Abstract
To obtain novel biodegradable sirolimus-releasing polymer films that could release nanovehicles incorporating sirolimus, polyester, a water-soluble amphiphilic phospholipid polymer, and sirolimus were blended. The sirolimus-releasing polymer films were characterized by scanning electron microscopy and differential scanning calorimetry. Nanovehicles were formed with the phospholipid polymer chains and could be released from the sirolimus-releasing polymer films. The hydrodynamic diameter of the nanovehicles in phosphate-buffered saline was smaller than 20 nm. The nanovehicles substantially enhanced the carrier-mediated delivery of sirolimus and attenuated its degradation product. This study demonstrates that the delivery of sirolimus was enhanced by nanovehicles released from sirolimus-eluting materials.
Introduction
Stent thrombosis is a catastrophic complication of drug-eluting stents; it results in abrupt vessel closure and myocardial infarction (Citation1). During the enthusiastic era when little was known about stent thrombosis, drug-eluting stents were implanted into millions of patients every year, despite a lack of evidence-based medicine (Citation1,Citation2). Prompted by the reality that stent thrombosis occurred more than 1 year after implantation, some researchers, including ourselves, have endeavored to develop drug-eluting stents without a permanent metallic platform (Citation3,Citation4).
The development and delivery of cytostatic molecules is a pharmacological approach for targeting the atherosclerotic lesion (Citation5). The most promising agent for this is known to be sirolimus (SRL) (Citation5–7). SRL is a potent immunosuppressant, which inhibits the mammalian target of rapamycin (mTOR), a central regulator of protein synthesis and cell growth (Citation5,Citation6). Not surprisingly, some clinical studies have reported better outcomes with SRL-eluting stents than with paclitaxel-eluting stents (Citation7), although the comparative clinical studies are still in process. However, there are few published reports on in vitro studies of SRL release, in spite of the poor control of the SRL release from the current SRL-eluting stents (Citation8,Citation9). The discrepancy between the clinical need and lack of published in vitro data is probably due to the instability of SRL in buffer solutions. SRL is an unstable agent; its degradation produces an open-chain isomer, 34-hydroxy SRL (); it retains less than 10% of the antiproliferative activity of the parent SRL (Citation10,Citation11).
Figure 1. A degradation product of SRL reported by Ferron and Jusko (Citation10) and Trepanier et al. (Citation11).
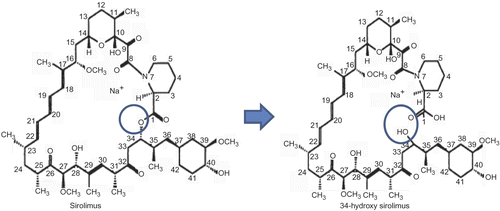
An attractive approach to stabilizing bioactive agents is to incorporate them into nanovehicles (Citation12). It was generally known that block copolymers could form reasonably sufficient nanovehicles before French and Canadian groups published several reports about the colloidal stability of the integral membrane proteins/amphiphilic random copolymers complex containing phosphorylcholine groups in buffer; the size dispersity was well controlled (Citation13,Citation14). Before this contribution to the field of polymer therapeutics, we have reported a biocompatible and water-soluble amphiphilic polymer, poly (2-methacryloyloxyethyl phosphorylcholine (MPC)-random-n-butyl methacrylate (BMA) (PMB30W) (). The PMB30W chains assembled themselves in an aqueous medium and formed colloidal aggregates. These aggregates were used as nanovehicles to maintain the hydrophobic drugs inside of the nanovehicles in a stable manner (Citation15–17). We hereby propose a new drug release system, PMB30W-functional biodegradable polymer films. These films can release nanovehicles with hydrophobic drugs in a solubilized form. Therefore, the solubility of the drugs in aqueous medium is enhanced and an efficient delivery can be expected. Polymer films composed of poly(L-lactide-random-caprolactone-random-glycolide) (PLCG), PMB30W, and SRL were produced by a simple solution blending technique in order to prepare the drug-releasing layer as a local temporary delivery system.
Materials and methods
Materials
PLCG (monomer unit composition, L-lactide/caprola- ctone/glycolide = 70/20/10; weight-averaged molecular weight (Mw) = 50 kDa) was purchased from Sigma-Aldrich (USA). PMB30W (Mw = 50 kDa) was synthesized as described in Ishihara et al. (Citation15). SRL (Mw = 914 Da) was purchased from Toronto Research Chemicals (Canada). All other chemicals and reagents used were of analytical grade.
