Abstract
The percutaneous absorption properties of daphnetin with chemical penetration enhancers were investigated to explore the feasibility of daphnetin as a candidate for transdermal delivery to treat arthritis. Permeation experiments were carried out in vitro using 2-chamber diffusion cells in isopropyl myristate (IPM) vehicle using rat abdominal skin as a barrier. Various enhancers were employed, including O-acylmenthol derivatives synthesized in the laboratory and many conventional enhancers. Among the O-acylmenthol derivatives, 2-isopropyl-5-methylcyclohexyl 2-hydroxypanoate (M-LA) demonstrated a significant enhancing effect on daphnetin permeation. The highest degree of enhancement was obtained when NMP combined with Span 80 and the cumulative transport was 667.29 μg/cm2 over 8 h. The solubility parameters, vehicle/stratum corneum partition, and diffusion coefficients were calculated to clarify the enhancing mechanism of classic enhancers on daphnetin. In conclusion, these findings allow a rational approach for designing an effective daphnetin transdermal delivery system.
Introduction
Skin has attracted much attention as an alternative route for drug administration. However, its potential use is often hindered by poor drug permeability through the skin, especially the stratum corneum (SC). Different methods have been used to overcome the barrier function of the SC (Citation1,Citation2). One of the most widely used techniques involves chemical penetration enhancers (Citation3). Although classic enhancers have a potent enhancing effect, many of them are toxic, allergenic, or irritate the skin to a degree depending on their concentration used. Therefore, the development of a new relatively safer enhancer is an attractive option for researchers.
A series of O-acylmenthol derivatives were synthesized in our laboratory using l-menthol and selected acids. Penetration experiments with five model western drugs, 5-fluorouracil (5-FU), isosorbide dinitrate, lidocaine, ketoprofen, and indomethacin, with a wide range of lipophilicity coefficient from −0.95–3.8, were preformed to investigate the promoting effect of these novel enhancers (Citation4,Citation5). Previous reports (Citation5) suggested that M-LA produced the greatest improvement on drugs with a log KO/W value of approximately 1, which was similar to that of daphnetin 0.82. The effect of O-acylmenthol on the hydrophilic drug 5-FU exhibited a parabolic relationship involving the alkyl chain length, and M-TET with a C14 alkyl chain had an optimal effect (Citation4). M-HEP and M-OA had general promoting effects on a broad range of selected model drugs (Citation4,Citation5). Therefore, M-LA, M-TET, M-HEP, and M-OA (the structures shown in ) were selected to investigate the promoting effect of O-acylmenthol derivatives on daphnetin.
Figure 1. The chemical structure of O-acylmenthol derivatives used as percutaneous penetration enhancers.
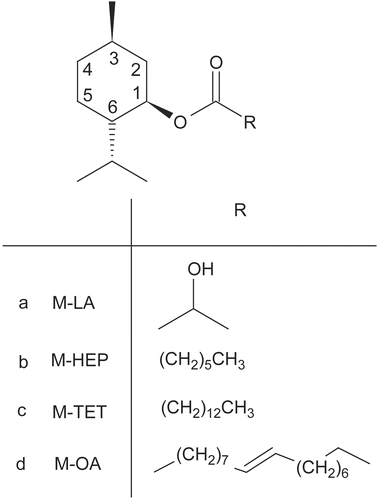
Daphnetin, a plant-derived dihydroxylated derivative of coumarin (the structure shown in ), has been approved as a valuable bioactive compound contained in Chinese herb Girald daphne bark, exhibiting a potent effect on anti-inflammatory effects in several pharmacological models (Citation6,Citation7). Daphnetin commercial preparations, including injections and oral tablets, are widely used to treat patients with arthritis. The recommended oral dosing regimen of daphnetin is 6 mg, three-times a day, and that by injection is 0.15–0.3mg, once or twice a day. The frequent and long-term administration of daphnetin for such a chronic disease makes the transdermal delivery a preferred pathway. Transdermal delivery system (TDS) offers a possible approach to overcome some of the drawbacks of other drug delivery methods: (a) improving compliance of patients suffering from chronic diseases; (b) ensuring an essentially constant drug plasma concentration; and (c) bypassing the gastrointestinal tract and liver as sites of metabolism which are responsible for the low oral bioavailability. Consequently, development of an effective TDS preparation of daphnetin is desirable to enhance patient compliance and obtain an ideal therapeutic effect.
Chemically, coarse girald daphne bark extract contains at least six major natural product chemical classes, including coumarins, diterpenoids, lignans, flavonoids, anthraquinones, and sterols (Citation8). However, side-effects, such as cutaneous irritation and insensible feeling induced by low polarity materials contained in coarse girald daphne bark extract has limited the development of daphnetin TDS (Citation9).
