Abstract
Multidrug resistance (MDR) caused by high expression of P-glycoprotein (Pgp) in cancer patients remains one of the major obstacles to successful therapy of cancer. Earlier studies have shown that the incorporation of Pgp-substrate drugs in liposomes may provide a strategy to circumvent Pgp-mediated drug efflux. The present study investigated the impact of liposome composition on the efflux of Pgp-substrate incorporated in liposomes. Liposomes with varying compositions were loaded with rhodamine 123, a fluorescent probe frequently used as a Pgp-substrate, and the retention of rhodamine was compared in two breast cancer cell lines: wild-type cells with no detectable Pgp expression (MCF-7/WT) and Pgp-expressing cells resulting from stable transfection of the MDR1 gene (MCF-7/Pgp). Pgp-expression decreased the rhodamine retention in MCF-7 cells, suggesting that Pgp is functional. Liposome loading increased rhodamine retention in MCF-7/Pgp cells, but not in MCF-7/WT cells. Surface charge of liposomes did not affect the rhodamine retention, whereas the incorporation of cholesterol and polyethyleneglycol-attached lipids was effective in further increasing the rhodamine retention in MCF-7/Pgp cells. Since further study demonstrated that the rate of rhodamine release from liposomes tended to be inversely correlated with rhodamine retention by cells, it seems likely that more rigid liposomes are able to sequester rhodamine more efficiently, thereby inhibiting direct interactions of rhodamine with Pgp proteins. Taken together, these findings suggest that Pgp-mediated MDR in cancer cells may be more effectively modulated by optimizing the composition of liposomes for loading Pgp-substrate anti-cancer drugs.
Introduction
Intrinsic or acquired resistance to chemotherapeutic drugs is one of the major obstacles to effective cancer treatment. The most frequent form of resistance observed in cancer patients is multidrug resistance (MDR), which is characterized by cross-resistance to a wide variety of structurally unrelated drugs, including the anthracyclines, some vinca alkaloids, and the epipodophyllotoxins (CitationBorst et al., 2000). Several MDR mechanisms have been identified, including over-expression of the ATP-binding cassette (ABC) superfamily of transporters, which function as pumps to extrude a broad spectrum of chemotherapeutic drugs from cancer cells.
Among the ABC transporters frequently over-expressed in drug-resistant cancer cells are multidrug resistance protein 1 (MRP1) and P-glycoprotein (Pgp); the latter is encoded by the human MDR1 gene (CitationMohri, Nitta, & Yamashita, 2000; CitationSharom, 2008). Substrates for Pgp proteins are typically non-polar or hydrophobic, including alkaloids, vinblastine, colchicines, and anthracyclines. The role of Pgp in mediating MDR has been extensively studied, and development of small Pgp inhibitors has been widely pursued to increase drug sensitivity (CitationShukla, Wu, & Ambudkar, 2008). However, clinical use of such inhibitors is hampered by side-effects, inadequate specificity, and alteration of Pgp function in normal tissues.
As an alternative strategy to overcome drug resistance caused by Pgp, drug delivery systems bypassing Pgp have been developed (CitationJabr-Milane et al., 2008). Many such studies have used liposomes to deliver Pgp-substrate drugs (CitationMamot et al., 2003). Liposomal delivery systems in combination with Pgp inhibitors have been particularly effective to overcome MDR (CitationAdvani et al., 2001), and incorporation of Pgp-substrate drugs in liposomes has provided a way to circumvent Pgp-mediated MDR. Increased cellular accumulation and/or cytotoxicity of liposomal drugs have been observed in many studies with drug-resistant cancer cells showing high Pgp expression (CitationThierry, Dritschilo, & Rahman, 1992; CitationMichieli et al., 1999; CitationMamot et al., 2003). The mechanisms of this liposomal effect remain unclear, but involve the direct interaction of liposomes, particularly those with anionic lipids, and Pgp (CitationThierry et al., 1993). Certain phospholipids, the primary constituents of liposomes, have been implicated as Pgp substrates that compete with drugs for Pgp binding (CitationBosch et al., 1997). Immunoliposomes, which can be internalized by cells using receptor-mediated endocytosis, thereby bypass membrane-associated Pgp (CitationGoren et al., 2000). Pgp has also been reported to interact directly with cholesterol (CitationEckford & Sharom, 2008). The binding of Pgp-substrate drugs with Pgp reconstituted into liposomes was affected by the chain lengths of liposome phospholipids (CitationRomsicki & Sharom, 1999). Collectively, these findings suggest that the liposomal effect on Pgp-related MDR may be affected by factors such as lipid composition.
