLET 200SXV Infrared Spectrophotometer (USA). The characteristic absorption bands of the drug and polymers were detected in samples including honokiol nanoparticles, chitosan, mannitol, and their physical mixtures and the prepared powders.
In vitro delivery behavior of honokiol-loaded powders
In vitro delivery behavior of honokiol from drug-loaded powder was carried out in phosphate buffer solution (PBS, pH7.4) at 37°C. One hundred milligrams of powders were added into 200 ml of PBS in stopper bottles at 100 rpm/min. Aliquots of 2 ml were withdrawn at specific time intervals and centrifuged at 13,000 rpm for 5 min, and the medium in the bottles was replaced with 2 ml of fresh PBS (pre-warmed to 37°C). The supernatant was stored at −20°C before further analysis by HPLC.
High performance liquid chromatography (HPLC) analysis
HPLC (Waters Alliance 2695-2996, USA) was used to detect the concentration of honokiol. All the samples were diluted with distilled water before detection. HPLC analysis was performed on a reversed phase C18 column (4.6 × 150 mm, 5 μm, Sunfire Analysis column) at room temperature. The mobile phase was Acetonitrile/water (60/40, v/v), filtered through a 0.22 μm Millipore filter and degassed prior to use. The flow-rate was 1.0 ml/min and the effluent was detected by Waters PDA detector at 210 nm.
Results and discussion
Microspheres preparation and morphological characterization
In this paper, dry powders were prepared by spray-drying technology using chitosan and mannitol as excipients. Both these excipients were approved by the Food and Drug Administration (FDA) for pulmonary delivery (CitationBosquillon et al., 2001). We investigated the effect of chitosan/mannitol mass ratio on aerodynamic diameter and morphologic properties of the powders. As shown in , moisture contents of powders ranged from 3.8–5.2%. It was noticed that different formulations had similar moisture content. Due to residual moisture in powders, some of these powders adhere to the drying chamber wall surface, piping, and/or cyclone, resulting in low production yields ranging from 30–48% (). Relative low production yield occur regularly in the spray-drying process and can be caused by properties of excipients and the temperature or humidity conditions in the drying chamber. Also, we should emphasize that the lab-scale drier typically had a lower product yield compared with the industrial-scale spray-dryers because of higher wall deposits, since air residence times and radial distances from the atomizer to the drying chamber wall are shorter (Simonoska et al., 2008). The morphological characterization of prepared powders was characterized on scanning electron microscopy (SEM). Four typical scanning electron micrographs of obtained powders are presented in . According to SEM analysis, the morphology of the prepared powders was strongly dependent on powder composition. As presented in , the honokiol nanoparticles loaded chitosan/mannitol (w/w, 1:2) microparticles are not well spherical and appeared to be aggregated and fused. Spherical well-separated microparticles of uniform size were observed with the formulations of honokiol nanoparticles loaded chitosan/mannitol (w/w, 1:4) microparticles in and honokiol nanoparticles loaded chitosan/mannitol (w/w, 1:6) microparticles in . In contrast, microparticles (data not shown) obtained from chitosan/acetic acid solutions containing honokiol nanoparticles were well spherical with rough surface, but a little aggregated, and the production yield was relatively low. According to , microparticles obtained from honokiol nanoparticles loaded chitosan/mannitol (w/w, 1:8) microparticles appeared to have undergone part fusion, but also had well spherical particles, probably due to the high content of mannitol in the formulation.
Table 1. Process yield and water content of prepared powders with different formulations.
Figure 1. Typical scanning electron micrographs (SEM) of spray-dried powders with different formulations. (A) Honokiol nanoparticles loaded chitosan/mannitol (w/w, 1:2) microparticles, (B) Honokiol nanoparticles loaded chitosan/mannitol (w/w, 1:4) microparticles, (C) Honokiol nanoparticles loaded chitosan/ mannitol (w/w, 1:6) microparticles, (D) Honokiol nanoparticles loaded chitosan/mannitol (w/w, 1:8) microparticles.
