Abstract
The primary objective of the study was to develop a pH and transit time controlled sigmoidal release polymeric matrix for colon-specific delivery of indomethacin. Tablet matrices were prepared using a combination of hydrophilic polymers (polycarbophil or carbopol) having pH-sensitive swelling properties with hydrophobic polymer ethyl cellulose. The prepared matrices were characterized for physical properties and in vitro release kinetics. The presence of ethyl cellulose in a hydrophilic polymer matrix resulted in a sigmoidal in vitro drug release pattern with negligible-to-very low drug release in the initial phase (0–6 h) followed by controlled release for 14–16 h. The retardation in initial release can be attributed to the presence of ethyl cellulose that reduced swelling of hydrophilic polymer(s), while in the later portion, polymer relaxation at alkaline pH due to the ionization of acrylic acid units on carbopol and polycarbophil resulted in enhanced drug release. Thus, a sigmoidal release pattern was obtained that could be ideal for colonic delivery of indomethacin in the potential treatment of colon cancer.
Introduction
Colon-specific drug delivery provides an opportunity for the treatment of local diseases of the colon like ulcerative colitis, Crohn’s disease, and colorectal cancer. Various approaches used for targeting drugs to colon either include development of prodrugs that are selectively cleaved into active form in the colon, or solid dosage forms that release the drug in response to various physiological/bacterial/enzymatic conditions existing in the colon (CitationRubinstein, 1995; CitationKinget et al., 1998; CitationBasit, 2005).
Indomethacin, a non-steroidal anti-inflammatory drug, has been implicated for its chemotherapeutic potential in the treatment of colorectal cancer (CitationHull, Gardner, & Hawcroft, 2003). It is reported to cause growth inhibition, induction of apoptosis, and reduction in proliferation rates of HT-29 colon cancer cells (CitationKapitanović et al., 2006). It has been established recently that indomethacin down-regulates survivin (an apoptosis inhibitor) in the case of colon cancer, thereby causing apoptosis and cancer cell death (CitationMandayam et al., 2007). Indomethacin is also reported to decrease EP2 prostanoid receptor expression in colon cancer cells (CitationFujino et al., 2007). When administered orally as an immediate release formulation, it results in side-effects and toxicities due to systemic accumulation of the drug (CitationMarsh, Sachuna, & Sundstrom, 1986). Therefore, a colon-specific formulation of indomethacin would achieve high local concentrations of drug in the colon with reduced systemic absorption, and thereby maximize therapeutic efficacy of the drug in the treatment of this disease. Colon-targeted formulations of indomethacin have been developed utilizing: compression coating technique with guar gum (CitationKrishnaiah et al., 1998) or pectin and chitosan mixtures (CitationFernández-Hervás & Fell, 1998). Indomethacin-loaded colon-targeted pellets have been designed using Eudragit (CitationAkhgari et al., 2005; CitationAkhgari, Sadeghi, & Garekani, 2006) and guar gum with Eudragit FS 30D (CitationJi C, Xu, & Wu, 2007) as coating polymer. Matrix embedded formulations of indomethacin have been designed using guar gum (CitationSinha & Kumria, 2002) and HPMC/pectin/calcium chloride mixture (CitationWu et al., 2007) and a combination of pH responsive polymers with ethyl cellulose (CitationAsghar & Chandran, 2008).
A bimodal (sigmoidal) release profile characterized by negligible to slower release in the initial stage (0–6 h) followed by controlled release during the later stage (6–16 h) will ensure targeted drug release to the colon. Solid dosage forms with such release characteristics can be designed either by coating the drug core with pH-sensitive polymer or incorporating the drug in polymeric matrix. However, the in vivo performance of tablet formulation coated with pH sensitive polymer has been reported to be highly variable (CitationIbekwe et al., 2006). The shallow pH gradient between the small and large intestine, intersubject variability in pH, and gastrointestinal (GI) pH change in diseased states are reported to be the reasons for inconsistent and unpredictable drug release from coated systems during their passage through the GI tract, resulting in release at distal small intestine or no release at all (CitationFriend, 1991,CitationAshford et al., 1993).
A pH- and time-controlled matrix design can mimic the advantage of a coated system and, at the same time, avoid the all or no release phenomenon associated with them. Matrix embedded systems are simple to manufacture and easy to scale up. Multi polymer matrix combining controlled release and pH dependent swelling properties of various polymers can effectively modulate release in a biphasic pattern. A critical issue in designing matrix tablets with bimodal release characteristic is to control the initial drug release.
