Abstract
All-trans-retinoic acid (ATRA) is now included in many antitumor therapeutic schemes for the treatment of acute promyelocytic leukemia, Kaposi’s sarcoma, head and neck squamous cell carcinoma, ovarian carcinoma, bladder cancer, and neuroblastoma. Unfortunately, its poor aqueous solubility hampers its parenteral formulation, whereas oral administration of ATRA is associated with progressively diminishing drug levels in plasma, which is related to induction of retinoic acid-binding proteins and increased drug catabolism by cytochrome P450-mediated reactions. An ATRA formulation, obtained by complexation of the drug into polymeric micelles, might be suitable for parenteral administration overcoming these unwanted effects. To this purpose, amphiphilic polymers were prepared by polyvinylalcohol (PVA) partial esterification with nicotinoyl moieties and their functional properties evaluated with regard to ATRA complexation. The physicochemical characteristics of the polymers and the complexes were analyzed by 1H-NMR, Dynamic Light Scattering (DLS), Capillary Electophoresis (CE), and were correlated with the complex ability to improve the drug solubilization and release the free drug in an aqueous environment. Subsequently, the best complex, providing the highest ATRA solubilization and release, was evaluated in vitro to test its citotoxicity towards neuroblastoma cell lines. The PVA substitution degree calculated from 1H-NMR was found to be 5.0%, 8.2%, 15.3% (nicotinoyl moiety:PVA monomer molar ratio), while capillary electrophoresis analysis on the complexes revealed that the drug loadings were 0.95%, 1.20%, 4.76% (ATRA:polymer w:w) for PVA substitution degrees of 5.0%, 8.2%, and 15.3%, respectively. Complexation strongly increased ATRA aqueous solubility, which reached 1.20 ± 0.25 mg/mL. The DLS measurements of the polymers and the complexes in aqueous solutions revealed mean sizes always below 400 nm, low polydispersity (min 0.202 ± 0.013, max 0.450 ± 0.032), and size almost unaffected by concentration. Drug fractional release did not exceed 8% after 48 h. The cytotoxicity studies against neuroblastoma cell lines outlined a significant growth inhibition effect of complexed ATRA with respect to free ATRA. These data suggest that the systems analyzed may be suitable carriers for parenteral administration of ATRA and other hydrophobic antitumor drugs, where the carriers are required to improve drug aqueous solubility and delay drug release almost after their accumulation in solid tumors.
Introduction
Neuroblastoma is the most common extracranial solid tumor in childhood, and accounts for around 15% of all pediatric oncology deaths (Citation1). The cells responsible for this cancer originate in the neural crest and peripheral nervous system (PNS). Neuroblastoma cells sometimes differentiate spontaneously or after mild chemotherapy to produce a benign ganglioneuroma which results in spontaneous regression of desease.
From the above premise, the effectiveness of differentiation-inducing drugs such as retinoids appears clear (Citation2). All-trans retinoic acid (ATRA), in particular, induces in vitro differentiation of numerous neuroblastoma cells (Citation3), and, moreover, is effective in the treatment of many epithelial and hematological malignancies. FDA has already approved ATRA for the treatment of acute promyelocytic leukemia (Citation4) (Atragen®; Aronex Pharmaceuticals, Woburn, MA).
However, in spite of its antineoplastic in vitro activity, the existence of certain side effects such as retinoid acute resistance, hypertriglyceridemia, mucocutaneous dryness, and headache (Citation5,Citation6) strongly limits its clinical application. Moreover, a rapid decrease in half life (Citation7,Citation8), due to accelerated metabolism of ATRA by increased expression of cytochrome P450 enzymes and upregulation of the cytoplasmic retinoic acid binding proteins (CRABPs), takes place after repeated administrations of the drug in the chronic daily therapy (Citation9,Citation10).
Another important drawback of ATRA is its very low aqueous solubility strongly limiting its bioavailability. Various formulations of ATRA, mainly based on micelles, cyclodextrins, and liposomes, have already been examined in an attempt to increase the drug aqueoussolubility and thus its bioavailability and therapeutic efficacy (Citation11–14). The improved bioavailability of ATRA, allowing a decrease in the administered dose, would also overcome its side effects and rapid decrease in half-life.