Accordingly, in this study, we purified Girald daphne bark extract to avoid its dermal side effects. Since there are only limited data on the transdermal properties of daphnetin, we investigated the feasibility of its transdermal application with chemical enhancers, including four O-acylmenthol derivatives and a wide range of classic penetration enhancers. We also studied the lipophilicity of daphnetin represented by log KO/W, solubility parameters of IPM and enhancers, vehicle/SC partition, and diffusion coefficient of daphnetin, with and without enhancers, to further clarify the intrinsic characteristics of daphnetin transdermal delivery.
Materials and methods
Daphnetin standard was purchased from the National Institute for the Control of Pharmaceutical and Biological Products (Beijing, China); girald daphne bark was obtained from the Liaoning traditional Chinese medical hospital (Liaoning, China); coumarin was obtained from Tiantai Chemistry Co. Ltd. (Tianjin, China); 2 -Isopropyl-5-methylcyclohexyl 2-hydroxypanoate (M-LA), (E)-2-isopropyl-5-methylcyclohexyl octadec- 9-enoate (M-OA), 2-isopropyl-5- methylcyclohexyl heptanoate (M-HEP), and 2-isopropyl-5-methylcyclohexyl tetradecanoate (M-TET) were synthesized in our laboratory; heptanoic acid, tetradecanoic acid, lactic acid, oleic acid, isopropyl myristate (IPM), and N-methyl- 2-pyrrolidone (NMP) were supplied by China National Medicines Co. Ltd. (Shanghai, China); menthol, Tween 80, Span 80, 2-(2-ethoxyethoxy) ethanol (Transcutol P), laurocapram (Azone), acetic ether (EtOAc), petroleum ether, and silica gel were purchased from the Yuwang Pharmaceutical Co. Ltd. (Shandong, China); propylene glycol (PG) and oleic acid were obtained from the Bodi Drug Manufacturing Co. Ltd. (Tianjin, China); macroporous resin D101 was purchased from Boen Co. Ltd. (Changzhou, China); methanol was of HPLC grade and was obtained from the Yuwang Pharmaceutical Co. Ltd. (Shandong, China). All other chemicals were of the highest reagent grade available.
HPLC determination of daphnetin
The determination of daphnetin was performed by high performance liquid chromatography (HPLC), using an internal calibration method with coumarin as internal standard. A Hitachi instrument (Pump L-7100, UV–VIS Detector L-7420, T2000L workstation) and a Kromasil C18 5 μm 200 mm × 4. 6 mm column were used. A mixture of methanol and distilled water containing 0.5% acetic acid (40:60, v/v) was used as the mobile phase. The column was maintained at 40°C, and the flow rate was 1 ml/min while the wavelength was 325 nm. Retention times in this assay were found to be 4.45 and 8.07 min for daphnetin and internal standard, respectively. The peak of daphnetin was well separated by this analytical method from those of the internal standard and other constituents contained in the herb, and there was no interference from any endogenous components.
Determination of daphnetin oil–water partition coefficient (Log KO/W)
The log KO/W value of daphnetin was determined using daphnetin standard, n-octanol as the oil phase and phosphate buffer adjusted to pH 7.4 as the aqueous phase. The phase volume ratio was 1:1. After equilibrating for 24 h in a water bath at 32°C, the organic phase and aqueous phase were separated and the concentrations of daphnetin were assayed. The oil–water partition coefficient was calculated as
where CO is the concentration of daphnetin in the oil phase and CW is the concentration of daphnetin in the aqueous phase (Citation10).
Preparation of refined girald daphne bark extract
Dried girald daphne bark (1000 g) was extracted twice with 70% ethanol using ultrasound: the first time was for 60 min with an 8-fold volume of solvent and the second time was for 30 min with a 6-fold volume of solvent. After removing ethanol in a rotary evaporator, the concentrated aqueous suspension was extracted three times with an equal volume of EtOAc. The dried EtOAc extract was subjected to silica gel column chromatography, and eluted with a gradient petroleum ether-EtOAc system with a ratio from 9:1 to 2:1. Fractions, each of 250 ml, were collected followed by determination by HPLC as described. The fractions containing daphnetin without other constituent peaks were collected and combined, solvent was removed, and the refined girald daphne bark extract was obtained.
Preparation of donor solutions
Donor solutions, with and without chemical enhancers, were obtained by placing predetermined methanol solutions of refined girald daphne bark extract in IPM and then removing the methanol in a rotary evaporator at a temperature lower than 50°C. Selected concentration enhancers were added, followed by a 5 min ultrasound treatment to obtain a uniform system. The content of daphnetin (1.41 mg/1 g IPM) in the donor compartments was based on obtaining sink conditions throughout the permeation experiments.