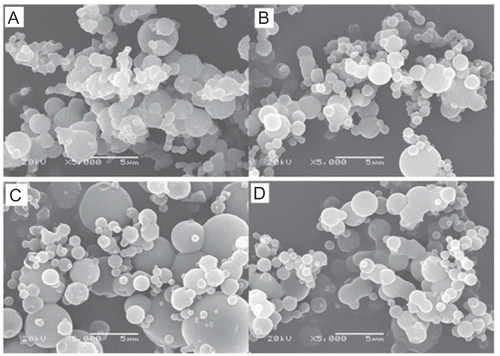
Particle size, density, and aerodynamic properties
The particle size of powder, together with the particle density, strongly influenced the dispersion and aerodynamic properties of the powders. As previously proved, the optimal aerodynamic diameter of the powders should be of approximately 1–5 μm (CitationBroadhead et al.,1992; CitationBosquillon et al., 2001). As presented in , the mean particle size of prepared powders ranged from 6.9–8.4 μm, and the aerodynamic diameter of powders was between 2.8–3.3 μm, suggesting that the powder with all these formulations were of a suitable aerodynamic size for pulmonary delivery. The tapped density of the spray-dried powders was similar for all powder ranging from 0.14– 0. 18 g/cm3. Carr’s Index was adopted as an indication of the powder flow properties: a value greater than 25% indicates cohesive powder characteristics, and a value less than 25% indicates a fluid flowing powder. As depicted in , the Carr’s Index of these prepared powders was between 13.5–34%. Powders from chitosan/mannitol (w/w, 1:2) and chitosan/mannitol (w/w, 1:8) had poor flowability, whereas powders from formulation of chitosan/mannitol (w/w, 1:4) and chitosan/mannitol (w/w, 1:6) indicated good flowability. Concerning aerodynamic properties and morphological characterization, we consider that the powders from formulation of chitosan/mannitol (w/w, 1:4) with an aerodynamic diameter of ca. 2.8 μm are the most suitable to carry the honokiol nanoparticles to the lung.
Table 2. Physical characterization of obtained powders with different formulations.
Polymer–drug interaction analysis using FTIR spectra
According to the characteristic spectra of polymers and honokiol nanoparticles separately, in physical mixtures and in the microparticles, we attempted to determine the existence and type of interactions between the polymers and the drug. Chitosan spectra has been described by Mi et al. (2002) and they correspond to: –CO ( 1660 cm−1), C–O–C ( 1154 cm−1), and –NH2 ( 1600 cm−1), while the band at 3430 cm−1 belongs to the stretching vibrations of the hydroxyl groups via hydrogen bonds (). The characteristic IR bands of the mannitol consisted of 3310 cm−1 belonging to the νOH, 1420 cm−1 belonging to νCH, and 1280 cm−1 belonging to νC–O (). The IR spectra of honokiol nanoparticles including: –OH ( 3330 cm−1), C–O ( 1260 cm−1), and phene ring (1430, 1490, 1600, and 1630 cm−1) (). In the IR spectra of physical mixtures of chitosan, mannitol, and honokiol nanoparticles (), the characteristic honokiol nanoparticles peaks can be observed, indicating an absence of interactions between the drug and the polymers. However, in the IR spectra of the honokiol nanoparticles loading chitosan/mannitol microparticles (), the characteristic IR bands of the honokiol nanoparticles (1400– 1600 cm−1 owing to phenol ring) disappeared, which implied that there was an existence of interactions (ionic, hydrogen, and/or van der Waals’ forces) between honokiol and polymers (chitosan and mannitol) occurring during the procedure, resulting in the honokiol nanoparticles embedding into the particles.