Hydrophilic polymers like carbopol (CP) and polycarbophil (PCP) which show pH-dependent swelling behavior (pH > 7.0) have been investigated to prepare controlled release formulations with zero-order and near zero-order release kinetics (CitationPerez-Marcos, Iglesias, & Gomez-Amoxa, 1991; CitationLuessen et al., 1995). The pH-dependent swellability of these polymers can be explored for their ability to target drug release to the colon. Ethylcellulose (EC) has been used as a retardant matrix base in sustained and controlled release dosage forms (CitationSaha, Sajeev, & Sahoo, 2001; CitationSajeev & Saha, 2001). Micronized EC, both alone and in combination with excipients, has been extensively investigated as a press coating outer shell around a drug core for imparting delayed release properties (CitationLin et al., 2001; CitationLin, Lin, & Li, 2004). EC has been used in film coating as a single polymer (CitationSinha & Kumria, 2003) and in combination with amylose (CitationLeong et al., 2002) for delaying drug release in colon-targeted systems. A timed release device for theophylline in a matrix composed of EC and hydroxyl ethyl cellulose coated with Eudragit S100 has also been reported as a colon-specific drug delivery system (CitationAlvarez-Fuentes et al., 2004).
The primary objective of the present work was to design and evaluate a pH and transit time controlled colon-specific drug delivery system. The system was designed utilizing a combination of hydrophilic polymer (polycarbophil or carbopol) with pH-dependent swelling property and hydrophobic polymer (ethyl cellulose) capable of imparting delayed release property. Effect of relative polymer proportion on its ability to provide pH-dependent swelling or delayed controlled release characteristics were evaluated.
Experimental
Indomethacin (micronised form) was obtained as a gift sample from Ajanta Pharma Ltd. (Aurangabad, India); Carbopol 934P and Ethylcellulose (N-22 cps, Aqualon, USA) was purchased from S.D. Fine Chemicals and Signet Chem (Mumbai, India), respectively. Polycarbophil (Noveon AA-1) was obtained as gift samples from B.F. Goodrich Chemicals, Mumbai, India. All other chemicals, excipients, and solvents used were of either analytical or pharmaceutical grade.
Tablet manufacturing
Controlled release matrix embedded tablets (each containing 75 mg of indomethacin) prepared using either PCP or CP alone or in combination with EC were prepared by wet granulation technique. Batch quantities of drug and polymer(s) pre-sieved through #120 mesh (ASTM) and dried at 55°C were mixed. The dry blend was granulated with ethyl alcohol (q.s.) and passed through #40 mesh and dried at 55°C on a tray drier. The dried granules were passed through #60 mesh and the passings blended with 1% w/w talc and 0.5% w/w magnesium stearate and compressed using 7 mm punches on a 16 station rotary tablet compression machine (Cadmach, Ahmedabad, India). Three batches of tablets were prepared for each formulation. Formula of prepared matrix embedded tablets is presented in and .
Table 1. Composition, physical characterization, and release rate kinetics of polycarbophil-based formulations.
Table 2. Composition, physical characterization, and release rate kinetics of carbopol-based formulations.
Physical characterization of designed tablets
The designed formulations were studied for their physicochemical properties like weight variation, thickness, crushing strength, friability, and drug content uniformity. For estimating weight variation, 20 tablets of each formulation were weighed on a Mettler Toledo balance (AG135, Mettler Toledo, GMBH, Greifensee, Switzerland). The crushing strength of 10 tablets was measured using Monsanto (standard type) tablet hardness tester. Friability was determined by taking 10 tablets in a Campbell Electronic Friabilator for 4 min at 25 rpm. For estimation of drug content, 10 tablets were crushed and the aliquot of powder equivalent to 10 mg of drug was extracted in methanol:phosphate buffer pH 7.4 (1:9), diluted using phosphate buffer pH 7.4 and analyzed spectrophotometrically at 320 nm.
In vitro release studies
In vitro dissolution studies were carried out using USP Type II (paddle method) apparatus (Electrolab TDT-08L with Autosampling unit, Mumbai, India) at 75 rpm. The dissolution was carried out for the first 2 h in distilled water (500 ml). Then, 200 ml of phosphate buffer concentrate (4.75 g of KH2PO4 and 1.07 g of NaOH in distilled water) was added to raise the total media volume to 700 ml and the pH to 7.4 for the remaining period. At predetermined time intervals, a 10 ml sample was withdrawn and replaced with fresh dissolution media. The samples were filtered, suitably diluted using phosphate buffer pH 7.4, and analyzed spectrophotometrically at 320 nm. The release studies were conducted in duplicate and the mean values from three batches along with the SD were plotted against time ().