Determination of daphnetin concentration in the donor vehicles
The concentrations of daphnetin in donor compartments, with and without chemical enhancers, were determined. For this, 0.5 ml prepared donor solution was sealed in 1 ml polypropylene micro-vials and equilibrated in a water bath at 32°C for 12 h. A shaking time longer than 12 h was not chosen, because the instability of the two hydroxyls in the daphnetin structure did not allow this. Finally, the solutions were centrifuged at 10,000 rpm for 10 min and the supernatants were diluted appropriately and analyzed by the HPLC method as described. The experiments were performed in triplicate.
Preparation of rat skin
Male Wistar rats weighing 180–220 g (6–8 weeks old) used in all experiments were supplied by the Experimental Animal Center of Shenyang Pharmaceutical University (Shenyang China). The experiments were performed in accordance with the guidelines for animal use published by the Life Science Research Center of Shenyang Pharmaceutical University. The rats were anesthetized with urethane (20%, w/v, i.p.) and the abdomen was carefully shaved with a razor after removal of hair by electric clippers (model 900, TGC, Japan). Full thickness skin (i.e. epidermis with SC and dermis) was excised from the shaved abdominal site, followed by careful separation of the skin from the connective fat and tissues. Skin was carefully checked using a magnifying lens to ensure the skin surface had no obvious defects. Then, the skin was kept frozen at −20°C and used within 2 weeks. Before starting the experiments, the skin was allowed to reach room temperature for at least 10 h.
In vitro transdermal permeation experiments
Skin permeation experiments were performed at 32°C in 2-chamber diffusion cells according to the method of Fang et al. (Citation11). Each half-cell had a volume of 2.5 ml and an effective area of 0.95 cm2 (Citation11). Rat skin was mounted between donor and receiver cells, with the SC side of the skin facing the donor compartment and the dermis side facing the receiver compartment. Excess skin at the sides was trimmed off to minimize lateral diffusion. The donor compartment was filled with donor solution and the receiver compartment with pH 7.4 phosphate buffer solution (PBS). During all permeation experiments, the daphnetin concentration in the donor cell was more than 10-times that in the receiver cell to maintain sink conditions. Both donor and receiver compartments were stirred with a star-head bar driven by a constant speed synchronous motor at 600 rpm. At predetermined time intervals, 2.0 ml of receptor solution was withdrawn from each receiver compartment for analysis and replaced with the same volume of fresh pH 7.4 PBS to maintain sink conditions. All the experiments were repeated at least four times. The daphnetin concentration was determined by reversed phase HPLC with reference to the calibration curve.
Data analysis
The cumulative amount of daphnetin permeating through rat skin was plotted vs time. The skin flux was determined from Fick’s law of diffusion:
where Js is the steady-state flux in μg/cm2/h, dQr is the change in quantity of the drug passing through the skin into the receptor compartment in μg, A is the active diffusion area in cm2, and dt is the change in time in h. The cumulative amount of daphnetin permeating through skin in 8 h (Q8) was calculated from the drug concentration in the receiver compartment. The flux was calculated from the slope of the linear portion of the profiles. The lag-time (Tlag) was determined by extrapolating the linear portion of the curve to the abscissa (Citation12).
To evaluate the promoting activity of each enhancer, the enhancement ratio (ER) was calculated as,
Solubility parameter, δ, is calculated using the expression,
where ΔEv is the energy of vaporization at 25°C in J/mol and V is the corresponding molar volume in cm3/mol (Citation13).
The permeability coefficient (Citation14), vehicle/SC partition, and diffusion coefficient were calculated as,
where P is the permeability coefficient, Cs is the concentration of daphnetin in donor solutions, K is the vehicle/SC partition coefficient, D is the diffusion coefficient in mm2/h, Tlag is the lag time in h, and H is the diffusion path length.
Since most molecules permeate through the SC mainly by a tortuous intercellular route, the thickness of the skin is not equal to the diffusion path length (Citation15). However, it is difficult to determine the exact path length of the permeant from vehicles. Therefore, the partition coefficient (K) and diffusivity (D) were normalized as K’ (KH) and D’ (D/H2), respectively (Citation15), and these can be obtained from the following equations:
For comparison between two groups of data, significance was determined by Student’s t-test. Differences were considered statistically significant at p < 0.05.
Results and discussion
Preparation and purification of Girald daphne bark extract
Dilution curves of daphnetin and its associated material were plotted as the peak areas of materials determined by HPLC in each 250 ml fraction vs the corresponding diluting volume (from the ratio 3:1 to the end), as shown in . From the profiles, we can see that the peak of daphnetin was sharp and almost baseline separation was obtained from its neighbors a, b, and other unrelated materials at 325 nm. The final yield of daphnetin from girald daphne bark by the optimized method was about 0.60‰, with the daphnetin purity of the final product being about 30%.