In a previous study it has been proved that polymeric micelles are more effective in increasing the bioavailability of ATRA than liposomes (Citation15); therefore we designed new drug delivery systems based on amphiphilic polymers able to provide micelles in aqueous environment complexing ATRA in their hydrophobic inner cores. The amphiphilic polymers were obtained by polyvynilalcohol (PVA) partial esterification with nicotinoyl moieties (). These polymers provided core-shell type micellar structures upon dissolution in water (Citation16) containing hydroxyl groups on the surface and hydrophobic nicotinoyl moieties inside, able to complex ATRA. The hydrated shells protect the complexed drug from protein adsorption or attack from reticuloendothelial system (RES) (Citation17), while the cores behave as non-aqueous reservoirs of the drug, improving its aqueous solubilization and delaying its release during the micelle circulation in the bloodstream. Additionally, the control of micelle dimensions favors their accumulation in the tumor tissues by the enhanced permeability and retention (EPR) effect (Citation18). The physicochemical characteristics of the amphiphilic polymers and the complexes were analyzed by 1H-NMR, Dynamic Light Scattering (DLS), Capillary Electophoresis (CE), and were correlated with the complex ability to improve the drug solubilization and release the free drug in an aqueous environment. The best complex, providing the highest ATRA solubilization and release, was evaluated in vitro to test its cytotoxicity towards neuroblastoma cell lines.
Materials and methods
Materials
Polyvinylalcohol (Mw = 10,000 Da, 80% hydrolyzed) was a commercial sample from Aldrich Chemical Co. (USA); ATRA and nicotinoyl chloride were purchased from Sigma Chemical Co. (St. Louis, MO), all the other reagents and solvents employed were from Fluka Chemie GmbH (Buchs, Suisse).
Preparation of amphiphilic PVA (PVA-NIC)
The amphiphilic PVA (PVA-NIC) was prepared by linking the hydroxyl groups of PVA with the carbonyl moieties of nicotinoyl chloride following a procedure previously described for the synthesis of esters from alcohols (Citation19). Briefly, polyvinylalcohol (524.16 mg; 10 mmol of hydroxyvinylmonomer) was dissolved in 10 mL of N-methylpyrrolidone (NMP) at 50°C. The solution was subsequently supplemented with different nicotinoyl chloride amounts: 5% (0.5 mmol, 89.01 mg), 10% (1 mmol, 178.02 mg), or 20% (2 mmol, 356.04 mg), to obtain different PVA substitution degrees. Coupling reactions were incubated overnight at room temperature with an excess of triethylamine as a buffer, and finally supplemented with diethyl ether to induce precipitation of the substituted polymers. The solids obtained were re-precipitated twice from NMP and dried under vacuum to constant weight. Following this, the substituted polymers were dialyzed against water (3500 Da, Mw cut-off). Deionized water (1 L) was exchanged every 2 h for 6 h and then every 12 h for 48 h. Finally, the dialyzed solutions were freeze-dried and the solid products obtained were stored in a dessiccator. The substitution degrees in the final products were determined by 1H–NMR performed by an Inova 600 spectrometer, recording the spectrum in (CD3)SO.
Preparation of ATRA:PVA-NIC solid complexes
The ATRA:PVA-NIC solid complexes were prepared by coprecipitation from organic solvent. PVA-NIC (50 mg) was dissolved in 10 mL of NMP containing 10 mg of ATRA. After stirring at room temperature for 1 h, 50 mL of diethyl ether was added to induce ATRA:PVA-NIC coprecipitation. The precipitates obtained were collected by centrifugation (12,000 rpm, 0°C) and dried under vacuum to constant weight.
Evaluation of the complex drug loading
The complex drug loading was determined by capillary electrophoresis; the CE experiments were performed on a BIORad Biofocus 2000 instrument from BioRad (Hercules, CA). The data were collected on a personal computer equipped with the Biofocus System Integration software (BioRad). The separations were carried out on a fused-silica capillary of 50 μm internal diameter (ID) with a total length of 36 cm (effective length 31. 5 cm). The electrophoretic runs were performed at a constant voltage of 30.0 kV with controlled temperature (20°C). The samples were injected hydrodynamically using a pressure of 2 p.s.i. × s (1 p.s.i. = 6894 Pa); the detection wavelength was 345 nm. The running buffer was constituted of a 50% aqueous solution of sodium tetraborate (borax) (10 mM) and boric acid (50 mM; pH 8.5) and 50% acetonitrile. In order to obtain reproducible migration times, the capillary was rinsed between the runs with water (2 min) and running buffer (2 min).