Preparation of polymer films
A 24 wt% PLCG/PMB30W/SRL (weight ratio, 90/10/1) blend polymer solution in acetone/ethanol (Film A; volume ratio, 70/30) or in acetone/methanol (Film B; volume ratio, 70/30) was prepared by a simple solvent blending method. The polymer solutions were spread onto the glass substrate, and the solvents were evaporated at room temperature overnight to form films. The polymer films were vacuum-dried at room temperature for 3 days and kept in a refrigerator at −20°C before use. The entire process, including preparation and evaluation of the SRL-releasing polymer films, was conducted by blocking out the light.
Characterization of polymer films
To observe the change in the surface morphology of SRL-releasing polymer films, the films were immersed in phosphate-buffered saline (PBS) and incubated at 37°C. After several periods of incubation, the polymer films were sufficiently rinsed with distilled water and freeze-dried. The films were subjected to gold sputtering, after which their surface was observed under a scanning electron microscope (SEM; SM-200, Topcon, Japan).
Thermal analysis was carried out with a differential scanning calorimeter (DSC; DSC 6100, Seiko Instruments, Japan). After the polymer films were cooled at −50°C, the temperature was raised to 250°C at a rate of 10°C/min. The glass transition temperature (Tg) was obtained at the inflection point of the DSC curve.
Characterization of the colloidal aggregates
Two hundred milligrams of SRL-releasing polymer films were incubated in 2 mL of PBS at 37°C for 3 days. The PBS was subjected to dynamic light scattering (DLS) measurements (Zetasizer Nano-ZS; green badge, ZEN3500, Malvern, UK) with a 633-nm He-Ne ion laser. Both PMB30W aggregates with and without SRL (PMB30W/SRL = 10/1, weight ratio) were also measured by the same method.
Evaluation of the released drug
Twenty milligrams of PLCG/SRL (1% drug/polymer loading) films were placed in 10 mL glass vials. Two milliliters of PBS containing 0.2% bovine serum albumin (BSA) or 2 mL PBS containing 0.2% BSA and 0.02% PMB30W were placed on the surface of the PLCG/SRL films. BSA was added into PBS to facilitate the carrier-mediated delivery of SRL. The 1-octanol (Kanto Chemical, Japan) formed an upper immiscible phase on top of the PBS; most of drug released into the PBS would partition into the 1-octanol (Citation18). The SRL is stable in organic solvents (Citation19). The vials were capped and incubated via the above-mentioned method. The 1-octanol containing SRL and 34-hydroxy SRL was filtered with 0.20-μm pore membranes and injected onto a reversed-phase separation column (TSKgel ODS-80Ts, TOSOH, Japan). The mobile phase was 85% methanol and 15% water, flowed by a 0.5 mL/min pump, leading to a 15 min run time per sample. The detection was performed at 276 nm.
Twenty milligrams of SRL-releasing polymer films were placed in 10 mL glass vials. Following the addition of 2 mL PBS containing 0.2% BSA, the 1-octanol was added to the vials containing the SRL-releasing polymer films and PBS with BSA. The amount of SRL partitioned into the 1-octanol was determined by the above-mentioned method. The HPLC peak of 34-hydroxy SRL did not interfere with that of SRL, and the concentration of both compounds could be calculated from the HPLC results (Citation10).
Results and discussion
Using different solvents for dissolving and blending polymers with the same ratio resulted in different blend film characteristics. Film A showed a low Tg of 1.7°C, which is similar to that of PLCG (0.7°C), but that of Film B was 11.0°C. These data indicate that the PMB30W and SRL did not affect the film formation of PLCG alone when prepared in the ethanol/acetone mixture. PMB30W and SRL may be located near the surface of the polymer film. However, in the methanol/acetone mixture, PMB30W and SRL precipitated much more easily and embedded in the PLCG matrix, which is supported from the solubility of polymers viewpoint. Since ethanol (boiling point (bp), 78.4°C) evaporates more slowly than methanol (bp, 64.7°C) or acetone (bp, 56.5°C), ethanol-rich solvents remaining on the surface was more likely than methanol-rich solvents. The PLCG could be dissolved well in acetone, but not in methanol or ethanol. Conversely, PMB30W was soluble in these alcohols but not in acetone. The SRL could be dissolved in every solvent tested. According to the solubility of polymers in those solvents, an acetone-rich solvent could not dissolve PMB30W and SRL. Thus, the PMB30W and SRL formed domains inside the PLCG matrix.