We reviewed reports investigating the effects of liposomal drug incorporation on Pgp-mediated drug efflux from Pgp-over-expressing cells, and found that most earlier studies used cell lines that had acquired MDR by long-term exposure to increasing drug concentrations. However, as exposure of cells to anti-cancer drugs may induce the expression of several MDR-related proteins, including drug efflux pumps other than Pgp, and because some anti-cancer drugs are also substrates for other drug efflux pumps, the observed differences in drug effluxes might have been caused by the activities of other pumps. This prompted us to investigate the liposomal effect on Pgp-related MDR in a cell line that had acquired high Pgp expression in a different manner. To this end, in the present study we investigated the impact of liposomal composition on efflux of Pgp-substrate from two breast cancer cell lines: wild-type cells with no detectable Pgp expression and Pgp-expressing cells resulting from stable transfection of the MDR1 gene. Here we show that inclusion of cholesterol and polyethyleneglycol (PEG)-attached lipids in liposomes is important to increase Pgp-substrate retention in Pgp-over-expressing cells.
Materials and methods
Cell lines and cultures
The human MCF-7/WT breast cancer cell lines and MCF-7/Pgp (originally named MCF-7/BC-19) cells, which acquired high Pgp expression by stable transfection with MDR1 cDNA, were kindly provided by Dr K. H. Cowan. (National Cancer Institute, Bethesda, MD) (CitationYu et al., 1991). Cancer cells were cultured in RPMI medium supplemented with 10% heat-inactivated FBS (Hyclone, Logan, UT) and 100 units/ml each of penicillin and streptomycin. Cells were grown in incubators in a humid atmosphere of 95% air/5% CO2.
Materials
Rhodamine 123 was purchased from Molecular Probes (Eugene, OR). Dimyristoylphosphatidylcholine (DMPC), dimyristoylphosphatidyl glycerol (DMPG), 1,2-Dioleoyl-3-Trimethylammonium-Propane (DOTAP), 2-Dipalmitoyl-sn-Glycero-3-Phosphoethanolamine-N-[Methoxy(Polyethyleneglycol)-350] (DPPE-PEG-350), and 1,2-Dipalmitoyl-sn-Glycero-3-Phosphoethanolamine-N-[Methoxy(Polyethyleneglycol)-5000] (DPPE-PEG-5000) were purchased from Avanti Polar lipids (Albaster, AL). Cholesterol (CHOL), dicetyl phosphate (DCP), and stearylamine (SA) were purchased from Sigma (St. Louis, MO). Antibodies to human Pgp were obtained from Calbiochem (La Jolla, CA). Antibodies to human β-actin were obtained from Santa Cruz Biotechology (Santa Cruz, CA). All other chemicals were of reagent grade and used without further purification.
RT-PCR analysis
Total cellular RNA was isolated from cells using a Qiagen RNeasy mini kit (Qiagen, Santa Clarita, CA). Single-stranded oligo(dT)-primed cDNA was generated from 2 μg RNA in a 25 μl reaction using Moloney murine leukemia virus reverse transcriptase (Promega, Madison, WI). Primers used for analysis of human MRP1, MDR1, and BCRP were: MRP1 (sense) 5’-CGTGTACTCCAACGCTGAC-3’ and MRP1 (antisense) 5’-CTGGACCGCTGACGCCCGTGAC-3’; MDR1 (sense) 5’-GGAAGCCAATGCCTATGACTTTA-3’ and MDR1 (antisense) 5’-GAACCACTGCTTCGCTTTCTG-3’; BCRP (sense) 5’-TGGCTGTCATGGCTTCAGTA-3’ and BCRP (antisense) 5’-GCCACGTGATTCTTCCACAA-3’. Primers for glyceraldehyde-3-phosphate dehydrogenase (GAPDH) were used as an internal standard (F: 5’-GTCAACGGATTTGGTCGTATT-3’, R: antisense primer 5’-AGTCTTCTGGGTG GCAG-TGAT-3’). The amplification reaction was carried out with 5 μl cDNA product for 27 cycles, and each cycle consisted of 94°C for 45 s, 57°C for 50 s, and 72°C for 50 s, followed by a final 1 min elongation at 72°C. The final PCR products were electrophoresed in 1% agarose gel.
