Abstract
The purpose of the present study is to develop a new method to prepare magnetite chitosan microspheres conjugated with methotrexate (MTX) for the controlled release of MTX as a magnetic targeting drug delivery system. MTX was first conjugated to the chitosan chain via a peptide bond and then a suspension cross-linking technique was used for the production of magnetic chitosan microspheres with glutaraldehyde as the cross-linker. The MTX-loading capacity of the magnetic chitosan microspheres was determined and drug release experiments were also carried out to discuss the MTX release behavior. All the data support that the magnetic chitosan-MTX microspheres prepared in this method would have great potential application in magnetic targeting drug delivery technology.
Introduction
During the past several years, targeting drug delivery systems have been extensively explored, especially for tumor diagnostics and therapeutics (CitationLaVan, McGuire, & Langer, 2003; CitationCaruthers & Wickline, 2007; CitationShay & Keith, 2008). The principle of the targeting drug delivery system consists of two steps: The first step is delivery of the desired amount of drug to the required compartment in an organism; the second is the release of drugs from its carrier at a controlled rate (CitationArias et al., 2001; CitationPrabaharan & Mano, 2004). Magnetic labeling of drugs could be easily transported and focused under the guidance of an external magnetic field, leading to a tissue-specific release of drugs with minimal side-effects. However, due to the limited functional groups of magnetite nanoparticles for selective binding drug molecules, the amount of drug carried and the rate released are rather limited, although their surfaces are decorated with hydroxyl groups that could render them intrinsically hydrophilic to decrease oxide particle clearance by the reticulo-endothelial system (RES), and thus increase their circulation time in the blood (CitationBarbé et al., 2004; CitationYang et al., 2006). In addition, the magnetic nanoparticles must remain non-aggregated and stable against oxidation because the surface of the nanoparticles can influence its behavior in a biological environment. Therefore, a suitable coating is necessary to improve these situations. Chitosan, the second most abundant natural polymer next to cellulose (CitationXue et al., 2006), is a polyaminosaccharide with many significant biological (i.e. biodegradable, biocompatible, bioactive) and chemical properties (polycationic, hydrogel, contains reactive groups such as OH and NH2) (CitationSinha et al., 2004; CitationDesai & Park, 2006; CitationZhou et al., 2006), including some unique anti-tumor and antibacterial bioactivities (CitationZhao et al., 2005). Hence, in this work, chitosan is employed as a base material for magnetic targeting drug delivery.
Methotrexate is a widely used anti-cancer agent, but its cytotoxicity to normal tissue and inherent multi-drug resistance effect are major problems to be solved (CitationWang et al., 2006; CitationLowenthal, Horn, & Kalb, 2008). In this work, MTX molecules were covalently bonded to the surface of magnetite chitosan microspheres, via a peptide bond, to be further investigated as magnetic targeting drug delivery. Due to the difficulties in quantifying the amount of MTX that reacted with the amine groups on the surface of the magnetite chitosan microspheres, the preparation method is different from those in the literatures (CitationJiang et al., 2005; CitationCekié et al., 2007; CitationZhang et al., 2007) and the overall schematic is presented in : MTX is first conjugated to the base material through amidation between the carboxyl acid groups of MTX and the amino groups of the chitosan chain in the present study. The suspension cross-linking technique is then used for the production of magnetic chitosan microspheres and glutaraldehyde is used as a cross-linker. It is expected that the preparation route revealed here would shed new light on the preparation of other magnetic targeting drug delivery systems. At the same time, results of the controlled release experiments show that by covalently modifying the surface of magnetite chitosan microspheres via peptide bond, MTX is not released from the surface of microspheres under intravenous conditions, and the cleavage of the amide bond mostly occurs under conditions of low pH and in the presence of lysozyme (CitationKohler et al., 2005), a typical environment inside the tumor cells, exhibiting the targeting effect.