Girald daphne bark has been studied by other researchers (Citation16) using extraction and separation methods, mainly in order to discover novel compounds and characterize their pharmacological activity. In our case, the daphnetin yield was the basic parameter during the optimization of extraction and purification methods to increase the efficiency of resource use. By this method, the maximum yield of daphnetin was achieved and toxic material of low polarity was removed to avoid any cutaneous side-effects. Traditional refluxing for 2 h, and ultrasound extraction for 0.5 h, 1 h, and 2 h were compared here. Data obtained (not shown) showed that ultrasound extraction for 1 h was much better than the other extraction methods with regard to the time needed consuming and total yield obtained due to daphnetin heat instability. As a result, extraction for 1 h by ultrasound was employed to prepare a coarse extract with a daphnetin yield of about 0.82‰.
Two purification methods were compared here. The relative yields of daphnetin (RY) (taking the daphnetin quantity in the coarse extract as 100%) after every step of the purification procedures are shown in . As we can see, the profile obtained from the silica gel method was much smoother than that from the macroporous resin method, with final relative yields of 73.2% and 8.3%, respectively. This result is likely due to the frequent use of a low concentration ethanol–water solution in the macroporous resin method, because daphnetin is unstable under such conditions. As a result, silica gel was finally used as the material for daphnetin purification.
Figure 4. Relative yield (RY) of daphnetin during purification procedures by silica gel method (a) and macroporous resin method (b). Steps in (a): 0. extracted with 70% ethanol by ultrasound; 1. removal of the ethanol in a rotary evaporator; 2. extraction with an equal volume of EtOAc; 3. purification with silica gel and removal of vehicle. Steps in (b): 0. extracted with 70% ethanol by ultrasound; 1. removal of ethanol in a rotary evaporator; 2. purification with macroporous resin; 3. evaporation of ethanol and concentration of daphnetin aqueous solution; 4. extraction with EtOAc and removal of vehicle.
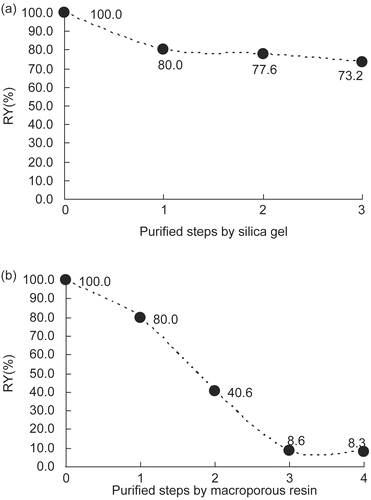
Penetration-enhancing effect of O-acylmenthol derivatives
Novel enhancers of O-acylmenthol derivatives synthesized in our laboratory, which had potent enhancing effects on five western model drugs (Citation4,Citation5), were tested here to investigate their potential as far as daphnetin activity was concerned. The accumulated amounts of daphnetin in the receptor compartments vs time are plotted in . From the profiles, we can see that M-LA has a very significant enhancing effect on daphnetin penetration, while the other O-acylmenthol derivatives had no obvious enhancing effect. The calculated parameters of daphnetin with these O-acylmenthol derivatives are shown in .
Figure 5. Permeation profiles of daphnetin through rat skin with O-acylmenthol derivatives (average ± SD, n = 4).
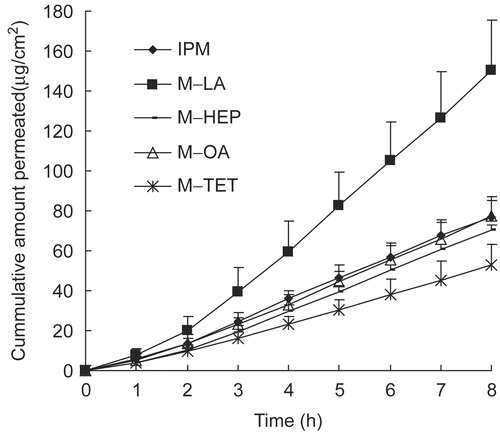
Table 1. Steady-state flux (Js), lag-time (Tlag), concentration in donor vehicle (C), permeability coefficient (P), and enhancement ratio (ER) of daphnetin. The receiver phase used pH 7.4 PBS and the donor phase consisted of IPM and 5% chemical enhancers (m/m).