In vitro drug release behavior
depicts the release profiles of the honokiol from the formulations of chitosan/mannitol (w/w, 1:2), chitosan/mannitol (w/w, 1:4), chitosan/mannitol (w/w, 1:6), and chitosan/mannitol (w/w, 1:8) in PBS (pH 7.4) at 37°C. During dissolution of prepared powders, the formation of a thick gel was observed, which expanded slowly over time. This phenomenon we observed might be caused by the chitosan which gelled in the PBS medium. Mannitol as a hydrophilic excipient was mixed with the chitosan matrix, causing a faster drug release because mannitol acted as a channeling agent in the formulation. As we predicted, the release rate of honokiol increased with an increase of the content of the mannitol in the formulation (release rate of honokiol:chitosan/mannitol (w/w, 1:8) > chitosan/mannitol (w/w, 1:6) > chitosan/mannitol (w/w, 1:4) > chitosan/mannitol (w/w, 1:2)). Ninety-six hours later, approximately 90% honokiol was released from the preparation of chitosan/mannitol (w/w, 1:8) in PBS (pH 7.4) at 37°C, yet only about 50% honokiol was released from the other formulations. Therefore, according to this study we could conclude that the content of mannitol in the formulations significantly affected the release rate of honokiol. Therefore, we can tailor the release rate of the honokiol by regulating the mannitol in the formulation as we anticipated.
Figure 3. In vitro release profile of the spray-dried powders with different formulations in PBS (pH7.4) at 37°C. (▪) Formulation of powders with chitosan/mannitol (1:8); (•) Formulation of powders with chitosan/mannitol (1:6); (▴) Formulation of powders with chitosan/mannitol (1:4); (▾) Formulation of powders with chitosan/mannitol (1:2).
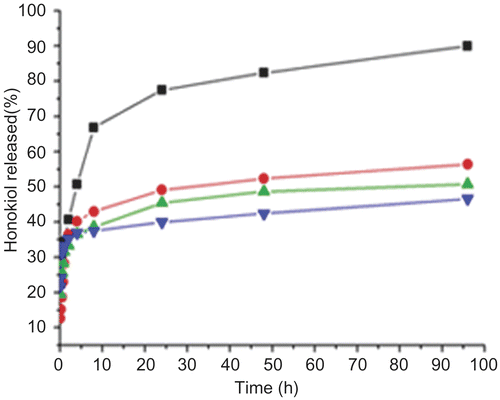
Conclusion
In this article, we demonstrated that honokiol nanoparticles can be successfully incorporated into microspheres by the spray-drying technique, resulting in respirable powders adequate for pulmonary delivery. The content of mannitol in formulation significantly affected the morphology and aerodynamic properties of the powders. In vitro release behavior of the powders indicated that the release rate of honokiol can be modified by modulating the content of mannitol in the formulation. Therefore, this system can be proposed for systemic delivery and local delivery of therapeutic drug by modulating the properties of powders.
Acknowledgements
Here we would like to show our great thanks to Jing Zheng (Library of Chengdu University of Information Technology) for her great help in proof-reading and correcting the manuscript.
This work was financially supported by National 863 project (2007AA021902), National Natural Science Foundation of China (NSFC20704027), Sichuan Key Project of Science and Technology (2007SGY019), Sichuan Prominent Young Talents Program (07ZQ026-033), and Chinese Key Basic Research Program (2004CB518807).
Declaration of interest: The authors report no conflicts of interest. The authors alone are responsible for the content and writing of the paper.
References
- Smith PL. (1997). Peptide delivery via the pulmonary route: a valid approach for local and systemic delivery. J Contr Rel, 46, 99–106.
- Bosquillon C, Préat V, Vanbever R. (2004). Pulmonary delivery of growth hormone using dry powders and visualization of its local fate in rats. J Contr Rel, 96, 233–44.
- Shoyele SA, Cawthorne S. (2006). Particle engineering techniques for inhaled biopharmaceuticals. Adv Drug Deliv Rev, 58, 1009–29.
- Byron PR. (1990). Determinants of drug and polypeptide bioavailability from aerosols delivered to the lung. Adv Drug Deliv Rev, 5, 107–32.
- Grenha A, Seijo B, Remuñán-López C. (2005). Microencapsulated chitosan nanoparticles for lung protein delivery. Eur J Pharm Sci, 25, 427–37.
- Broadhead J, Edmond-Rouan SK, Rhodes CT. (1992). The spray drying of pharmaceuticals. Drug Dev Ind Pharm, 18, 1169–206.
- Bosquillon C, Lombry C, Préat V, Vanbever R. (2001). Influence of formulation excipients and physical characteristics of inhalation dry powders on their aerosolization performance. J Contr Rel, 70, 329–39.