Immunoblotting
Actively growing cells were scraped from the culture, washed twice with PBS, and incubated for 15–30 min on ice in lysis buffer containing 150 mM NaCl, 10 mM Tris, 0.2% Triton X-100, 0.3% NP-40, 0.2 mM Na3VO4, and protease inhibitors (pH 7.4) (Roche). After centrifugation at 16,000 g for 15 min at 4°C, supernatants were collected and the protein concentration in each was measured by the Bradford method. Aliquots of supernatants containing equal amounts of protein were boiled in SDS-reducing buffer for 5 min, electrophoresed on SDS-polyacrylamide gels, and transferred to nitrocellulose membranes. Membranes were blocked with 5% non-fat dry milk and probed with specific primary antibodies, followed by incubation with appropriate peroxidase-conjugated secondary antibodies. Blots were developed with ECL Plus reagent (Amercham, Arlington Heights, IL) according to the manufacturer’s protocol.
Preparation of liposomes
Fourteen micromoles of lipid mixtures composed of DMPC and cholesterol and 1 mg of rhodamine 123 (dissolved in methanol at 2 mg/ml) were dissolved in chloroform. The organic solvents were removed on a rotary evaporator under reduced pressure. The dried thin film on the inner surface of the flask was hydrated in 100 mM phosphate buffered saline (PBS, pH 7.4). After 30 min incubation at room temperature, the suspension of multilamellar liposomes was sonicated in a bath-type sonicator at 37°C for 1 h. Liposomes were loaded onto Sephadex G-50 column to remove unloaded rhodamine, and stored at 4°C until use. The concentration of rhodamine loaded in liposomes was assayed by a spectrofluorimteter after lysing liposomes with 1% Triton X-100. A known concentration of free rhodamine was used as a standard.
Size determination of liposomes
The mean particle diameter and polydispersity index (P.I.) of rhodamine-loaded liposomes were determined by a dynamic light scattering method using a fiber-optics particle analyzer (FPAR-1000, Otsuka Electronics Co. Ltd., Japan). The particle diameter analysis data were evaluated using volume distribution to detect even a few large particles. The P.I. is a measure of the distribution of nanoparticle population.
Rhodamine efflux assay
To assess the Pgp functional activity, the retention of rhodamine 123 was examined. Rhodamine 123 has been reliably used as a probe for Pgp functional activity (CitationElamanchili, McEachern, & Burt, 2008; CitationThews et al., 2008). Cells were seeded in 24-well culture plates in 1 mL of medium per well. At 24-h post-incubation, cells were washed with PBS and 1 ml of fresh medium containing free rhodamine or liposomal rhodamine was added to each well. Cells were then incubated for indicated periods of time. At the end of the incubation period, cells were washed twice in PBS, and further incubated with fresh media free of rhodamine. At indicated times, cells were washed three times with PBS and finally lysed in 0.1% Triton X-100/PBS (200 μl/well). The intracellular concentration of rhodamine was determined by measuring fluorescence of the cell lysates with a fluorescence microplate reader (Molecular Devices, Gemini EM). The excitation and emission wavelengths were 485 and 535 nm. Extracts from cells incubated with media free of rhodamine were used as blank. The intracellular concentration of rhodamine was determined from a standard curve and normalized to cellular numbers per well
Release study
The release test was performed using vertical diffusion cells, with the upper and lower chambers separated using a dialysis membrane (molecular cut-off of 10,000 Da) (CitationLee, Lim, & Kim, 2007). The lower receiver chamber was filled with 0.1 M PBS (4% in PBS, pH 7.4), which was mixed evenly by stirring with a star-shaped magnetic bar at 300 rpm. The joint between upper and lower chambers was sealed with Teflon tape and clamped tightly to avoid fluid loss. The temperature was controlled using a water circulator. Liposomes in culture media supplemented with 10% fetal calf serum were added to the upper chamber, which was then covered with laboratory film. At the indicated times, 0.1 mL of solution was removed from the lower receiver chamber and subjected to spectrofluorometric analysis of rhodamine. After solution removal, the same volume of fresh solution was added to maintain the total volume in the receiver chamber.