M-LA greatly enhanced daphnetin penetration, which agreed well with the published literature (Citation5). The superior effect of M-LA was probably due to its hydroxyl moiety inducing hygroscopic properties, exerting a skin hydration effect, and, consequently, facilitating the partition of water-soluble compounds into the skin and permeation across the skin (Citation17). In addition, the hydroxyl group contained in the M-LA structure might form hydrogen bonds with those in the ceramide molecule (which plays a major role in the skin lipid barrier function) (Citation18). It was postulated that these hydrogen bonds between M-LA and the ceramide head group could, initially, rupture the interlamellar hydrogen bonding networks in the SC, increasing the distance between the two opposite lamellae and forming new pathways or channels for daphnetin passage and, then, weaken the hydrogen bonds between daphnetin and ceramides, which hindered daphnetin transport through the skin. However, further investigation is required to prove this hypothesis. Nevertheless, for the other O-acylmenthol derivatives tested, generalization of previous published results does not appear to be realistic. Unlike the reports in the literature, the other three O-acylmenthol derivatives had no significant promoting effects on daphnetin transport, even producing a slight reduction. It is obvious that the lipophilicity alone cannot explain the interactions between vehicle, drugs, and skin in all cases. It is likely that the skin permeation flux reflects a combination of enhancer, solvent, and global characteristics of the permeant such as the size, shape, lipophilicity, and special groups in the molecule.
Penetration-enhancing effect of conventional chemical enhancers
To improve the transdermal permeation of daphnetin, experiments were carried out in vitro using daphnetin together with a wide range of conventional chemical penetration enhancers. A concentration of 5% was used throughout the experiments. The accumulated amounts of daphnetin in the receptor compartments as a function of time for all the conditions assayed in this part are plotted in . The effect of different penetration enhancers on the permeation parameters of daphnetin (flux, Tlag, concentration in donor vehicle, permeation coefficient, and enhancement ratio) are presented in .
Figure 6. Permeation profiles of daphnetin through rat skin with conventional penetration enhancers (average ± SD, n = 4).
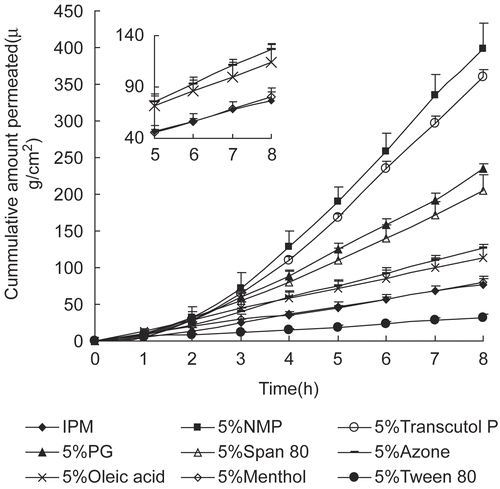
As shown in , NMP exhibited the most significant enhancing effects on daphnetin percutaneous absorption, with the cumulative amount being 398.96 μg/cm2. Despite not being superior to NMP representing, Transcutol P, PG, and Span 80 also had potent enhancing effects, with the cumulative amounts being 360.35, 234.88, and 204.29 μg/cm2, respectively. Oleic acid and Azone exhibited moderate promoting activity. Menthol had no effect on daphnetin penetration as the cumulative amount was 80.23 μg/cm2, almost the same as that of control (76.68 μg/cm2). A significant reduction was observed when surfactant Tween 80 was used in the IPM system, with the cumulative amount being 32.39 μg/cm2. Overall, the different chemical enhancers exhibited significantly different promoting activity on daphnetin transport across rat skin.
Yoneto et al. (Citation19,Citation20) reported that N-alkyl-pyrrolidone derivatives increased drug solubility in skin and also fluidized lipids in the stratum corneum. However, NMP possessing a very short alkyl chain might not contribute much to the lipid fluidity, and thus have little effect on the drug diffusion through the SC. Therefore, increasing drug solubility in the skin might be the main enhancing mechanism of NMP on daphnetin transdermal penetration. This result is supported by the observation of Koizumi et al. (Citation21), who believed that NMP partitioned into the skin, further increased estradiol solubility in the skin, and, consequently, improved the permeation of estradiol.
Transcutol P had a slightly lower promoting effect on daphnetin percutaneous absorption compared with NMP. The superior potency of Transcutol P as a penetration enhancer could be attributed to an increased drug solubility in the SC (Citation22) and creation of a depot in the SC and upper epidermal layers resulting in drug penetration enhancement (Citation23).
PG is widely used as a vehicle for penetration enhancers and has also been used as a penetration enhancer in its own right. PG produced its promoting effect by increasing the drug solubility in the vehicle and altering the tissue solubility of the drug (Citation24). However, from our results shown in (column 4) and (column 2), PG exerted an enhancing effect by increasing daphnetin solubility in the SC (K’) rather than in vehicle (concentration in donor vehicle).