Materials and methods
Materials
Chitosan with Mw 5 × 105 and 90% degree of deacetylation was purchased from Yuhuan Ocean Biochemistry Co Ltd. (Zhejiang, China). Methotrexate hydrochlorate (MTX. HCl) was purchased from Haizheng Co. (Zhejiang, China). Protease type I: crude protease from bovine pancreas and 1-ethyl-3-(3-(dimethylamino) propyl) carbodiimide (EDC) were obtained from Sigma (Missouri, USA). N-Hydroxysuccinimide (NHS, 98%) was obtained from Aldrich Chemical Co. (USA) Mineral oil, Tween-20, Span-80, and glutaraldehyde were supplied by Shanghai Chemical Reagent Co. (Shanghai, China). Other chemicals were purchased from Beijing Chemical Co. (Beijing, China) All chemicals are of analytical grade reagents and used directly without further purification.
Characterization
Characterization of the products were performed by X-ray diffraction (XRD, Rigaku-D/max 2500 V with Cu KR radiation (λ = 1.5418 å)), Fourier transform infrared spectrometer (FTIR, BIO-RAD FTS135), TGA (Perkin–Elmer, SDT-2960), UV-Vis Spectrometer (Cary 300), and Scanning electron microscopy (SEM, XL30 ESEM FEG). The magnetic property was measured on a VSM Controller Model 7300 vibrating sample magnetometer at room temperature.
Grafting MTX to the chitosan chain
Five hundred milligrams of chitosan was dissolved in 60 ml 1% aqueous acetic acid; 500 mg MTX.HCl, 500 mg EDC·HCl and 57 mg NHS were then added into the chitosan solution. The reaction mixture was stirred for 2 days at room temperature after the pH of the solution was adjusted to 7–8 by addition of 0.5 M NaOH. The resultant yellow precipitate was collected by filtration. Purification of the conjugation from the unreacted free MTX was done by dialysis in NaHCO3 solution (pH = 8.35) with a cellulose membrane (MWCO = 8000 Da) for 3 days and subsequently in distilled water for another 3 days. The conjugated powder was finally obtained by lyophilization.
Synthesis of the magnetite nanoparticles
Magnetite nanoparticles were synthesized by a coprecipitation method. An aqueous solution containing 2 mM Fe3+ and 1 mM Fe2+ in a 250 ml flask was heated to 80°C with the deaeration of O2 by bubbling N2. Then 7 ml NH3.H2O was added under vigorous stirring. After 2 h, the precipitate was isolated by Nd-Fe-B magnet and washed twice with deionized water and three times with ethanol. Then the collected magnetic nanoparticles were dried at 60°C in vacuum. To gain the well dispersed Fe3O4 nanoparticles, the prepared magnetic nanoparticles were added to 0.1 M citric acid with ultrasonic treatment for 30 min and then the reaction was sustained for 12 h at room temperature. The obtained citric acid modified magnetic nanoparticles were isolated with Nd-Fe-B magnet and dried at 60°C under vacuum.
Preparation of magnetite chitosan microspheres conjugated with methotrexate (magnetic chitosan-MTX microspheres)
The suspension cross-linking technique was used here for the production of magnetic chitosan microspheres. In a typical procedure, 50 mg of the obtained magnetic nanoparticles was dispersed in 5 ml deionized water and was treated by ultrasonic wave for 30 min; 100 mg chitosan-MTX conjugate was dissolved in 5 ml 5% aqueous acetic acid and then mixed with the magnetic dispersion with agitation. The mixture solution (water phase) was poured, dropwise, into 200 ml of paraffin oil containing 6.4 g of Span-80, 1.2 g Tween-20 (oil phase) in a 400 ml beaker at room temperature. Then, the suspension was emulsified by a homogenizer operating at 30 KW for another 30 min after being stirred for 30 min. A certain amount of 50% glutaraldehyde solution was consecutively and slowly added into the suspension and the mixture was stirred for 30 min at 40°C. Subsequently, drops of 0.5 M NaOH solution were gradually added until the pH of the mixture reached 9–10 and further stirred for 2 h at 70°C. Finally, the product mixture was centrifuged and washed consecutively with ethyl oxide, ethanol, and distilled water. The obtained microspheres were dried overnight at 60°C in an air forced oven.