The developed method was validated for linearity of the response in the concentration range of 0.01–0.500 mg/mL of ATRA; a 5-point calibration graph was obtained by triplicate injections for each standard ATRA solution (methanol), and the ratios (Y) of the corrected peak area (area/migration time) of the analyte vs the corrected peak area of the internal standard were plotted against the drug concentration (C; mg/mL). The linear regression analysis provided a correlation coefficient r > 0.999. The sensitivity of the method expressed as the limit of detection (LOD) corresponding to a signal-to-noise ratio (S/N) of approximately 3, was found to be 5 μg/mL; the limit of quantification can be considered as the lowest calibration point. The repeatability of migration time and corrected peak area was estimated by the relative standard deviation (RSD%) of five consecutive analyseis (n = 5) on a standard solution of ATRA (0.05 mg/mL); RSD% values corresponding to 0.9% and 2.6% were obtained for the migration time and corrected peak area, respectively. The samples were prepared by dissolving 1.0 mg of the solid complex in methanol; after ultrasonication for 15 min at room temperature (ultrasonic bath Bandelin Sonorex Super RK103H, Germany), a 0.200 mL aliquot of the obtained solution was diluted with 0.200 mL solution of Usnic acid (Fluka, Switzerland) dissolved in the borax-borate buffer (internal standard solution); the final concentration of the internal standard was 0.5 mg/mL. The quantitation of ATRA in the actual samples was performed by the external standard method, based on the comparison of the response (corrected peak area) of a standard solution of ATRA with that of the considered samples.
Solubilization studies of complexed ATRA
The solubility of complexed ATRA was evaluated by dispersing an excess of solid complex in 10 mL of water and, after stirring at 37°C for 24 h, filtering through 0.20 μm pore filter the suspension to eliminate the undissolved material. The clear solution obtained was freeze-dried and the solid obtained was evaluated by CE for its ATRA content following the above described procedure.
Dynamic light scattering measurements on polymer and complex aqueous solutions
The mean size of the micelles formed after polymer and complex dissolution in water were measured by Dynamic Light Scattering (DLS). The measurements were performed at concentrations ranging between 0.05–1.00 mg/mL. The aim was to gain information on their suitability in parenteral formulations and ability to extravasate in the capillary discontinuity of the solid tumors. The measurements were performed by a Brookhaven 90-PLUS instrument equipped with a 50 mW He-Ne laser (532 nm) thermo-regulated at 37°C. The scattering angle was fixed at 90°. Results were the combination of three 10-min runs for a total accumulation correlation function (ACF) time of 30 min. The mean size of the polymer aggregates in solution was provided by the average hydrodynamic radius. The results were volume-weighted.
ATRA release from complexes in aqueous solution
ATRA release from complexes was evaluated to assess the stability of the drug–polymer complexes in the aqueous body fluids after administration, and their ability to provide suitable amount of free drug after time periods compatible with extravasation in the solid tumors. The release studies were carried out by placing 1 mL of complex solution in water for a total complex content of 10 mg/mL in a releasing cell separated by a dialysis membrane (3500 Da, Mw cut-off) from a receiving compartment, containing 10 mL of phosphate buffered saline (PBS, 0.1 M, pH 7.4) and 4 mL chloroform. The membrane allowed permeation only to the free drug, released from the complex, and not to the drug–polymer complex whose mean molecular weight exceeded the membrane cut-off. The drug release from the polymer was followed by drug diffusion through the membrane and partition towards chloroform. The organic solvent behaved as an extractive phase for the free ATRA, assuring sink conditions throughout the experiment. The system was thermostated at 37°C with a stirring rate of 100 rpm. The whole release medium was exchanged with fresh medium at predetermined times. The organic phase was removed, evaporated, and the residue dissolved in methanol. The methanolic phase was analyzed for the determination of the free drug content by RP-HPLC. The HPLC assays were carried out by a Nova-Pak C18 (150 × 3. 9 mm, 4 mm, Waters) column, UV detector at 340 nm. The mobile phase was methanol isocratically eluted; the injecting volume 20 μL; the flow rate 1.0 mL/min. The system was thermostated at 37°C. In these chromatographic conditions retention time of ATRA was 11.00 min. These studies were performed in triplicate for each samples and the SD were less than 5%.