Figure 1. Release profile of indomethacin from matrix tablets with varying proportion of PCP. Each data point is expressed as mean ± SD (n = 6).
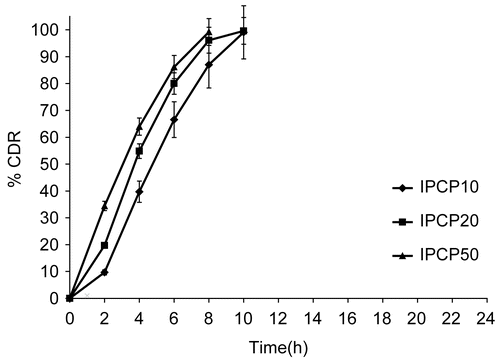
Figure 2. Release profile of indomethacin from matrix tablets with varying proportion of CP. Each data point is expressed as mean ± SD (n = 6).
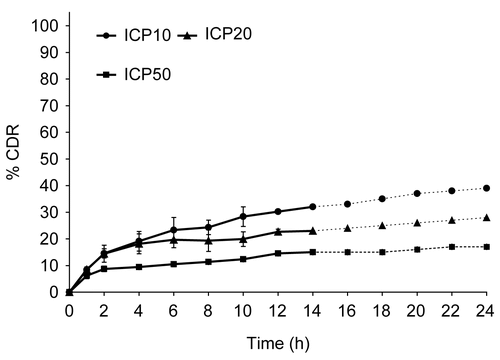
Figure 3. Release profile of indomethacin from matrix tablet showing effect of varying proportion of EC on 10% PCP. Each data point is expressed as mean ± SD (n = 6). The dotted trend line represents the predicted release profile for each formulation beyond 14 h till 24 h.
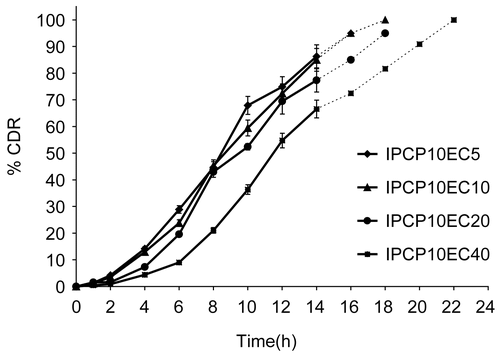
Figure 4. Release profile of indomethacin from matrix tablet showing effect of varying proportion of EC on 20% PCP. Each data point is expressed as mean ± SD (n = 6). The dotted trend line represents the predicted release profile for each formulation beyond 14 h till 24 h.
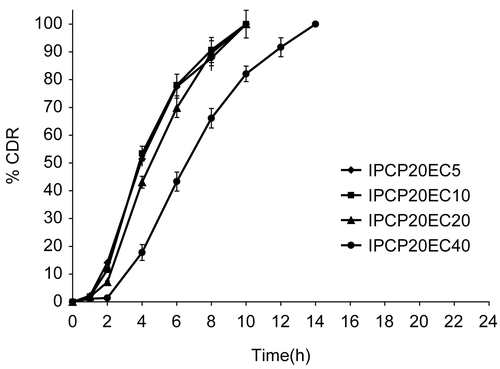
Effect of simulated GI fluid pH (without enzymes) on release
Selected formulations from a previous study were studied in a medium of changing pH. The initial condition was 350 ml of 0.1 N HCl (pH 1.2 for simulated gastric fluid) for 0–2 h. From 2–4 h, the pH of the media was raised to 4.5 (simulated duodenum) by addition of 3.75 g of KH2PO4 in 190 ml and 60 ml of 0.5 M NaOH in distilled water) with total dissolution media volume of 600 ml. From 4 h onwards, the pH was raised to 7.4 (simulated small intestine and colon) by adding 300 ml phosphate buffer concentrate (2.18 g of KH2PO4 and 1.46 g of NaOH in distilled water) to get a dissolution volume of 900 ml. The study was further continued till the end in 900 ml volume. At predetermined time intervals, a 10 ml sample was withdrawn and replaced with fresh dissolution media. After appropriate dilutions, the samples were analyzed by the UV method discussed in the previous section. The corresponding release profiles are presented in .