Evaluation of MTX-loading capacity of the magnetic chitosan microspheres
The MTX-loading capacity (LC) of the magnetic chitosan microspheres was calculated using the following equation:
where α is the mass percentage of cross-linked chitosan-MTX conjugation occupied in total magnetic chitosan microspheres, β is the amount of MTX in 1 mg cross-linked chitosan–MTX conjugation. It is not easy to determine the value of β directly, but it can be considered equivalent to the MTX loading in 1 mg cross-linked chitosan–MTX microspheres, which are prepared by the similar procedure to prepare magnetic chitosan–MTX microspheres described above with the same reaction conditions, except for the absence of magnetic nanoparticles. Five milligrams of such chitosan–MTX microspheres were suspended in 10 ml of phosphate-buffered saline (PBS) solution at 37°C under constant stirring. The solution pH was adjusted to 2~3 by the titration of 1.0 M HCl. After incubation for 7 days, the suspension was centrifuged at 1000 rpm every 12 h to isolate the microspheres from the cleaved MTX and PBS and the absorptance of supernatant at a wavelength of 262 nm was measured with a UV-Vis spectrometer. Until the absorbance value at 262 nm was constant, free MTX in supernatant was thought to be the total methotrexate weight loaded in chitosan–MTX microspheres. The UV absorbance of MTX was converted to its concentration according to the calibration curve of MTX (Conc (μg/ml) = 20.2224 ABS-0.0283, r = 0.9983) in the same buffer. Then the amount of total methotrexate loaded in chitosan–MTX microspheres could be calculated.
Analysis of MTX release behavior
Magnetite chitosan-MTX microspheres prepared here are expected to release drugs in tumor cells and decrease potential side-effects to healthy tissues. The lower pH value of cancer cells is a marked difference from normal cells. Thus, it is necessary to carry out controlled release experiments at various pH values. To vividly simulate intracellular lysosomal conditions, controlled experiments are all performed in the presence of protease type I, a kind of hydrolase with catalysis to the cleavage of amide bond. The concrete process is as follows: magnetic chitosan–MTX microspheres at a concentration of 0.1 mg/ml were suspended in a solution of 0.1 mg/ml crude protease from bovine pancreas in 5 ml of phosphate-buttered saline (PBS) solution at 37°C under constant stirring. The solution pH was adjusted by the titration of 1.0 M HCl and 1.0 M NaOH to achieve pH values of 2, 5, and 7.44, respectively. After incubation for a predetermined time, the suspensions were centrifuged at 1000 rpm to isolate the microsphere from the cleaved MTX, PBS, and protease solutions. Cleaved MTX was quantified with a UV-Vis spectrometer at wavelength of 262 nm. To examine the possible influences of the suspension cross-linking process and the presence of magnetite nanoparticles on the release behavior of MTX, release of MTX from chitosan-MTX conjugation was also carried out at pH = 7.44 by placing the sample inside a dialysis membrane under shaking at the same incubation conditions and monitored with a UV-Vis spectrometer at a fixed wavelength of 262 nm.
Results and discussion
SEM image
shows the typical SEM photographs of magnetic chitosan–MTX microspheres prepared by a suspension cross-linking technique. The microspheres exhibit a smooth surface morphology. This may be due to the higher cross-linking density of the magnetic microspheres. The average particle size is ~ 6 μm and thus macrospheres would be easily retained by pulmonary capillaries, favoring the treatment of pulmonary carcinoma.