Statistical analysis
Statistically significant differences between values obtained under different experimental conditions were determined using two-tailed unpaired Student’s t-tests.
Results and discussion
Expression of drug efflux pumps
To compare drug efflux pump expression in MCF-7/WT and MCF-7/Pgp cells, we first measured mRNA levels from genes encoding Pgp, MRP1, and breast cancer resistance protein (BCRP), all of which are drug efflux pumps frequently expressed in breast cancer patients (CitationColey, 2008). Semiquantitative RT-PCR analysis revealed that Pgp mRNA was detectable in MCF-7/Pgp cells, but not in MCF-7/WT cells. In contrast, the mRNA levels encoding MRP1 and BCRP were similar in the two cell lines (). The apparent difference in Pgp expression was also observed at the protein level (). Our data suggest that MDR1 cDNA transfection into MCF-7/WT cells resulted in stable Pgp expression without affecting expression of other drug efflux pumps.
Figure 1. Expression of p-glycoprotein in MCF-7/WT and MCF-7/Pgp cells. (A) Expression of Pgp, MRP1 and BCRP mRNA in MCF-7 cells was determined by semi-quantitative RT-PCR, with GAPDH mRNA serving as a loading control. (B) Immunoblot analysis of Pgp protein expression in cells. Immunoblotting with an antibody to β-actin was used to ensure equal loading of proteins in each lane.
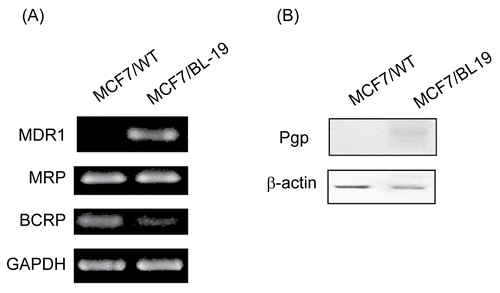
Uptake and retention of rhodamine
Cellular uptake and retention of rhodamine were studied by monitoring time-dependent changes in the intracellular concentrations of rhodamine. We first determined the time course of rhodamine uptake by MCF-7/WT cells. During 8 h of incubation, rhodamine cellular uptake increased in the first 4 h and then remained rather constant up to 8 h (). Rhodamine uptake increased with rises in initial rhodamine concentration (). In MCF-7/Pgp cells, the time course and dose-dependency of rhodamine uptake were similar (data not shown), suggesting that rhodamine uptake was not altered by Pgp expression in MCF-7 cells.
Figure 2. Rhodamine uptake and retention in MCF-7/WT and MCF-7/Pgp cells. (A) Time course of rhodamine uptake into MCF-7/WT cells. Cells were exposed to 90 ng/ml rhodamine for the time period indicated. (B) Dose-dependent uptake of rhodamine into MCF-7/WT cells. Cells were exposed to indicating concentrations of rhodamine for 4 h. (C) Rhodamine retention in MCF-7/WT and MCF-7/Pgp cells. Cells pre-incubated with 90 ng/ml rhodamine for 4 h were washed three times with PBS and further incubated in medium free of rhodamine for the indicated times. Cellular extracts were obtained and subjected to spectrofluorimetric analysis as described in the text. Data are presented as means ± SD (n = 3) and analyzed by Student’s t-test. Statistical comparisons between MCF-7/WT and MCF-7/Pgp cells are presented: * p < 0.05, ** p < 0.005.