- Johnson KA. (1997). Preparation of peptide and protein powders for inhalation. Adv Drug Deliv Rev, 26, 3–15.
- Irngartinger M, Camuglia V, Damm M, Goedea J, Frijlink HW. (2004). Pulmonary delivery of therapeutic peptides via dry powder inhalation: effects of micronisation and manufacturing. Eur J Pharm Biopharm, 58, 7–14.
- Niven RW, Lott FD, Ip AY, Cribbs JM. (1994). Pulmonary delivery of powders and solutions containing recombinant human granulocyte colony-stimulating factor. Pharm Res, 11, 1101–9.
- Skyler, SJ, Cefalu WT, Kourides IA, Landschulz WH, Balagtas CC, Cheng SL, Gelfand RA. (2001). Efficacy of inhaled human insulin in type 1 diabetes mellitus: a randomized proof-concept study. Lancet, 357, 331–5.
- Ventura CA, Tommasini S, Crupi E, Giannone I, Cardilec V, Musumecib T, Puglisi G. (2008). Chitosan microspheres for intrapulmonary administration of moxifloxacin-Interaction with biomembrane models and in vitro permeation studies. Eur J Pharm Biopharm, 68, 235–44.
- Alpar HO, Somavarapu S, Atuah KN, Bramwell VW. (2005). Biodegradable mucoadhesive particulates for nasal and pulmonary antigen and DNA delivery. Adv Drug Deliv Rev, 57, 411–30.
- Yamamoto H, Kuno Y, Sugimoto S, Takeuchi H, Kawashima Y. (2005). Surface-modified PLGA nanosphere with chitosan improved pulmonary delivery of calcitonin by mucoadhesion and opening of the intercellular tight junctions. J Contr Rel, 102, 373–81.
- Vasir JK, Tambwekar K, Garg S. (2003). Bioadhesive microspheres as a controlled drug delivery system. Int J Pharm, 255, 13–32.
- Griese M, Reinhardt D. (1998). Smaller sized particles are preferentially taken up by alveolar type II pneumocytes. J Drug Target, 5, 471–9.
- Dickinson PA, Howells SW, Kellaway IW. (2001). Novel nanoparticles for pulmonary drug administration. J Drug Target, 9, 295–302.
- Clark A. (2002). Formulation of proteins and peptides for inhalation. Drug Deliv Syst Sci, 2, 73–7.
- Makino K, Yamamoto N, Higuchi K, Harada N, Ohshima H, Terada H. (2003). Phagocytic uptake of polystyrene microspheres by alveolar macrophages: effects of the size and surface properties of the microspheres. Colloids Surf B Biointerf, 27, 33–9.
- Chen L, Zhang Q, Yang G, Fan L, Tang J, Garrard I, Ignatova S, Fisher D, Sutherland IA. (2007). Rapid purification and scale-up of honokiol and magnolol using high-capacity high-speed counter-current chromatography. J Chromatography A, 1142, 115–22.
- Gou ML, Li XY, Dai M, Wang XH, Gong CY, Deng HX, Xie Y, Wang K, Zhao X, Qian ZY, Wei YQ. (2008). A novel injectable local hydrophobic drug delivery system: biodegradable nanoparticles in thermo-sensitive hydrogel. Int J Pharm, 359, 228–33.
- Rattes ALR, Oliveira WP. (2007). Spray drying conditions and encapsulating composition effects on formation and properties of sodium diclofenac microparticles. Powder Technol, 171, 7–14.
- De Villiers MM. (2005). Powder flow and compressibility. In: Ghosh TK, Jasti BR, eds. Theory and Practice of Contemporary Pharmaceutics. Boca Raton, FL: CRC Press, 298–9.
- Learoyd TP, Burrows JL, French E, Seville PC. (2008). Chitosan-based spray-dried respirable powders for sustained delivery of terbutaline sulfate. Eur J Pharm Biopharm, 68, 224–34.
- Chan HK. (2006). Dry powder aerosol drug delivery-opportunities for colloid and surface scientists. Colloids Surf A Physicochem Eng Aspects, 284–5, 50–5.