Characterization of sigmoidal release profiles by power law equation
In order to understand the mechanism of drug release from these formulations, the cumulative percentage drug release data was fitted into the power law equation given by CitationKorsemeyer et al. (1983) and CitationRitger and Peppas (1987).
where, Mt/M∞ is percentage of drug released at any time ‘t’; K is release rate constant incorporating the structural and geometric characteristics of the polymeric system and the drug; n is the diffusion exponent indicative of the release mechanism of the drug. The value of n for a cylinder is < 0.45 for Fickian release (diffusion controlled), > 0.45 and < 0.89 for non-Fickian release (diffusion and polymer relaxation), 0.89 for Case II release (only relaxation and swelling) and > 0.89 for Super case II release (relaxation and erosion). For cylindrical systems like tablets, the n-values of 0.45 and 0.89 represent pure diffusion or erosion controlled release, respectively. The values of the coefficient were calculated based on linear regression analysis between log Mt/M∞ and log t data obtained from drug release studies. The value of n was obtained as slope of the regression equation, and K was calculated as antilog of the intercept value. The t10% (time required for 10% drug release) was determined directly from the plot of cumulative percentage drug released vs time, while the t90% (time required for 90% drug release) was calculated as
The values of correlation time span, K, n, t10% and t90%, and r (correlation coefficient of the regression analysis), as obtained from the dissolution data of designed formulations, are given in and . The correlation time span is the period of drug release phase taken for calculation of release kinetics. Using the calculated values of K and n, the release profiles were predicted beyond 14 h till 24 h for each formulation and are shown as dotted trend line(s) in the respective figures.
Batch reproducibility and stability on storage
Three batches of each formulation were prepared and the release studies were done using the same conditions. In order to assess the long-term stability of the various formulations prepared, selected formulations from each batch were stored at accelerated stability conditions (40 ± 2°C and 75 ± 5% RH) for 6 months. At the end of the study period, the formulations were observed for change in physical appearance, drug content, and in vitro drug release characteristics. The initial (zero time) results were compared with post-stability testing period results for statistical differences.
Data analysis
The difference in the release data between the different formulations was compared using student’s t-test for means and one-way analysis of variance (ANOVA) at 5% level of significance (p < 0.05) using Microsoft Office 2003, Excel package.
Results and discussion
Colonic residence is highly variable and has been reported to vary from 10 h (CitationFollonier & Doelker, 1992) to 30–40 h (CitationHinton et al., 1969). Hence, a drug release program that lasts for 14–16 h would ensure that maximum drug release occurred even when colonic transit time is quite low. Therefore, for colon targeting purpose, a 14–16 h extended release formulation with negligible to no release in the initial 4–6 h would be suitable. This time lag would ensure the passage of the formulation intact through the stomach and small intestine without appreciable drug loss. Based on these assumptions, a theoretical target drug release profile with a sigmoidal character was defined and is shown with other release profiles in simulated GI fluid as target profile.
Polymers like polycarbophil and carbopol, when employed in tablet matrices, tend to swell in alkaline pH to form hydrogels due to the ionization of their acidic groups (CitationHuang & Schwartz, 1995). This ionization results in repulsion between the negative charges. The polymer chains start gyrating and the matrix swells. Drug release is controlled by diffusion through such a swollen gel layer (CitationTaylan et al., 1996). The true challenge is to control drug release from the formulation in the initial stages due to matrix swelling in the presence of dissolution fluid(s). So it was thought to investigate if presence of EC in PCP or CP polymer-based matrix can confer a delayed release character by inhibiting this initial swelling of PCP or CP matrix.
Indomethacin, an indole acetic acid derivative with a pKa of 4.5, has been reported to have solubility of 0.01 mmol/L (3.66 μg/ml) in pH 1.2 and 5.52 mmol/L (1975 μg/ml) in pH 7.2 at 37°C (CitationValizadeh et al., 2004). Our studies reveal that drug (present in a micronized form) has a solubility of ∼ 50 μg/ml in distilled water at 25°C and ∼ 80 μg/ml at 37°C, and, therefore, dissolution was carried out in distilled water for the first 2 h as saturation solubility was not achieved in distilled water even when 65–75% of the complete dose is released. The choice of distilled water as dissolution medium is supported by previous reports that state that as the patient consumes a tablet with a good quantity of water, dissolution of poorly water-soluble drugs can be done in distilled water for the initial period (CitationSheu et al., 1992; CitationSaha et al., 2001). Further, during preliminary studies it was observed that distilled water could discriminate well between the various formulations with and without EC (). Drug release studies were then continued in phosphate buffer (pH 7.4) medium for the remaining period of study. This medium was considered suitable as the drug was freely soluble at this pH and it also mimics the alkaline environment of distal small intestine and colon (CitationMarvola et al., 1999).