Table 2. Vehicle/SC partition and diffusion coefficients of daphnetin, and solubility parameters of enhancers and IPM. The receiver phase used pH 7.4 PBS and the donor phase consisted of IPM and 5% chemical enhancers (m/m).
The promoting effect of surfactant Span 80 on daphnetin penetration may be related to its reduction of the surfactant tension between the hydrophilic drug and the lipophilic intercellular lipid matrix of the stratum corneum, facilitating daphnetin partition into the SC.
Oleic acid and Azone are believed to produce their enhancing effects through direct interaction with SC lipids, disrupting the molecular arrangement, leading to a more fluid environment, producing an increased diffusion coefficient of permeant, and, thus, increasing drug penetration through the skin (Citation25–27). However, they demonstrated only a moderate promoting potential on daphnetin in the IPM system.
Generally, since different enhancers produced significantly different enhancing effects on daphnetin (shown in ), three factors are summarized in terms of their interactions with (a) skin (SC), (b) vehicle, and (c) drugs.
Enhancer–skin (SC)
Drug permeation depends on its partition from the vehicle (formulation) into the SC and its diffusion through the membrane from the lipophilic region (SC) to the aqueous region (viable tissue) (Citation28). An interesting rule finding can be concluded from the permeation data, namely that the enhancers working by increasing the partition coefficient of the permeant into SC, such as NMP and Transcutol P, have a more significant effect than that by changing the diffusion coefficient through the SC, such as Azone and oleic acid.
The partition of a compound into skin, a critical procedure for transdermal delivery, depends on a number of physicochemical properties of the permeant, especially its lipophilicity. Daphnetin, possessing two hydroxyls, is relatively hydrophilic with a log KO/W value of 0.82. Thus, it is more difficult for daphnetin to partition into the SC which mainly consists of lipophilic materials. This might explain the phenomenon that enhancers that involve the partition step have a more potent effect than those which act on the diffusion step. To find proof for this assumption, the vehicle/SC partition coefficient (K’) and diffusion coefficient (D’) based on permeation data were calculated (shown in ). As we can see from (columns 2 and 3), the steady-state flux grew up as the partition coefficient K’ increased, which agrees well with our hypothesis. It can be concluded that partition into SC lipid is the rate-limiting step for hydrophilic compounds with a log KO/W value below 1, and, thus, enhancers exerting their promoting effect by increasing the retention of the drugs within the SC exhibit a more potent effect. However, in the case of lipophilic compounds, enhancers that facilitate drug passage through the lipid layer into aqueous tissue might be more efficient.
Enhancer–vehicle
The solubility parameters of the enhancers and vehicle IPM were calculated according to the method published by Fedors (Citation13) shown in . The more similar the solubility parameters of the two chemicals, the more compatible they are when mixed. Enhancers having a very different solubility parameter from the vehicle, such as NMP, Transcutol P, and Span 80, with Δδenhancers, IPM values of 2.74, 2.92, and 4.70, respectively (shown in , column 5), could be released easily from the vehicle onto the skin surface to produce superior enhancing effects. However, it was hard for enhancers with a very similar solubility parameter to that of the vehicle, such as oleic acid and Azone, with Δδenhancers, IPM values of 0.90 and 0.73, to be released from the vehicle onto the skin surface, and, thus, they had only moderate enhancing effects. However, PG which had the largest difference in the solubility parameter from IPM produced a lower cumulative amount than NMP and Transcutol P, this was mainly because that the difference was so significant that PG was incompatible with IPM, forming two phases in the donor solution, and, consequently, preventing its enhancing effect. We can conclude that for the enhancers compatible with the vehicle, the greater the difference in the solubility parameter between enhancers and solvent, the greater the cumulative amount permeating from the vehicle.
Enhancer–drug
Certain interactions, such as hydrogen bonding, might take place between the permeant and enhancer. NMP, Transcutol P, PG, and Span 80 have excellent enhancing effects which might be due to the hydroxyl groups and polar oxygen atoms in their structures interacting with the two hydroxyl groups in daphnetin, and, consequently, improving the ability of daphnetin to permeate into the skin, as suggested by Garcia et al. (Citation29), who believed that interactions between drug and other substances in vehicle may result in changes in drug physicochemical properties leading to increased drug permeation. However, further proof is still required.