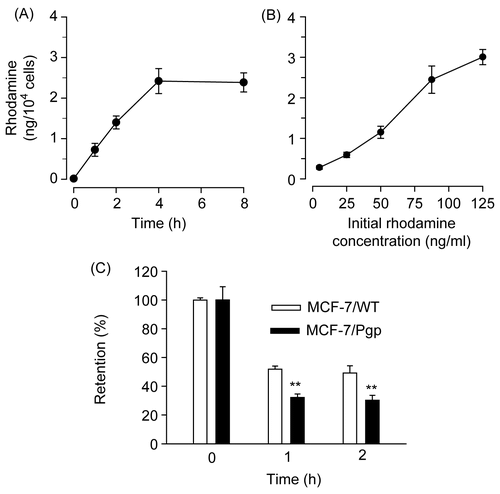
As Pgp is known to act as a drug efflux pump, we next investigated rhodamine retention in MCF-7/WT and MCF-7/Pgp cells. When MCF-7 cells incubated with rhodamine for 4 h were washed and incubated in medium free of rhodamine for additional periods of 1 h and 2 h, the intracellular rhodamine concentration decreased in a time-dependent manner regardless of Pgp expression. However, the decrease was much faster in MCF-7/Pgp cells compared with MCF-7/WT cells; the levels of rhodamine released were 1.42-fold and 1.37-fold greater from MCF-7/Pgp cells at 1 h and 2 h post-incubation, compared with MCF-7/WT cells (). Our data indicate that Pgp expression increased rhodamine efflux, suggesting that Pgp in MCF-7/Pgp cells is functional.
Effect of liposome incorporation on rhodamine efflux
To investigate whether use of liposomes as rhodamine carriers altered rhodamine efflux, we loaded rhodamine into liposomes composed of a 7:3 molar ratio of DMPC and CHOL. One- and 2-h retention levels of rhodamine in MCF-7/WT cells did not differ significantly from values seen when cells were incubated with free rhodamine (p > 0.05). In contrast, loading of rhodamine into liposomes increased the 1 h and 2 h retention levels in MCF-7/Pgp cells by 1.6-fold and 1.5-fold (, p < 0.05 and p < 0.005, respectively). Thus, liposome loading increased rhodamine retention in cells showing high Pgp expression, but not in cells without Pgp. After rhodamine incubation, no significant change in Pgp expression was observed, suggesting that the liposomal effect was not caused by alteration of Pgp expression (data not shown).
Figure 3. Retention of rhodamine in MCF-7/WT and MCF-7/Pgp cells following incubation with rhodamine in free forms or liposome-loaded forms. Rhodamine was loaded in liposomes prepared with 7:3 molar ratios of DMPC and CHOL. Cells pre-incubated with 90 ng/ml of free or liposomal rhodamine for 4 h were washed three times with PBS and further incubated in medium free of rhodamine for the indicated times. Cellular extracts were obtained and subjected to spectrofluorimetric analysis as described in the text. Data are presented as means ± SD (n = 3) and analyzed by Student’s t-test. Statistical comparisons between MCF-7/WT and MCF-7/Pgp cells are presented: * p < 0.05, ** p < 0.005.
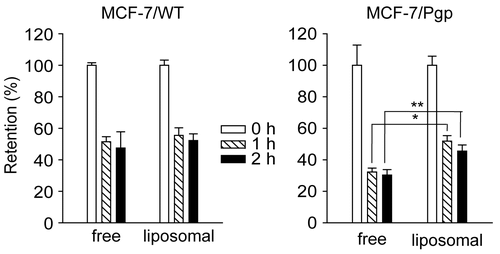
Effect of liposome composition on rhodamine efflux
Liposome composition is known to be important in determining the physicochemical characteristics of liposomes, affecting the in vitro and in vivo release, distribution, and metabolism of incorporated drugs (CitationSlepushkin et al., 1997). We were therefore interested to determine whether rhodamine retention in MCF-7/Pgp cells could be further increased by modulating liposome composition.