The prepared tablets from all the batches were found to be of good quality with acceptable physical characteristics ( and ). The crushing strength was found to vary between 4.0–5.0 kg. The percentage friability was not more than 0.5% while weight variation in all the formulations was limited to within ± 5.0% of the average tablet weight in each batch, respectively. Drug content varied within ±7% of the theoretical formula value (75 mg).
The drug release profiles for matrix tablets showing the effect of varying PCP alone is shown in . The drug release rate was found to be directly proportional to the PCP content in the matrix. The drug release rate was slowest for 10% matrix, IPCP10 (K = 4.201%/h1.46) and fastest for 50% polymer matrix, IPCP50 (K = 20.710%/ h0.78), and release was complete within 6–8 h for all three formulations (). This was in contrast to a previous report wherein indomethacin release was retarded with increase in PCP content (CitationHosny, 1993). Apart from the release rate constant K (%/hn), the release kinetics from the various matrix-based formulations were also compared with respect to t10% and t90%. The observed t10% values were less than 2 h and t90% varied between 6.7–8.2 h. Hence, they were not suitable for colon-specific release. However, a good inverse correlation was found between % PCP in the matrix and t10% (r = 0.8765) and also between % PCP and t90% (r = 0.8923).
In the designed formulations of PCP, as the drug-to-polymer ratio increases from 1:0.1 (for IPCP10) to 1:0.5 (for IPCP50), at higher relative proportion of drug in the matrix, the highly hydrophobic nature of the drug probably resulted in decreased swelling of matrices, thereby giving lower release rates. Whereas, in formulations with higher polymer proportions (IPCP20 & IPCP50), alkaline pH of the dissolution medium resulted in swelling of the polymer leading to rapid erosion of the matrix and a very rapid rate of drug release. Values of diffusional exponent n indicate super case-II mechanism (n > 1) for IPCP10 and IPCP20, while the release mechanism was anomalous (non-Fickian) type in the case of IPCP50. For hydrophobic drugs like indomethacin, a lower relative proportion of PCP in the matrix would be more suited for delaying the initial release.
In the case of carbopol-based formulations, rate of drug release was found to be inversely proportional to the proportion of polymer in matrix (). The release rate was highest for ICP10 (K = 5.411%/h0.74) and slowest for ICP50 (K = 4.966%/h0.37). Though the initial release was very slow in the case of ICP50 (t10% = 4.2 h), the overall drug release in 14 h was found to be very low (10–45%) and hence CP matrix alone was considered unsuitable for this purpose. A good positive correlation was obtained between t10% and % CP in the matrix with an r-value of 0.9672. The fast initial drug release from carbopol matrices (at lower polymer proportions) has been attributed to be due to the quick penetration of water into pores of the polymer matrix and diffusion of the drug through these pores prior to complete gel formation (CitationCooper, Doshi, & Patel, 1995). Increasing the amount of polymer decreased the size of the channels, with increase in the degree of swelling. In this case t90% values were not calculated as the release extended significantly beyond the target profile (). In the present case, the n-values ranged from 0.74–0.37, indicating a shift from non-Fickian (anomalous) release mechanism (at lower polymer proportions) to pseudo-Fickian (ICP50) type of release ().
The release profiles from matrices containing PCP at 10% w/w of drug and varying levels of EC (from 5–40% w/w of drug) revealed that increasing the relative proportion of EC in the matrix retarded drug release during the initial as well as the later stages, as shown in . There was a decrease in the rate of drug release with an increase in proportion of EC. The t10% values ranged from 3.8–5.9 h when EC content was increased from 5–40% w/w of drug in 10% PCP matrix (). There was good correlation between EC level and t10% (r = 0.9957). Similarly, the t90% values varied from 14.5–19.9 h when EC content was increased from 5% to 40% w/w of drug in 10% PCP matrix with a reasonably good correlation with % EC in matrix (r = 0.6994). The presence of ethyl cellulose in a hydrophilic polymer matrix resulted in the decreased penetration of the solvent molecules that led to decreased diffusion of the drug from the matrix (CitationTiwari et al., 2003). It has been shown that high levels of EC reduce drug release rates on account of formation of a strong matrix with reduced porosity (CitationKhan & Meidan, 2007). This increases diffusional path length, leading to reduced dissolution media penetration through the micropores, resulting in slower drug release. It was found that drug release profiles from the prepared PCP and EC matrices followed a sigmoidal pattern ().