Synergistic effect of enhancers
Single chemicals, however, offer limited improvement of skin permeability. Mixtures of chemicals can overcome this limitation owing to their synergistic interactions. As the results obtained before, NMP had a maximum enhancing effect on daphnetin transdermal absorption. Whether a synergistic effect existed between NMP and other enhancers was investigated in this part. Transcutol P and Span 80, which exhibited a stronger enhancing effect compared with the other enhancers, were applied here in the combination with NMP to study the synergistic effect of enhancers on daphnetin permeation through rat skin. Surprisingly, as shown in , the two most effective transdermal enhancers, NMP and Transcutol P, produced reduced cumulative amounts when used simultaneously in the IPM system, even lower than that of either enhancer used alone. However, as shown in , NMP and Span 80 had a potent synergistic effect on daphnetin transdermal penetration, with a cumulative transport of 667.29 μg/cm2 in 8 h.
Figure 7. Permeation profiles of daphnetin through rat skin with synergistic enhancers (a) 5% NMP + 5% Transcutol P, and (b) 5% NMP + 5% Span 80 (average ± SD, n = 4).
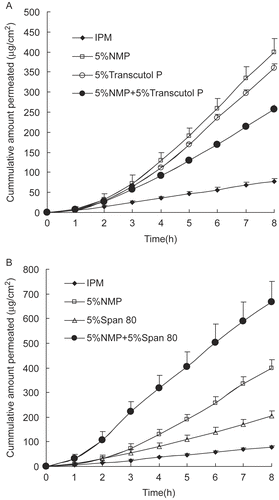
As discussed previously, the superior enhancing effect on daphnetin by NMP was probably due to the increased drug solubility in the SC barrier, which was similar to that of Transcutol P. Thus, combination of these two enhancers with a similar enhancing mechanism may not demonstrate a significant synergistic effect. However, such a marked reduction needs further clarification.
Non-ionic surfactants tend to be widely regarded as safe, generally having low chronic toxicity, and most have been shown to increase the flux of materials permeating through biological membranes (Citation24). Span 80 is a non-ionic surfactant with a polar head combined with a long alkyl chain making the SC layer less rigid by inserting its C16 alkyl chain into the lipid domain, as occurs with other surfactants, such as Span 20 (Citation30). The effect of Span 80 as a penetration enhancer could be attributed to the improvement in drug diffusion through the SC barrier and reduction of the surfactant tension between daphnetin and the SC, which is different from that of NMP. Consequently, a potent synergistic effect was obtained when Span 80 and NMP were both present. In addition, daphnetin extract could disperse homogeneously in pressure sensitive adhesive (PSA) with the aid of surfactant Span 80 when prepared in the form of patches. As a result, the chemical enhancer NMP with Span 80 was probably the optimal combination for daphnetin TDS preparations such as patches for the clinical treatment of arthritis.
Conclusion
The data from the in vitro transdermal permeation study showed that among O-acylmenthol derivatives, M-LA, with an α-hydroxyl group, produced a significant improvement in daphnetin transport across rat skin. NMP, exerting its enhancing activity by mainly influencing the partition parameter, produced the greatest percutaneous penetration enhancement. In addition, the highest accumulative amount permeated was obtained in the presence of both NMP and Span 80. Overall, this study provides preliminary data to assist in the development of effective daphnetin TDS preparations, such as patches. However, further investigation of the transdermal delivery of daphnetin from PSA with these excellent chemical enhancers, including M-LA, NMP, and Span 80, is now in progress.
Acknowledgments
Declaration of interest: The authors report no conflicts of interest. The authors alone are responsible for the content and writing of the paper.
References
- Prausnitz MR, Mitragotri S, Langer R. 2004. Current status and future potential of transdermal drug delivery. Nat Rev Drug Discov 3:115–124.
- Hadgraft J, Lane M. 2006. Passive transdermal drug delivery systems: recent considerations and advances. Am J Drug Deliv 4:153–160.
- Benson HAE. 2005. Transdermal drug delivery: penetration enhancement techniques. Curr Drug Deliv 2:23–33.
- Zhao LG, Fang L, Xu YN, Liu S, He ZG, Zhao YY. 2008. Transdermal delivery of penetrants with differing lipophilicities using O-acylmenthol derivatives as penetration enhancers. Eur J Pharm Biopharm 69:199–213.
- Zhao LG, Fang L, Xu YN, Zhao YY, He ZG. 2008. Effect of O-acylmenthol on transdermal delivery of drugs with different lipophilicity. Int J Pharm 352:92–103.
- Zhang WL, Li RH. 2007. Advanced review of daphnetin pharmacological effect. Shi yong zhong yi zha zhi 23:402–403.
- Gao Q, Shan J, Di L, Jiang L, Xu H. 2008. Therapeutic effects of daphnetin on adjuvant-induced arthritic rats. J Ethnopharmacol 120:259–63.
- Li SH, Wu LJ, Yin HY. 2002. Chemical and pharmacological advances of the study on zushima. Zhongguo Zhong Yao Za Zhi 27:401–403.