FTIR analysis
To directly confirm the successful preparation of magnetite chitosan microspheres and the evidence of MTX on the microspheres surface, FTIR studies are carried out. shows the FTIR spetrum of chitosan, MTX.HCl, chitosan–MTX, and the magnetite chitosan microspheres conjugated with methotrexate. The assignments of the characteristic FTIR absorption bands presented in are summarized in . For the IR spectrum of pure chitosan (), characteristic peaks have amide band I at 1654 cm−1, amide band II at 1603 cm−1, amide band III at 1323 cm−1, and 3348 cm−1 of ν (NH2). In the IR spectrum of MTX (), the characteristic stretching vibration of the aromatic ring skeleton appears at 1607 cm−1. The other two strong peaks observed at 1564 cm−1 and 1410 cm−1 are the asymmetrical and symmetrical stretching of COO− groups. The characteristic absorption bands of FTIR spectra of chitosan–MTX () include ν (N-H···H, O-H···H) ( 3423 cm−1), (C-H) ( 2920, 2876 cm−1), ν (aromatic ring skeleton of methotrexate molecule) ( 1607 cm−1), ν (COO−as, COO−s) (1562, 1407 cm−1), δ (N-H) ( 1508 cm−1), δ (CH2, CH3) (1450, 1378 cm−1), (C-O) ( 1322 cm−1), ν (C-N) ( 1208 cm−1), ν (C3-OH, C6-OH) (1123, 1020 cm−1) and ν (β-glycosidic bond) ( 894 cm−1). It should be noted that the intensity of the amide I in became stronger than that of pure chitosan presented in , and that the characteristic stretching vibration band of the aromatic ring skeleton of methotrexate molecule appeared at 1607 cm−1 also became broader than that shown in due to the coupling action with amide band I. These observations imply successful grafting of chitosan chain with MTX using an acylation reaction via a peptide covalent bond.
Figure 3. FTIR spectra of chitosan (a), MTX (b), Chitosan–MTX (c), and magnetite chitosan–MTX microspheres (d).
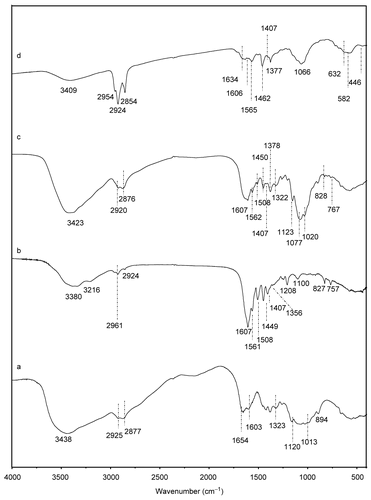
Table 1. Assignments of the characteristic FTIR absorption bands presented in .
Compared with the FTIR spectra of chitosan-MTX () and the magnetite chitosan-MTX microspheres (), their spectra are almost the same with the exception of four new bands appeared at 1634, 632, 582, and 446 cm−1. The peak at 1634 cm−1 observed in corresponds to the stretching vibration of the C=N bond. This strong peak indicates the formation of schiff’s base as the result of the reaction between the carbonyl group of glutaraldehyde and residual amine group of chitosan–MTX conjugation. The other three new bands in relate to Fe-O bonds in Fe3O4 nanoparticles, indicating the formation of magnetite chitosan microspheres.
XRD analysis
shows the XRD patterns of the prepared magnetite nanoparticles () and the magnetite chitosan-MTX microspheres (). All diffraction peaks of the XRD pattern of the prepared magnetite nanoparticles presented in can be easily indexed to be a pure cubic inverse spinel structure, which matches well with the reported value (JCPDS: 65-3107), indicating the formation of Fe3O4 phase. The average particle size calculated using the Debye-Scherrer formula from the reflection peak of (311) is ~ 10 nm. For the magnetite chitosan microspheres conjugated with methotrexate, the intensities of the corresponding diffraction peaks of magnetite nanoparticles are slightly reduced due to the presence of chitosan. It is also illustrated from the XRD data that the chitosan shell coating on the surface of the magnetic nanoparticles is amorphous, as evident from the absence of the diffraction peak at ~ 2θ = 10° and the presence of a broader hump at 2θ = 18.8°. This is an important property for the magnetic carrier technology because the amorphous chitosan are more accessible to water and more vulnerable to degradation due to the reason that chemical degradation of the shell material including a number of stages always begins with water absorption from the environment (CitationSödergård & Stolt, 2002).