illustrate the change in surface morphology of Film A after incubation in PBS. The air contact surface of Film A, which is the blood-contacting surface in vivo, demonstrated numerous pores after PBS incubation. PMB30W/SRL-rich materials were aggregated at the air contact surface and could be released into PBS in the case of Film A. In contrast, the air contact surface of Film B exhibited non-porous membranes after PBS incubation.
Figure 3. SEM images of the air contact surface during incubation in PBS. Film A: (A) before; (B) after 3 days. Film B: (C) before; (D) after 3 days.
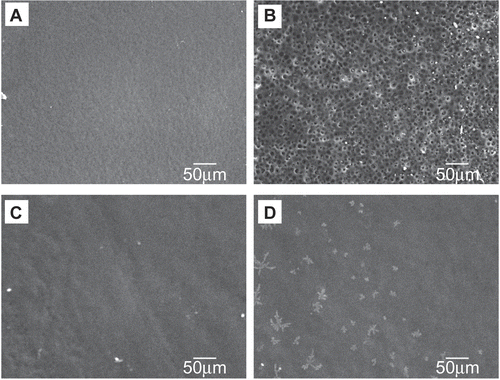
To exploit the potential of SRL delivery by PMB30W nanovehicles, SRL release profiles from PLCG/SRL films were studied in different mediums (). During PBS incubation with BSA and PMB30W, the SRL release substantially increased compared to that of the incubation in PBS with BSA only. PMB30W nanovehicles create the potential for drug carriers to have a strong affinity with SRL and reduce the amount of its degradation product.
Figure 4. Release profiles of (A) SRL and (B) 34-hydroxy SRL from PLGC/SRL films during incubation in PBS with BSA (diamonds) and that in PBS with BSA + PMB3OW (cross). Data points and error bars represent means ± SD for n = 4.
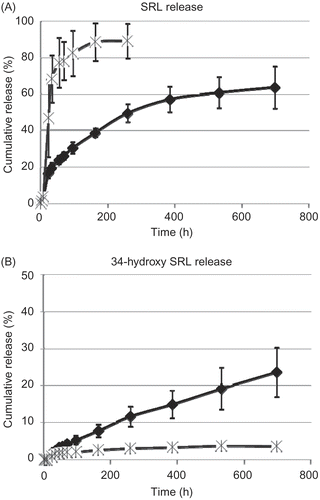
The hydrodynamic diameter of the PMB30W nanovehicles was evaluated using DLS (). PMB30W started to self-assemble and turn into nanovehicles with hydrophobic drugs, due to its amphiphilic characteristics and strong hydrophilicity from its phosphorylcholine groups after the PMB30W concentration reached 1 mg/mL in PBS. The PMB30W nanovehicles showed the steady hydrodynamic diameter since the PMB30W self-assembled regardless of its concentration in PBS. This result was consistent with the previous reports (Citation15,Citation20). The PMB30W nanovehicles solubilized SRL in PBS. The hydrodynamic diameter of PMB30W nanovehicles after incorporating SRL was slightly right-shift to that of PMB30W nanovehicles alone. The size of the nanovehicles released from the air contact surface of Film A was similar to that of the PMB30W nanovehicles incorporating SRL. The hydrodynamic diameter of all of nanovehicles was under 20 nm.
Figure 5. The size distribution of nanovehicles: PMB30W nanovehicles (dotted line); PMB30W nanovehicles incorporating SRL (broken line); the nanovehicles released from Film A (solid line).