Biological studies
The human neuroblastoma-derived LAN-5 cell line was used. Cells were maintained in DMEM growth medium containing 10% fetal bovine serum and 100 ng/ml each penicillin and streptomycin (all from Sigma) at 37°C in 5% CO2. Experiments were performed during the logarithmic phase of cell growth. Cells were seeded in 6-well plates (Corning Incorporated, NY) (105 cells/well) as triplicates. After 48 h the cells were treated with growth medium containing 5 μM pure ATRA, 5 μM of complexed ATRA or an excess (25 μM) of the pure polymer (PVA-NIC). The effect of ATRA and ATRA:PVA-NIC on cell growth and death were determined by cell count and the trypan blue exclusion method. Cells maintained in medium alone and treated with pure polymer were used as controls. Culture medium and treatment agents were renewed after 3 days of culture. The results from biological experiments were statistically evaluated and analyzed using two-tailed unpaired t-test with Welch’s correction, with confidence intervals set at 99%.
Results and discussion
Characterization of the amphiphilic PVA
By 1H-NMR analysis, the substitution degrees of the polymers were obtained comparing the integral of the peak at 8.7 δ assigned to the aryl protons of pyridine with the integral of the peak at 1.95 δ assigned to the methyl protons (COCH3) of the acetyl moiety present at 20% in the PVA backbone. The substitution degrees were found to be 5.0%, 8.2%, 15.3% (nicotinoyl moiety:PVA monomer molar ratio), respectively, starting from 5%, 10%, 20% (nicotinoyl chloride:PVA monomer molar ratio in the preparative mixture). These data indicate that an efficient esterification of the PVA hydroxyls is obtained in the present conditions, being the substitution efficiency 100% at the lowest substitution degree (5%) and slightly decreasing at the higher substitution degrees (10% and 20%) in accordance with the growing steric hindrance associated with increased substitution.
The conjugates will be referred throughout the text as PVA-NIC 5%, PVA-NIC 8% and PVA-NIC 15%.
Drug loading of the solid complexes
Capillary electrophoresis analysis on the ATRA:PVA-NIC solid complexes revealed that the drug loadings were 0.95%, 1.20%, and 4.76% (w:w) for PVA-NIC 5%, PVA-NIC 8%, and PVA-NIC 15%, respectively. The highest ATRA loading was found in the most substituted polymer (PVA-NIC 15%) in accordance with the maximum presence of nicotinoyl moieties in the polymer backbone, which are expected to form hydrophobic domains, during the solid complex formation, trapping inside the hydrophobic drug. Indeed the precipitation of amphiphilic polymers in solutions may be obtained either by aggregation of the hydrophilic portions of the polymer or by aggregation of the hydrophobic ones. In the former event a non-polar solvent needs to be added to the amphiphilic polymer solution, in the latter a polar one. In the present study the ATRA:PVA-NIC solid complexes have been obtained by diethyl ether addition to the ATRA:polymer solutions in NMP. Diethyl ether is expected to cause desolvation of the polymer’s hydroxyl groups, with formation of solid precipitates containing tightly aggregated hydroxyls and loosely dispersed nicotinoyl moieties. These last ones, providing hydrophobic domains throughout the precipitate, may trap the solubilized ATRA, with efficiencies related to the nicotinoyl concentration and distribution in the different precipitates.
Solubilization studies of the complexed ATRA
Complexation with PVA-NIC strongly increased ATRA aqueous solubility: 0.60 ± 0.16 mg/mL (PVA-NIC 5%), 0.84 ± 0.14 mg/mL (PVA-NIC 8%), 1.20 ± 0.25 mg/mL (PVA-NIC 15%), with respect to the pure drug: 0.018 ± 0.004 mg/mL. This may be attributed to solid complexes ability to maintain interactions with ATRA also after dissolution in water, in accordance with the general behavior of amphiphilic polymers towards dissolution. Indeed, in the presence of water, the solid amphiphilic polymers may dissolve their hydrophilic portions which, in turn, trigger aggregation of the hydrophobic ones with formation of thermodynamically stable structures, i.e core-shell structures with shielded hydrophobic cores. In the same way, the dissolution of the solid ATRA:PVA-NIC complexes is driven by solubilization of the hydroxy vinyl portions of the polymers, triggering aggregation of the hydrophobic nicotinoyl-ATRA clusters. This provides soluble micellar complexes containing ATRA in their hydrophobic inner cores, which therefore retain the drug in solution at concentrations higher than its intrinsic solubility and dependent on the physico-chemical characteristics of the complex. The complex characterized by the highest substitution degree and drug loading (PVA-NIC 15% substitution degree, 4.76% drug loading) is also characterized by the highest solubilization ability towards ATRA (1.2 ± 0.25 mg/mL). This may be attributed to the increased presence of nicotinoyl domains in the solid complex, enhancing ATRA loading both in the solid and in the micellar complex formed after dissolution in water.