Drug-loading capacity
The amount of MTX conjugated with magnetite chitosan microspheres is determined by the combined technology of the TGA method and UV-Vis spectrometry. The former is used to determine the value of α, while the later is for β. shows the TGA curves of the prepared magnetite nanoparticles () and the magnetite chitosan–MTX microspheres (). The magnetite nanoparticles display little weight-loss, while the microsphere show appreciable weight loss. The former is attributed to the decomposition of the modified citric acid while the later is the decomposition of both modified citric acid and the cross-linked chitosan-MTX shell materials. Thus, the margin of the weight loss percentage between the two TGA curves during heating may be taken as a measure of the rate of the cross-linked chitosan-MTX. The weight loss of the magnetite nanoparticles and the magnetite chitosan microspheres conjugated with methotrexate are 6.17% and 84.10%, respectively. The mass percentage of surface-grafted chitosan-MTX (α) is calculated as 77.93%.
Figure 5. TGA curves of the prepared magnetite nanoparticles (a) and magnetite chitosan–MTX microspheres (b).
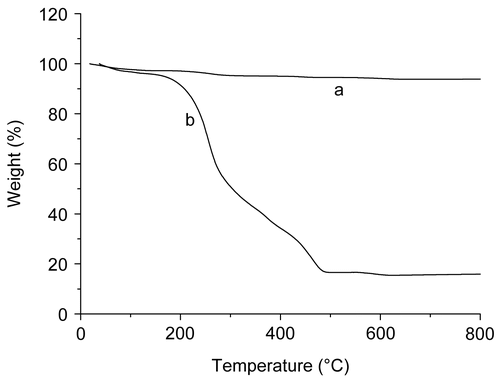
As mentioned above, β can be considered equivalent to the total amount of MTX released from 1 mg cross-linked chitosan–MTX microspheres and the result calculated with the UV-Vis absorbance data was 232 μg. So, according to equation (1), LC of the magnetic chitosan microspheres was 18.1%.
Drug release
The released MTX is monitored by determining the UV absorbance of MTX at 262 nm as a function of incubation time, and gives the MTX release profiles of the prepared magnetic chitosan–MTX microspheres incubated with protease solution at different pH values. Obviously, the drug release behavior is closely related to pH value. As shown in , the amount of released MTX increases with decreasing pH. This is because the drug is covalently grafted (via an amide bond) to the surface of magnetic chitosan microspheres. Its release is controlled by its dissociation from the microspheres rather than by simple diffusion. The cleavage of the amide bond depends on the pH value and the concentration of the presence of active protease. Specifically speaking, the lower the pH value in medium is, the faster the drug release from the microspheres. This pH sensitivity of the release rate of drug from the microspheres can probably be attributed to cleavage sensitivity of the amide bonds to pH value and the effect of pH value on the activity of the protease. Because the released amount of MTX at pH 2 is nearly twice that at pH 7.4, is most likely to illustrate that the concentration of active protease at pH 2 is the greatest. Though the concentration of active protease is higher at low pH, the activity of protease is decreased with extended time and the velocity that protease cleaves the peptide bond is significantly slowed, thus most of the conjugated methotrexate is released within the first 12 h at low pH.
Figure 6. Release profiles of methotrexate from magnetic chitosan–MTX microspheres in the presence of 0.1 mg/ml crude protease from bovine pancreas with various pH values at 37°C.