To examine the impact of CHOL liposomal content on rhodamine efflux, we prepared liposomes containing 0%, 20%, 40%, or 55 molar % CHOL. Inclusion of CHOL in liposomes, up to 40% of total lipids, increased rhodamine retention in MCF-7/Pgp cells. When retention was determined after incubation with liposomes containing 0%, 20%, or 40% CHOL, the percentages of retained rhodamine were 44.9%, 61.1%, and 68.0%, respectively, after 0.5 h, and 41.0%, 45.6%, and 63.7%, respectively, after 1 h (). However, further increases in CHOL content, up to 55%, decreased rhodamine retention. Therefore, maximal retention could be achieved by incorporating rhodamine in liposomes containing 40% CHOL.
Figure 4. Effect of liposomal composition on the retention of rhodamine loaded in liposomes. (A) Rhodamine was loaded in liposomes prepared with 10:0, 8:2, 6:4, or 4.5:5.5 molar ratios of DMPC:CHOL. (B) Liposomes were prepared with 14 μmole of lipid mixtures composed of DMPC:CHOL (6:4), DMPC:CHOL:DCP (6:4:1), DMPC:CHOL:DMPG (6:4:1), DMPC:CHOL:SA (6:4:1), or DMPC:CHOL:DOTAP (6:4:1). (C) liposomes were prepared with 14 μmole of lipid mixtures composed of DMPC:CHOL (6:4), DMPC:CHOL:DPPE-PEG350 (6:4:1), or DMPC:CHOL:DPPE-PEG5000 (6:4:1). Cells pre-incubated with 90 ng/ml of liposomal rhodamine for 4 h were washed, and further incubated in medium free of rhodamine for the indicated times. Cellular extracts were obtained and subjected to spectrofluorimetric analysis as described in the text. Data are presented as means ± SD (n = 3).
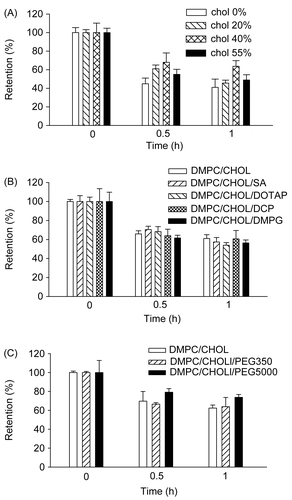
As most membrane proteins exist as charged forms under physiological conditions, we hypothesized that liposome surface charge might affect interactions with Pgp, thereby affecting Pgp-mediated drug efflux. Thus, we examined whether liposome surface charge affected rhodamine efflux. Positively charged liposomes were made by including SA or DOTAP at a 6:4 molar ratio in the DMPC:CHOL lipid mixture, and negatively charged liposomes were prepared by including DCP or DMPG at a 6:4 molar ratio. As shown in , no significant differences in rhodamine efflux from MCF-Pgp cells were found regardless of liposome surface charge. These data suggest that liposome surface charge was not a major factor modulating rhodamine efflux under our experimental conditions.
Attachment of hydrophilic polymers such as PEG to liposome phospholipids often affects the release and distribution of incorporated drugs, presumably because of steric inhibition of liposomal membrane interaction with other proteins or destabilizing factors (CitationKono, Igawa, & Takagishi, 1997). Thus, we prepared liposomes without PEG, and liposomes attached to short-chain PEG (PEG350) or long-chain PEG (PEG5000). Attachment of PEG5000, but not PEG350, increased rhodamine retention by cells (); the percentages of retained rhodamine in MCF-7/Pgp cells were 62.4%, 63.9%, and 73.8% 1 h post-incubation with liposomes prepared with DMPC/CHOL, DMPC/CHOL/PEG350, or DMPC/CHOL/PEG5000, respectively. These data indicate that liposome attachment of a PEG with a long hydrophilic chain was particularly effective in increasing rhodamine efflux.
Release characteristics of liposomes
As our data demonstrated that liposome composition was important in control of rhodamine efflux, and that inclusion of CHOL (up to 40%, w/w) and PEG5000 in liposomal membranes increased rhodamine retention, we investigated whether the kinetics of liposomal rhodamine release were correlated with observed retention data.