When the relative proportion of PCP in the matrix was increased from 10% to 20% w/w of drug (with varying EC proportion as earlier), higher release rates were observed when compared to corresponding 10% PCP + EC matrices. This could be due to enhanced swelling of the matrix with increase in percentage of hydrophilic polymer (). However, the t10% and t90% depended on the proportion of EC, resulting in highest release rates for IPCP20EC5 (K = 7.495%/h1.21; t10% = 1.6 h; t90% = 7.8 h) and slowest release for IPCP20EC40 (K = 0.484%/h2.1; t10% = 3.8 h; t90% = 11.9 h). A good positive correlation was found for both t10% and t90% with % EC in 20% PCP matrix with an r-value of 0.9948 and 0.9291, respectively.
Therefore, it was concluded that inclusion of ethyl cellulose in a polycarbophil matrix base resulted in the formation of a delayed release system with 10% PCP matrix showing a more desirable release profile for colon targeting. In a previous study, the effect of incorporating a hydrophobic polymer Eudragit RL100 on the mechanical and mucoadhesive properties of polycarbophil matrices was investigated (CitationTirosh et al., 1997). Eudragit RL100 was able to significantly decrease the swelling of polycarbophil films in a concentration-dependent manner. The swelling of the mixture was less in pH 5.0 than in pH 7.4. The amount of Eudragit RL100 also correlated well with the rate of erosion of the matrix. A similar effect was observed in our case with incorporation of EC in polycarbophil matrix. Further, values of exponent n () indicate super case-II mechanism which can be attributed to matrix erosion due to the presence of EC accompanied with swelling due to the hydrophilic component.
The effect of EC on drug release from 10% CP matrices is shown in . Increase in relative proportion of EC (from 5% to 40% w/w of drug) resulted in decrease in the release rate of the drug. This effect was similar to that observed for PCP and EC matrices. The increase in percentage of EC correlated well with increase in t10% (r = 0.9849) and t90% (r = 0.9524) values. In the case of CP + EC matrices, both the hydrophilic and hydrophobic polymers were observed to contribute together in retarding drug release from the matrix as opposed to PCP + EC matrix bases wherein increase in PCP proportion enhanced drug release rates. Therefore, when the relative proportion of CP in the matrix was increased from 10% w/w to 20% w/w of drug and EC levels varied from 5–20% w/w of drug, it resulted in a still greater retardation in the release rates, as shown in . The t10% values () ranged from 4.7–7.2 h, which correlated well with % EC (r = 0.9790). The t90% values also increased from 18.2 h to 30.3 h, respectively, and also correlated well with % EC (r = 0.9984). The values of the diffusional exponent n in the case of CP- and EC-based formulations indicate a super case-II type of release, implying that drug release occurred by matrix swelling and erosion. Also, polymer relaxation in alkaline medium contributed to enhanced drug release by diffusion.
Therefore, it was concluded that PCP matrices with an optimized proportion of EC can provide pH- and transit time-based suitable time delay in initial drug release followed by controlled release for colon-specific release. The use of 10% and 20% CP matrix with different proportions of EC resulted in a deviation in some cases (ICP10EC40, ICP20EC20, ICP20EC40) from the theoretical target release beyond 12 h (t90% > 14–16 h), but may still be valuable for colonic delivery in cases where patient colonic transit times are severely prolonged, as is the case with patients suffering from inflammatory bowel disease.
The formulations selected by their closeness to ideal expected profile when studied in simulated GI fluid pH (without enzymes) gave t10% values of 4.5 h for IPCP10EC5, 5.4 h for ICP10EC5 and 4.3 h for ICP20EC5, indicating good potential of these matrix bases as pH- and transit time-controlled sigmoidal release colon-specific formulations (). Increasing the total polymer proportion from 20% (IPCP10EC10) to 40% (IPCP20EC20) resulted in an increase in t10% (8.3 h) and t90% (16.1 h), with the release profile mimicking the expected target sigmoidal profile that is pH- and transit time-dependent (). The selected formulations showed less than 10% release in the first 6 h, with expected complete drug release in 16–18 h (except for ICP20EC5).
No significant difference was observed in the release profile of different batches of each matrix formulation, indicating that the manufacturing process employed was reliable and reproducible. Also, the release kinetics and tablet characteristics remained unaltered after storage at accelerated conditions up to 6 months, suggesting that indomethacin was stable in designed matrices.