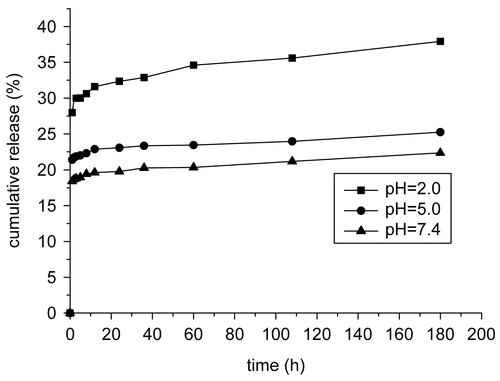
The result that no more than 30 cumulative % of the MTX released even at pH = 2 is an expectation from the point of application. Because rapid dissociation of drug from microspheres may result in its premature release in the blood stream, and hence the efficacy of the carrier system to target drug to the desired tissue/organ, and the sustained period of time could be dramatically reduced. Drug-loaded microspheres are expected to act as an intracellular depot with sustaining drug retention.
shows the release profiles of MTX from magnetic chitosan–MTX microspheres and chitosan–MTX conjugations incubated with protease solution at pH = 7.4, respectively. There is no appreciable difference between both, except that the amount of MTX released from the chitosan–MTX conjugations is slightly more than that from the magnetic microspheres with the same incubation time. Therefore, it is concluded that neither the cross-linking process nor the introduced magnetic nanoparticles has any significant effect on the release behavior of MTX.
Magnetism test
The magnetization curve for magnetic chitosan–MTX microspheres we prepared is shown in (dash dot). Room temperature specific magnetization (M) vs applied magnetic field (H) curve measurements of the sample indicates a saturation magnetization value (Ms) of 11.3 emu/g, much lower than that of bare magnetic nanoparticles (70.8 emu/g, solid line in ) due to the coated shell, but Ms can be improved by changing the fraction of magnetite nanoparticles in microspheres, i.e. increasing the Fe3O4/chitosan–MTX ration in the cross-linking process since the magnetic quality increases significantly with the Fe3O4 content (CitationDenkbaş et al., 2002), and the amount of mangetic nanoparticles does not influence the release behavior of MTX. In , we can also see that the two magnetization curves both follow a Langevin behavior over the applied magnetic field and the coercivity (HC) could be ignored, which can be considered as superparamagnetism. From the point of view of the magnetic targeting drug-delivery technology, the superparamagnetic property is the other special important parameter besides the high Ms. With the superparamagnetic property, capillary blockage by aggregations formed by residue magnetism after removal of the applied field will be avoided (CitationHu, Neoh, & Kang, 2006).
Conclusions
In conclusion, we developed a new method to prepare magnetite chitosan microspheres conjugated with methotrexate (MTX) for the controlled release of MTX as a magnetic targeting drug delivery system. The successful reaction of each stage was confirmed by FTIR. SEM images indicated the average diameter of the microspheres was 6 μm. The MTX-loading capacity determined by the combined technology of the TGA method and UV-Vis spectrometry was ~ 18.1%. Drug release experiments of the microspheres showed the same release behavior to that of chitosan-MTX conjugations, and displayed a pH-dependent behavior that MTX was cleaved from the microspheres easily under a low pH condition in the presence of protease and that most of the conjugated methotrexate were released within the first 12 h. With adjustable Ms value (CitationDenkbaş et al., 2002) and superparamagnetic property, magnetic chitosan-MTX microspheres prepared according to the method proposed in this work indicated a great potential application in the treatment of cancer using magnetic targeting drug delivery technology.
Acknowledgments
Declaration of interest: The authors report no conflicts of interest. The authors alone are responsible for the content and writing of the paper.
References
- Arias, J.L., Gallardo, V., Gómez-Lopera, S.A., Plaza, R.C., Delgado, A.V. (2001). Synthesis and characterization of poly (ethyl-2- cyanoacrylate) nanoparticles with a magnetic core. J Contr Rel. 77, 309–21.
- Barbé, C., Bartlett, J., Kong, L., Finnie, K., Lin, H.Q., Larkin, M., Calleja, S., Bush, A., Calleja, G. (2004). Silica particles: a novel drug-delivery system. Adv Mater. 16, 1959–66.