As shown in , rhodamine release from liposomes incubated in media containing 10% (v/v) fetal calf serum was fastest when liposomes were composed of DMPC alone, followed by DMPC/CHOL liposomes, DMPC/COL/PEG350 liposomes, and DMPC/CHOL/PEG5000 liposomes. At 4 h post-incubation, the percentages of rhodamine retained in liposomes were 43.5%, 50.5%, 53.0%, and 62% of initial loads, respectively. As the mean liposome particle size and polydispersity index were similar regardless of liposome composition (), it seems unlikely that rhodamine release was affected by liposome size characteristics. Probably, inclusion of CHOL and PEG5000 in liposomal membranes increased the rigidity of liposomes in the presence of destabilizing serum factors, thereby inhibiting the rhodamine release. Taken together, our data demonstrate that the kinetics of liposomal rhodamine release were correlated with observed retention data.
Figure 5. Mean particle size and rhodamine release characteristics of liposomes. (A) Release profile of rhodamine from liposomes in culture media containing 10% FCS at 37°C. (B) Mean particle size (χ) and polydispersity index (PI) (•) of liposomes. Rhodamine-loaded liposomes were prepared with 14 μmole of lipid mixtures composed of DMPC alone, DMPC:CHOL (6:4), DMPC:CHOL:DPPE-PEG350 (6:4:1), or DMPC:CHOL:DPPE-PEG5000 (6:4:1). Data are presented as means ± SD (n = 3).
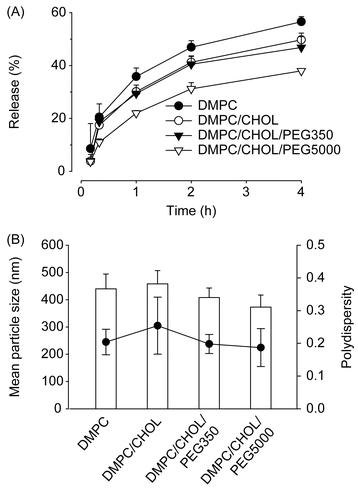
Conclusions
In this study, we compare the retention of rhodamine 123, a probe for Pgp-substrate drugs, in two cell lines showing similar expression of several drug efflux pumps, except Pgp. We show that liposome loading of rhodamine significantly increased rhodamine retention in cells showing high Pgp expression, but not in cells without Pgp expression. Further, inclusion of CHOL and PEG5000 in liposomal membranes permitted increased rhodamine retention in Pgp-expressing cells. In contrast, inclusion of charged lipids in liposomal membranes did not affect rhodamine retention. As the rate of rhodamine release from liposomes tended to be inversely correlated with rhodamine retention by cells, it is probable that more rigid liposomes are able to sequester rhodamine more efficiently, thereby inhibiting direct interactions of rhodamine with Pgp proteins. Our findings suggest that Pgp-mediated MDR in cancer cells may be more effectively modulated by optimizing the composition of liposomes, loaded with Pgp-substrate anti-cancer drugs.
Acknowledgement
This work was supported in part by Grant No. R01-2006-000-10672-0 from the Basic Research Program of the Korea Science and Engineering Foundation, and also in part by the Pioneer Research Program for Converging Technology of the Ministry of Education, Science and Technology, Republic of Korea (Grant No. M1071118001-08M1118-00110) (2008).
Declaration of interest: The authors report no conflicts of interest. The authors alone are responsible for the content and writing of the paper.
References
- Advani, R., Fisher, G.A., Lum, B.L., Hausdorff, J., Halsey, J., Litchman, M., Sikic, B.I. (2001). A phase I trial of doxorubicin, paclitaxel, and valspodar (PSC 833), a modulator of multidrug resistance. Clin Cancer Res. 7, 1221–9.
- Borst, P., Evers, R., Kool, M., Wijnholds, J. (2000). A family of drug transporters: the multidrug resistance-associated proteins. J Natl Cancer Inst. 92, 1295–302.
- Bosch, I., Dunussi-Joannopoulos, K., Wu, R.L., Furlong, S.T., Croop, J. (1997). Phosphatidylcholine and phosphatidylethanolamine behave as substrates of the human MDR1 P-glycoprotein. Biochemistry. 36, 5685–94.
- Coley, H.M. (2008). Mechanisms and strategies to overcome chemotherapy resistance in metastatic breast cancer. Cancer Treat Rev. 34, 378–90.