Conclusion
The present study was aimed at development of a delayed release system by employing a suitable proportion of hydrophilic polymers with pH-sensitive swelling properties with a hydrophobic polymer that could control the initial swelling of the matrix base. Such formulations are expected to show pH- and transit time-dependent sigmoidal release profiles. The effect of inclusion of ethyl cellulose in a polymeric matrix was statistically significant (at 5% level) when compared to pure polycarbophil and carbopol matrices in initial retardation of drug release followed by controlled release for a period ranging from 14–16 h in most cases. It was shown that a suitable time lag was attained with acceptable release kinetics that could ensure site-specific drug release in the colon. A matrix design of this kind can serve as an alternative strategy to enteric film and press coating techniques commonly employed for design of delayed release systems. Such systems will have the added advantage of easy commercial scalability for large scale production.
Acknowledgements
Declaration of interest: The authors report no conflicts of interest. The authors alone are responsible for the content and writing of the paper.
References
- Akhgari, A., Garekani, H.A., Sadeghi, F., Azimaie, M. (2005). Statistical optimization of indomethacin pellets coated with pH dependent methacrylic polymers for possible colonic drug delivery. Int J Pharm, 305, 22–30.
- Akhgari, A., Sadeghi, F., Garekani, H.A. (2006). Combination of time-dependent and pH-dependent polymethacrylates as a single coating formulation for colonic delivery of indomethacin pellets. Int J Pharm, 320, 137–42.
- Alvarez-Fuentes, J., Fernández-Arévalo, M., González-Rodríguez, M.L., Cirri, M., Mura, P. (2004). Development of enteric-coated timed-release matrix tablets for colon targeting. J Drug Target, 12, 607–12.
- Asghar, L.F.A., Chandran, S. (2008). Design and evaluation of pH modulated controlled release matrix systems for colon specific delivery of indomethacin. Pharmazie, 63, 736–42.
- Ashford, M., Fell, J.T., Attwood, D., Woodhead, P.J. (1993). An in vitro investigation into the suitability of pH-dependent polymers for colonic targeting. Int J Pharm, 91, 193–9.
- Basit, A.W. (2005). Advances in colonic drug delivery. Drugs, 65, 1991–2007.
- Cooper, A.M., Doshi, D.H., Patel, M.V. (1995). In vitro prolonged release of basic CNS compounds from HPMC and Carbopol 974P hydrophilic matrixes: polymer/drug ionization and counterion effects. Proc Int Symp Contr Rel Bioact Mater, 22, 288–9.
- Fernández-Hervás, M.J., Fell, J.T. (1998). Pectin/chitosan mixtures as coatings for colon-specific drug delivery: an in vitro evaluation. Int J Pharm, 169, 115–9.
- Follonier, N., Doelker, E. (1992). Biopharmaceutical comparison of oral multiple-unit and single-unit sustained-release dosage forms. STP Pharma Sci, 2, 141–55.
- Friend, D.R. (1991). Colon-specific drug delivery. Adv Drug Deliv Rev, 7, 149–99.
- Fujino, H., Chen, X.B., Regan, J.W., Murayama, T. (2007). Indomethacin decreases EP2 prostanoid receptor expression in colon cancer cells. Biochem Biophys Res Commun, 359, 568–73.
- Hinton, J.M., Lennard-Jonnes, J.E., Young, A.C. (1969). A new method for studying gut transit times using radioopaque markers. Gut, 10, 842.
- Hosny, E.A. (1993). Polycarbophil as a controlled release matrix. Int J Pharm, 98, 235–8.
- Huang, L-L., Schwartz, J.B. (1995). Studies on drug release from a carbomer tablet matrix. Drug Dev Ind Pharm, 21, 1487–501.
- Hull, M.A., Gardner, S.H., Hawcroft, G. (2003). Activity of the non-steroidal anti- inflammatory drug indomethacin against colorectal cancer. Cancer Treat Rev, 29, 309–20.
- Ibekwe, V.C., Liu, F., Fadda, H.M., Khela, M.K., Evans, D.F., Parsons, G.E., Basit, A.W. (2006). An investigation into the in vivo performance variability of pH responsive polymers for ileo-colonic drug delivery using gamma scintigraphy in humans. J Pharm Sci, 95, 2760–6.
- Ji, C., Xu, H., Wu, W. (2007). In vitro evaluation and pharmacokinetics in dogs of guar gum and Eudragit FS 30D-coated colon-targeted pellets of indomethacin. J Drug Target, 15, 123–31.
- Kapitanović, S., čačev, T., Antica, M., Kralj, M., Cavrić, G., Krešimir, P., Spaventi, R. (2006). Effect of indomethacin on E-cadherin and β-catenin expression in HT-29 colon cancer cells. Exp Mol Path, 80, 91–6.
- Khan, G.M., Meidan, V.K. (2007). Drug release kinetics from tablet matrices based upon ethylcellulose ether-derivatives: a comparison between different formulations. Drug Dev Ind Pharm, 33, 627–39.