- Caruthers, S.D., Wickline, S.A. (2007). Lanza GM. Nanotechnological applications in medicine. Curr Opin Biotech. 18, 26–30.
- Cekié, N.D., Savi, S.D., Mili, J., Savi, M.M., Javi, ž., Maleševi, M. (2007). Preparation and characterisation of Phenytoin-loaded alginate and alginate-chitosan microparticles. Drug Deliv. 14, 483–90.
- Denkbaş, E.B., Kiliçay, E., Birlikseven, C., öztürk, E. (2002). Magnetic chitosan microspheres: preparation and characterization. React Funct Polym. 50, 225–32.
- Desai, K.G., Park, H.J. (2006). Preparation, characterization and protein loading of hexanoyl-modified chitosan nanoparticles. Drug Deliv. 13, 375–81.
- Hu, F.X., Neoh, K.G., Kang, E.T. (2006). Synthesis and in vitro anti-cancer evaluation of tamoxifen-loaded magnetite/PLLA composite nanoparticles. Biomaterials. 27, 5725–33.
- Jiang, D.S., Long, S.Y., Huang, J., Xiao, H.Y., Zhou, J.Y. (2005). Immobilization of Pycnoporus sanguineus laccase on magnetic chitosan microspheres. Biochem Eng J. 25, 15–23.
- Kohler, N., Sun, C., Wang, J., Zhang, M.Q. (2005). Methotrexate-modified superparamagnetic nanoparticles and their intracellular uptake into human cancer cells. Langmuir. 21, 8858–64.
- LaVan, D.A., McGuire, T., Langer, R. (2003). Small-scale systems for in vivo drug delivery. Nat Biotechnol. 21, 1184–91.
- Lowenthal, K.E., Horn, P.J., Kalb, R.E. (2008). Concurrent use of methotrexate and acitretin revisited. J Dermatol. 19, 22–6.
- Prabaharan, M., Mano, J.F. (2004). Chitosan-based particles as controlled drug delivery systems. Drug Deliv. 12, 41–57.
- Shay, J.W., Keith, W.N. (2008). Targeting telomerase for cancer therapeutics. Br J Cancer. 98, 677–83.
- Sinha, V.R., Singla, A.K., Wadhawan, S., Kaushik, R., Kumria, R., Bansal, K., Dhawan, S. (2004). Chitosan microspheres as a potential carrier for drugs. Int J Pharm. 274, 1–33.
- Södergård, A., Stolt, M. (2002). Properties of lactic acid based polymers and their correlation with composition. Prog Polym Sci. 27, 1123–63.
- Wang, L.Y., Gu, Y.H., Su, Z.G., Ma, G.H. (2006). Preparation and improvement of release behavior of chitosan microspheres containinginsulin. Int J Pharm. 311, 187–95.
- Xue, Z.X., Yang, G.P., Zhang, Z.P., He, B.L. (2006). Application of chitosan microspheres as carriers of LH-RH analogue TX46. React Funct Polym. 66, 893–901.
- Yang, J., Lee, H., Hyung, W., Park, S.B., Haan, S. (2006). Magnetic PECA nanoparticles as drug carriers for targeted delivery: Synthesis and release characteristics. J Microencapsulation. 23, 203–12.
- Zhang, J., Zhang, S.T., Wang, Y.P., Gao, S.F. (2007). Synthesis and characterization of composite magnetic microspheres of artemisia seed gum and chitosan. J Appl Polym Sci. 103, 3045–9.
- Zhao, A.J., Yao, P., Kang, C.S., Yuan, X.B., Chang, J., Pu, P.Y. (2005). Synthesis and characterization of tat-mediated O-CMC magnetic nanoparticles having anticancer function. J Magn Magn Mater. 295, 37–43.
- Zhou, H.Y., Chen, X.G., Liu, C.S., Hong, X., Liu, C.G., He, J., Yu, L.J. (2006). Cellulose acetate/chitosan multimicrospheres preparation and ranitidine hydrochloride release in vitro. Drug Deliv. 13, 261–7.