- Li CL, Ye YW, Liang ZF. 1983. Comparison of dapne extrat before and after removal of poison constituent. Zhong guo yi yuan yao xue za zhi 3:318–319.
- Sales OD, Castellano AL, Lacer FJM, Dominguez MH. 1993. An in vitro percutaneous absorption study of non-ionic compound s across human skin. Pharmazie 48:684–686.
- Fang L, Kobayashi Y, Numajiri S, Kobayashi D, Sugibayashi K, Morimoto Y. 2002. The enhancing effect of a triethanolamine–ethanol–isopropyl myristate mixed system on the skin permeation of acidic drugs. Biol Pharm Bull 25:1339–1344.
- Niazy EM. 1996. Differences in penetration-enhancing effect of Azone throughexcised rabbit, rat, hairless mouse, guinea pig and human skins. Int J Pharm 130:225–230.
- Fedors RF. 1974. A method for estimating both the solubility parameters and molar volumes of liquids. Polym Eng Sci 14:147–154.
- Scheuplein RJ. 1978. Site variations in diffusion and permeability. In: Jarret A, ed. The Physiology and Pathophysiology of Skin. New York: Academic Press, 1693–1730.
- Williams AC, Barry BW. 1991. Terpenes and the lipid-protein partitioning theory of skin penetration enhancement. Pharm Res 8:17–24.
- Ullah N, Ahmed S, Muhammad P, Ahmed Z, Nawaz HR, Malik A. 1999. Coumarinolignoid glycoside from Daphne oleoides. Phytochemistry 51:103–105.
- Kraeling MEK, Bronaugh RL. 1997. In vitro percutaneous absorption of alpha hydroxyl acids in human skin. J Soc Cosmet Chem 48:187–197.
- Narishetty ST, Panchagnula R. 2004. Transdermal delivery of zidovudine: effect of terpenes and their mechanism of action. J Contr Rel 95:367–379.
- Yoneto K, Ghanem AH, Higuchi WI, Peck KD, Li SK. 1995. Mechanistic studies of the 1-alkyl-2-pyrrolidones as skin permeation enhancers. J Pharm Sci 84:312–317.
- Yoneto K, Li SK, Higuchi WI, Shimabayashi S. 1997. Influence of the permeation enhancers 1-alkyl-2-pyrrolidones on permeant partitioning into the stratum corneum. J Pharm Sci 87:209–214.
- Koizumi A, Fujii M, Kondoh M, Watanabe Y. 2004. Effect of N-methyl-2-pyrrolidone on skin permeation of estradiol. Eur J Pharm Biopharm 57:473–478.
- Williams AC, Megrab NA, Barry BW. 1995. Permeation of oestradiol through human epidermal and silastic membranes from saturated transcutol/water systems. In: Brain KR, Walters KA, James VJ, eds. Prediction of percutaneous penetration. Cardiff: STS Publishing, pp. 43.
- Ritscel WA, Panchagnula R, Stemmer K, Ashraf M. 1991. Development of an intracutaneous depot for drugs. Binding, drug accumulation and retention studies, and mechanisms of depot. Skin Pharmacol. 4:235–245.
- Williams AC, Barry BW. 2004. Penetration enhancers. Adv Drug Deliv Rev 56:603–618.
- Harrison JE, Watkinson AC, Green DM, Hadgraft J, Brain K. 1996. The relative effect of Azone and Transcutol on permeant diffusivity and solubility in human stratum corneum. Pharm Res 13:542–546.
- Moser K, Kriwet K, Naik A, Kalia YN, Guy RH. 2001. Passive skin penetration enhancement and its quantification in vitro. Eur J Pharm Biopharm 52:103–112.
- Lewis D, Hadgraft J. 1990. Mixed monolayers of dipalmitoylphospatidylcholine with Azonew or oleic acid at the air–water interface. Int J Pharm 65:211–218.
- Brown MB, Hanpanitcharoen M, Martin GP. 2001. An in vitro investigation into the effect of glycosaminoglycans on the skin partitioning and deposition of NSAIDs. Int J Pharm 225:113–121.
- Garcia MT, da Silva CH, de Oliveira DC, Braga EC, Thomazini JA, Bentley MV. 2006. Transdermal delivery of ketoprofen: the influence of drug-dioleylphosphatidylcholine interactions. Pharm Res 23:1776–1785.
- Copoví A, Díez-Sales O, Herráez-Domínguez JV, Herráez-Domínguez M. 2006. Enhancing effect of alpha-hydroxyacids on ‘in vitro’ permeation across the human skin of compounds with different lipophilicity. Int J Pharm 314:31–36.