Dynamic light scattering measurements
The DLS measurements of PVA-NIC 5%, PVA-NIC 8%, and PVA-NIC 15% aqueous solutions revealed mean sizes always below 400 nm, low polydispersity (min 0.202 ± 0.013, max 0.450 ± 0.032) and size almost unaffected by concentration (). The increase in substitution degree slightly raised the size of the aggregates due to the presence of increasing nicotinoyl moieties on the polymer backbones. The DLS measurements of the aqueous solutions of the complexes revealed lower mean diameters of the complexes than the corresponding polymers, suggesting that the drug strictly associates with the hydrophobic micellar cores of the PVA-NIC polymers. Moreover, the complex solutions were stable for a month (data not shown), that is, particle size was not significantly changed and precipitants were not observed. These characteristics indicated suitability of the systems studied for i.v. injection, drug targeting (Citation20), and long-term storage.
Figure 2. Mean diameter (nm) of the micelles formed at 37°C in water by dissolution, at different polymer concentrations, of PVA-NIC 5% (▵), PVA-NIC 8% (◊), PVA-NIC 15% (□), ATRA:PVA-NIC 5% (▴), ATRA:PVA-NIC 8% (♦), and ATRA:PVA-NIC 15% (▪). Each value represents the mean ± SD of four independent experiments.
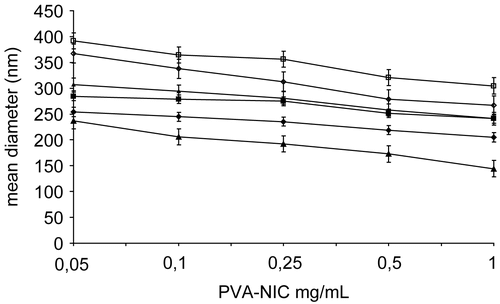
Study of ATRA release from the complexes
The release of the free drug from the complexes has been evaluated by the fractional release obtained by comparing the free drug concentration in the receiving phase to the total drug concentration solubilized in the releasing phase. The release rate of ATRA is higher from PVA-NIC 15%, followed by PVA-NIC 8%, and PVA-NIC 5%, respectively (). This may be explained considering that the drug release from the complexes is due to the drug partition between the polymer and the aqueous solution. This becomes a dynamic process, promoting drug dissociation from the complex, when absorption in vivo or the presence of a sink in vitro decrease the drug concentration in the aqueous solution. The complex therefore behaves as a reservoir of the free drug in solution, restoring the free drug concentration in equilibrium with the complexed drug.
Figure 3. Fractional release (%) of ATRA from micellar complexes in PBS at 37°C: ATRA:PVA-NIC 5% (▴), ATRA:PVA-NIC 8% (♦), and ATRA:PVA-NIC 15% (▪). Results are the mean ± standard error from four independent experiments.
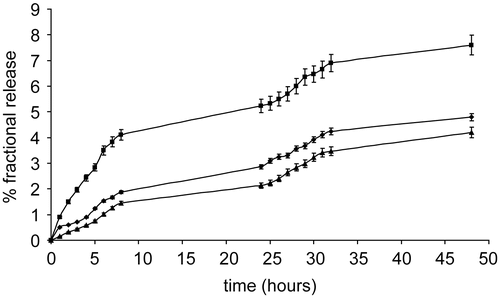
The diffusion through the hydrated polymeric shell of micellar complex is the rate-limiting step of the whole process and is favored by the less polymer amount per drug diffusing molecule, thus in the presence of the complex characterized by the highest drug loading (ATRA:PVA-NIC 15%).