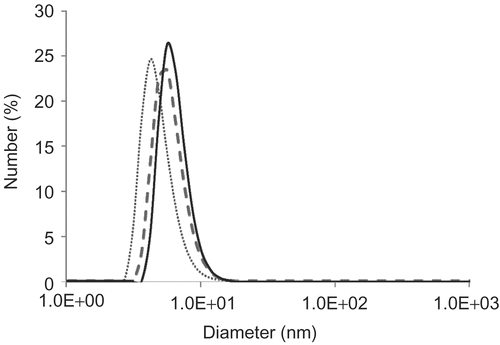
shows that the actual SRL release was enhanced by nanovehicles released from Film A. The release of nanovehicles from Film A resulted in more than 85% SRL release of the total SRL loading on polymer films into the 1-octanol phase. However, the SRL release (PLCG/SRL and Film B) in the BSA solution demonstrated less than 60% of the total SRL loading on polymer films into the 1-octanol phase. The PMB30W nanovehicles provided apolar circumstances, which dissolved SRL. In addition, the hydrophobic part (BMA units) of PMB30W nanovehicles could inhibit the entrance of water molecules, while the hydrophilic part (phosphorylcholine groups) of PMB30W is endowed with forming the small size of nanovehicles in an aqueous medium (Citation15,Citation20). Therefore, the shielding effect provided by PMB30W nanovehicles suppressed the hydradation of SRL and enhanced the carrier-mediated delivery of SRL.
Figure 6. Release profiles of (A) SRL and (B) 34-hydroxy SRL from PLCG/SRL films (black squares), Film A (white circles), and Film B (white triangles) during incubation in PBS with BSA. Data points and error bars represent mean ± SD for n = 4–5.
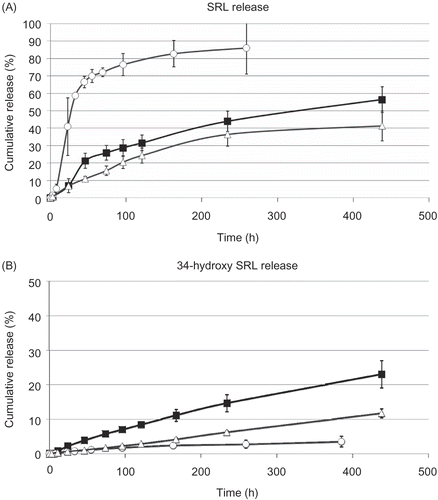
The internal elastic lamina of vascular tissues is supposed to be an impermeable barrier to water and solute, except fenestral pores (Citation21). In an in vivo situation, most of the SRL is taken up by red blood cells; few remnants (less than 0.1%) of SRL are incorporated by carriers such as albumin to be released into the target area (Citation11). It has also been discussed that drug disposition on the arterial endothelium may promote thrombus formation (Citation22), although the etiology of stent thrombosis is multifactorial and not fully understood. The SRL release incorporated by nanovehicles might deliver the SRL into smooth muscle cells through fenestral openings. Therefore, this novel method for enhancing the carrier-mediated delivery of SRL is a candidate for reducing the required amount of drug loading, and may attenuate the stent thrombosis. Studies are underway to evaluate blood clot formation and hyperplasia after implantation of these biodegradable SRL-releasing films into anastomotic sites of the abdominal aorta in rats.
Conclusion
This study demonstrates that the SRL release was facilitated by nanovehicles from a SRL-releasing biodegradable polymer blend. The nanovehicles could suppress the unfavorable reaction of SRL and enhance the release of hydrophobic SRL due to their shielding effects. Choosing the solvent for preparation of the SRL-releasing polymer blend could regulate the release pattern. We concluded that the SRL-releasing polymer blend for releasing nanovehicles that incorporate SRL is one of the candidates for SRL delivery methods in order to develop a biodegradable cardiovascular stent with drug releasing capabilities.
Acknowledgements
The authors thank Mr. J.-H. Seo and Mr. Y. Goto, The University of Tokyo for their technical supports and guidance. One of the authors (H.-I. Kim) thanks for supporting from G-COE program (Center for Medical System Innovation).
Declaration of interest: The authors report no conflicts of interest. The authors alone are responsible for the content and writing of the paper.
References
- Mauri L, Hsieh W, Massaro JM, Ho KKL, D’Agostino R, Cutlip DE. (2007). Stent thrombosis in randomized clinical trials of drug-eluting stents. N Engl J Med, 356, 1020–9.
- Kukreja N, Onuma Y, Daemen J, Serruys PW. (2008). The future of drug-eluting stents. Pharmacol Res, 57, 171–80.
- Ormiston JA, Serruys PW, Regar E, Dudek D, Thuesen L, Webster MW, Onuma Y, Garcia-Garcia HM, McGreevy R, Veldhof S. (2008). A bioabsorbable everolimus-eluting coronary stent system for patients with single de-novo coronary artery lesions (ABSORB): a prospective open-label trial. Lancet, 371, 899–907.