- Eckford, P.D., Sharom, F.J. (2008). Interaction of the P-glycoprotein multidrug efflux pump with cholesterol: effects on ATPase activity, drug binding and transport. Biochemistry. 47, 13686–98.
- Elamanchili, P., McEachern, C., Burt, H. (2008). Reversal of multidrug resistance by methoxypolyethylene glycol-block- polycaprolactone diblock copolymers through the inhibition of P-glycoprotein function. J Pharm Sci. 98, 945–58.
- Goren, D., Horowitz, A.T., Tzemach, D., Tarshish, M., Zalipsky, S., Gabizon, A. (2000). Nuclear delivery of doxorubicin via folate-targeted liposomes with bypass of multidrug-resistance efflux pump. Clin Cancer Res. 6, 1949–57.
- Jabr-Milane, L.S., van Vlerken, L.E., Yadav, S., Amiji, M.M. (2008). Multi-functional nanocarriers to overcome tumor drug resistance. Cancer Treat Rev. 34, 592–602.
- Kono, K., Igawa, T., Takagishi, T. (1997). Cytoplasmic delivery of calcein mediated by liposomes modified with a pH-sensitive poly(ethylene glycol) derivative. Biochim Biophys Acta. 1325, 143–54.
- Lee, M.K., Lim, S.J., Kim, C.K. (2007). Preparation, characterization and in vitro cytotoxicity of paclitaxel-loaded sterically stabilized solid lipid nanoparticles. Biomaterials. 28, 2137–46.
- Mamot, C., Drummond, D.C., Hong, K., Kirpotin, D.B., Park, J.W. (2003). Liposome-based approaches to overcome anticancer drug resistance. Drug Resist Updat. 6, 271–9.
- Michieli, M., Damiani, D., Ermacora, A., Masolini, P., Michelutti, A., Michelutti, T., Russo, D., Pea, F., Baccarani, M. (1999). Liposome-encapsulated daunorubicin for PGP-related multidrug resistance. Br J Haematol. 106, 92–99.
- Mohri, M., Nitta, H., Yamashita, J. (2000). Expression of multidrug resistance-associated protein (MRP) in human gliomas. J Neurooncol. 49, 105–15.
- Romsicki, Y., Sharom, F.J. (1999). The membrane lipid environment modulates drug interactions with the P-glycoprotein multidrug transporter. Biochemistry. 38, 6887–96.
- Sharom, F.J. (2008). ABC multidrug transporters: structure, function and role in chemoresistance. Pharmacogenomics. 9, 105–27.
- Shukla, S., Wu, C.P., Ambudkar, S.V. (2008). Development of inhibitors of ATP-binding cassette drug transporters: present status and challenges. Expert Opin Drug Metab Toxicol. 4, 205–23.
- Slepushkin, V.A., Simoes, S., Dazin, P., Newman, M.S., Guo, L.S., Pedroso de Lima, M.C., Duzgunes, N. (1997). Sterically stabilized pH-sensitive liposomes. Intracellular delivery of aqueous contents and prolonged circulation in vivo. J Biol Chem. 272, 2382–8.
- Thews, O., Gassner, B., Kelleher, D.K., Gekle, M. (2008). Activity of drug efflux transporters in tumor cells under hypoxic conditions. Adv Exp Med Biol. 614, 157–64.
- Thierry, A.R., Dritschilo, A., Rahman, A. (1992). Effect of liposomes on P-glycoprotein function in multidrug resistant cells. Biochem Biophys Res Commun. 187, 1098–105.
- Thierry, A.R., Vige, D., Coughlin, S.S., Belli, J.A., Dritschilo, A., Rahman, A. (1993). Modulation of doxorubicin resistance in multidrug- resistant cells by liposomes. FASEB J. 7, 572–9.
- Yu, G., Ahmad, S., Aquino, A., Fairchild, C.R., Trepel, J.B., Ohno, S., Suzuki, K., Tsuruo, T., Cowan, K.H., Glazer, R.I. (1991). Transfection with protein kinase C alpha confers increased multidrug resistance to MCF-7 cells expressing P-glycoprotein. Cancer Commun. 3, 181–9.