The fractional release after 48 h did not exceed 8% in any of the studied systems, indicating stability towards drug release in the typical time lag required by injected drug-carriers to significantly accumulate in the solid tumor or its metastases. In this period the stability of the complex towards drug release is very important in order to prevent uncontrolled distribution of the drug in the body and, thus, unforeseen toxicity. On the other hand, the ability to release the drug over time is also essential to assure therapeutic levels once the carrier has been trapped in the solid tumor. Among the complexes analyzed, ATRA:PVA-NIC 15% meets both these features and is also characterized by the highest drug loading and solubilization ability towards ATRA. It may be thus considered the best one among the analyzed complexes.
Biological studies: Inhibition of neuroblastoma cell proliferation
The biological studies were performed on human neuroblastoma cell line LAN-5, which is well known to extensively differentiate upon treatment with ATRA (Citation21). Due to its improved characteristics among the analyzed systems, ATRA:PVA-NIC 15% was selected for the present study. Cell proliferation was inhibited in a time- and dose-dependent manner by free and complexed ATRA. To examine the kinetics of growth inhibition induced by free ATRA with respect to ATRA:PVA-NIC 15%, we performed time-course analyses setting drug concentration at 5 μM. LAN-5 cells did not change morphology, nor was their growth rate affected by treatment after 24 h. By 48 h of treatment, however, the ATRA-polymer complex induced a significant cytotoxic effect (p < 0.05), which became more evident at 72 h (p < 0.001) (), suggesting a more than additive effect between ATRA and the polymer alone. Morphologically, as expected, the cells treated with ATRA as a single agent underwent mostly growth arrest and extensive neuritic outgrowth (), whereas cells treated with ATRA-polymer complex showed a mixed effect consisting of both differentiation and cell detachment from the culture plate and cell death (). Growth trend, obtained by counting of viable cell, is depicted in . ATRA:PVA-NIC 15% enhanced the monolayer growth inhibition of free ATRA in LAN-5 cells (p < 0.0001). The higher activity of ATRA:PVA-NIC 15% than ATRA may be attributed to the improved aqueous solubilization of the complexed drug and the ability of the complex to provide a continuous supply of molecularly dispersed (solubilized) drug to the cells. Free ATRA, in contrast, is present in solution in molecular aggregates generated after dilution of the ethanolic solution in the aqueous medium (Citation22). The low dissolution rate of the molecular aggregates decreases the aqueous concentration of the solubilized drug and thus its bioavailability, reducing its antitumor activity. Taken together, the above findings suggest that our complexes are suitable for parenteral administration of ATRA, increasing its aqueous solubility, bioavailability, and biological efficacy. Further investigations focused on the behavior and effectiveness of this complex in animal models of NB xenotransplants are thus warranted.
Figure 4. Time course of the growth inhibiting and cytotoxic properties of pure ATRA (5 μM), complexed ATRA (5 μM), and pure polymer (PVA-NIC 15%, 25 μM) on human neuroblastoma LAN-5 cells. * p < 0.02; ** p < 0.001; *** p < 0.0001. White columns: control; black columns: pure polymer; gray columns: pure ATRA; dark gray columns: complexed ATRA.
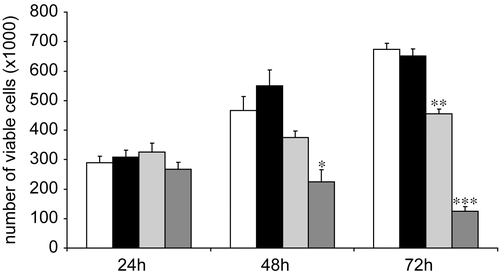
Acknowledgments
This work was carried out with MURST Funds (ex-60%).
Declaration of interest: The authors report no conflicts of interest. The authors alone are responsible for the content and writing of the paper.
References
- Marris JM, Hogarty MD, Bagatell R, Cohn SL. (2007). Neuroblastoma, Lancet, 369, 2106–20.
- Reynolds CK, Matthay KK, Villablanca JG, Maurer BJ. (2003). Retinoid therapy of high-risk neuroblastoma. Cancer Lett, 197, 185–92.
- Reynolds CP, Kane DJ, Einhorn PA, Matthay KK, Crouse VL, Wilbur JR, Shurin SB, Seeger RC. (1991). Response of neuroblastoma to retinoic acid in vitro and in vivo. Prog Clin Biol Res, 366, 203–11.
- Ozpolat B, Lopez-Berestein G. (2002). Liposomal-all-trans-retinoic acid in treatment of acute promyelocytic leukaemia. Leuk Lymphoma, 43, 933–41.