- Kim HI, Takai M, Ishihara K. (2009). The bioabsorbable materials containing phosphorylcholine group-rich surfaces for temporary scaffolding of the vessel wall. Tissue Eng, doi: 10.1089/ten.tea.2008.0307.
- Dzau VJ, Braun-Dullaes RC, Sedding DG. (2002). Vascular proliferation and atherosclerosis: new perspectives and therapeutic strategies. Nat Med, 8, 1249–56.
- Rainer W, Blaich B, BelAiba RS, Merl S, Görlach A, Kastrati A. (2007). Comparative characterization of cellular and molecular anti-restenotic profiles of paclitaxel and sirolimus. Thromb Haemost, 97, 1003–12.
- Serruys PW, Kutryk MJB, Ong ATL. (2006). Coronary-artery stents. N Engl J Med, 354, 483–95.
- Wang X, Venkatraman SS, Boey FYC, Loo JSC, Tan LP. (2006). Controlled release of sirolimus from a multilayered PLGA stent matrix. Biomaterials, 27, 5588–95.
- Alexis F, Venkatraman SS, Rath SK, Boey F. (2004). In vitro study of release mechanisms of paclitaxel and rapamycin from drug-incorporated biodegradable stent matrices. J Contr Rel, 98, 67–74.
- Ferron GM, Jusko WJ. (1998). Species differences in sirolimus stability in humans, rabbits, and rats. Drug Metab Dispos, 26, 83–4.
- Trepanier DJ, Gallant H, Legatt DF, Yatscoff RW. (1998). Rapamycin: distribution, pharmacokinetics and therapeutic range investigations: an update. Clin Biochem, 31, 1345–51.
- Duncan R. (2003). The dawning era of polymer therapeutics. Nat Rev Drug Discov, 2, 347–60.
- Diab C, Tribet C, Gohon Y, Popot J-L, Winnik FM. (2007). Complexation of integral membrane proteins by phosphorylcholine-based amphipols. Biochim Biophys Acta, 1768, 2737–47.
- Gohon Y, Giusti F, Prata C, Charvolin D, Timmins P, Ebel C, Tribet C, Popot J -L. (2006). Well-defined nanoparticles formed by hydrophobic assembly of a short and polydisperse random terpolymer, amphipol A8-35. Langmuir, 22, 1281–90.
- Ishihara K, Iwasaki Y, Nakabayashi N. (1999). Polymeric lipid nanosphere consisting of water-soluble poly(2-methacryloyloxyethyl phosphorylcholine-co-n-butyl methacrylate). Polym J, 31, 1231–6.
- Konno T, Watanabe J, Ishihara K. (2003). Enhanced solubility of paclitaxel using water-soluble and biocompatible 2-methacryloyloxyethyl phophorylcholine polymers. J Biomed Mater Res,65A, 210–5.
- Wada M, Jinno H, Ueda M, Ikeda T, Kitajima M, Konno T, Watanabe J, Ishihara K. (2007). Efficacy of an MPC-BMA co-polymer as a nanotransporter for paclitaxiel. Anticancer Res, 27, 1431–6.
- Pires NMM, van der Hoeven BL, de Vries MR, Havekes LM, van Vlijmen BJ, Hennink WE, Quax PHA, Jukema JW. (2005). Local perivascular delivery of anti-restenotic agents from a drug- eluting poly(ϵ-caprolactone) stent cuff. Biomaterials, 26, 5386–94.
- Ricciutelli M, Martino PD, Barboni L, Martelli S. (2006). Evaluation of rapamycin chemical stability in volatile-organic solvents by HPLC. J Pharm Biomed, 41, 1070–4.
- Diab C, Winnik FM, Tribet C. (2007). Enthalpy of interaction and binding isotherms of non-ionic surfactants onto micellar amphiphilic polymers (amphipols). Langmuir, 23, 3025–35.
- Tada S, Tarbell JM. (2004). Internal elastic lamina affects the distribution of macromolecules in the arterial wall: a computational study. Am J Physiol Heart Circ Physiol, 287, 905–13.
- Balakrishnan B, Dooley J, Kopia G, Edelman ER. (2008). Thrombus causes fluctuations in arterial drug delivery from intravascular stents. J Contr Rel. 131, 173–180.