- Ragazzi MB, Iacona I, Gervasutti C, Lazzarino M, Toma S. (1997). Clinical pharmacokinetics of tretinoin. Clin Pharmacokinet, 32, 382–402.
- Adamson PC, Pilot HC, Balis FM, Rubin J, Murphy RF, Poplack DG. (1993). Variability in the oral bioavailability of all-trans-retinoic acid. J Natl Cancer Inst, 85, 993–6.
- Castaigne S, Chomienne C, Daniel MT, Ballerini P, Berger R, Fenaux P, Degos L. (1990). All-trans retinoic acid as a differentiation therapy for acute promyelocytic leukemia. I. Clinical results. Blood, 76, 1704–9.
- Muindi J, Frankel SR, Miller WH, Jakubowski A, Scheinberg DA, Young CW, Dmitrovsky E, Warrell RP. (1992). Continuous treatment with all-trans retinoic acid causes a progressive reduction in plasma drug concentrations: implications for relapse and retinoid ‘resistance’ in patients with acute promyelocytic leukaemia. Blood, 79, 299–303.
- Yamamoto Y, Zolfaghari R, Ross AC. (2000). Regulation of CYP26 (cytochrome P450RAI) mRNA expression and retinoic acid metabolism by retinoids and dietary vitamin A in liver of mice and rats. FASEB J, 14, 2119–27.
- Miller WH. (1998). The emerging role of retinoids and retinoic acid metabolism blocking agents in the treatment of cancer. Cancer, 83, 1471–82.
- Zuccari G, Carosio R, Fini A, Montaldo PG, Orienti I. (2005). Modified polyvinylalcohol for encapsulation of all-trans-retinoic acid in polymeric micelles. J Contr Rel, 103, 369–80.
- Lin HS, Chean CS, Ng YY, Chan SY, Ho PC. (2000). 2-Hydroxypropyl-β-cyclodextrin increases aqueous solubility and photostability of all-trans-retinoic acid. J Clin Pharm Ther, 25, 265–9.
- Lukyanov AN, Torchilin VP. (2004). Micelles from lipid derivatives of water-soluble polymers as delivery systems for poorly soluble drugs. Adv Drug Deliv Rev, 56, 1273–89.
- Ozpolat B, Lopez-Berestein G, Adamson P, Fu CJ, Williams AH. (2003). Pharmacokinetics of intravenously administered liposomal all-trans-retinoic acid (ATRA) and orally administered ATRA in healthy volunteers. J Pharm Pharm Sci, 6, 292–301.
- Kawakami S, Opanasopit P, Yokoyama M, Chansri N, Yamamoto T, Okano T, Yamashita F, Hashida M. (2005). Biodistribution characteristics of all-tran retinoic acid incorporated in liposomes and polymeric micelles following intravenous administration. J Pharm Sci, 94, 2606–15.
- Orienti I, Bigucci F, Gentilomi G, Zecchi V. (2001). Self-assembling poly(vinyl alcohol) derivatives, interactions with drugs and control of release. J Pharm Sci, 90, 1435–44.
- Duncan R. (2004). The dawning era of polymer therapeutics. Nat Rev Drug Disc, 2, 347–60.
- Maeda H, Wu J, Sawa T, Matsamura Y, Hori K. (2000). Tumor vascular permeability and the EPR effect in macromolecular therapeutics: a review. J Contr Rel, 65, 271–84.
- Orienti I, Zuccari G, Carosio R, Fini A, Rabasco AM, Montaldo PG. (2005). Modified doxorubicin for improved encapsulation in PVA polymeric micelles. Drug Del, 12, 15–20.
- Hobbes SK, Monsky WL, Yuan F, Roberts WG, Griffith L, Torchilin VP, Jain RK. (1999). Regulation of transport pathways in tumor vessels: Role of tumor type and microenvironment. Proc Natl Acad Sci, 95, 4607–12.
- Lanciotti M, Montaldo PG, Folghera S, Lucarelli E, Cornaglia-Ferraris P, Ponzoni M. (1992). A combined evaluation of biochemical and morphological changes during human neuroblastoma cell differentiation. Cell Mol Neurobiol, 12, 225–40.
- Orienti I, Zuccari G, Bergamante V, Fini A, Carosio R, Montaldo PG. (2007). Enhancement of oleyl alcohol anti tumor activity through complexation in polyvinylalcohol amphiphilic derivatives. Drug Deliv, 14, 209–17.