- Kinget, R., Kalala, W., Vervoort, L., Mooter, G.U. (1998). Colonic drug targeting. J Drug Target, 6, 129–49.
- Korsmeyer, R.W., Gurny, R., Doelker, E., Buri, P., Peppas, N.A. (1983). Mechanisms of solute release from porous hydrophilic polymers. Int J Pharm, 15, 25–35.
- Krishnaiah, Y.S.R., Satyanarayana, S., Rama, Prasad YV, Narasimha, R.S. (1998). Evaluation of guar gum as a compression coat for drug targeting to colon. Int J Pharm, 171, 137–46.
- Leong, C.W., Newton, J.M., Basit, A.W., Podczeck, F., Cummings, J.H., Ring, S.G. (2002). The formation of colonic digestible films of amylose and ethylcellulose from aqueous dispersions at temperatures below 37°C. Eur J Pharm Biopharm, 54, 291–7.
- Lin, S.Y., Lin, K.H., Li, M.J. (2001). Micronized ethylcellulose used for designing a directly compressed time-controlled disintegration tablet. J Contr Rel, 70, 321–8.
- Lin, S.Y., Lin, K.H., Li, M.J. (2004). Formulation design of double-layer in the outershell of dry-coated tablet to modulate lag time and time-controlled dissolution function: studies on micronized ethylcellulose for dosage form design (VII). AAPS Journal, 6 (3) Article 17. Available online at: http://www.aapsj.org, Oct 31, 2008.
- Luessen, H.L., Verhoef, J.C., Borchard, G., Leh, C.M., de Boer, A.G., Junginger, H.E. (1995). Mucoadhesive polymers in peroral peptide drug delivery. II. Carbomer and polycarbophil are potent inhibitors of the intestinal proteolytic enzyme trypsin. Pharm Res, 12, 1293–8.
- Mandayam, S., Huang, R., Tarnawski, A.S., Chiou, S.K. (2007). Roles of survivin isoforms in the chemopreventive actions of NSAIDS on colon cancer cells. Apoptosis, 12, 1109–16.
- Marsh, C.C., Sachuna, A.A., Sundstrom, W.R. (1986). A review of selected investigational non steroidal anti inflammatory drugs of the 1980’s. Pharmacotherapy, 6, 10–25.
- Marvola, M., Nykanen, P., Rautio, S., Isonen, N., Autere, A.M. (1999). Enteric polymers as binders and coating materials in multiple-unit site-specific drug delivery systems. Eur J Pharm Sci, 7, 259–67.
- Perez-Marcos, B., Iglesias, R., Gomez-Amoxa, J.L. (1991). Mechanical and drug release properties of atenolol-carbomer hydrophilic matrix tablets. J Contr Rel, 17, 267–76.
- Ritger, P.L., Peppas, N.A. (1987). A simple equation for the description of solute release. I. Fickian and non-Fickian release from non-swellable devices in the form of slabs, spheres, cylinders or discs. J Contr Rel, 5, 23–36.
- Rubinstein, A. (1995). Approaches and opportunities in colon- specific drug delivery. Crit Rev Ther Drug Carrier Syst, 12, 101–49.
- Saha, R.N., Sajeev, C., Sahoo, J. (2001). A comparative study of controlled release matrix tablets of diclofenac sodium, ciprofloxacin hydrochloride and theophylline. Drug Deliv, 8, 149–54.
- Sajeev, C., Saha, R.N. (2001). Formulation and comparative evaluation of controlled release diclofenac tablets prepared by matrix- embedding technique, membrane barrier technique and combination of the two. Drug Dev Res, 53, 1–8.
- Sheu, M.T., Chou, H.L., Kao, C.C., Liu, C.H., Sokoloski, T.D. (1992). Dissolution of diclofenac sodium from matrix tablets. Int J Pharm, 85, 57–63.
- Sinha, V.R., Kumria, R. (2002). Binders for colon specific drug delivery: an in vitro evaluation. Int J Pharm, 249, 23–31.
- Sinha, V.R., Kumria, R. (2003). Coating polymers for colon specific drug delivery: a comparative in vitro evaluation. Acta Pharm, 53, 41–7.
- Taylan, B., Capan, Y., Guven, O., Kes, S., Hincall, A.A. (1996). Design and evaluation of sustained release and buccal adhesive propranolol hydrochloride tablets. J Contr Rel, 37, 11–20.
- Tirosh, B.M., Baloum, T., Nassar, M., Friedman, A., Rubinstein, A. (1997). The effect of Eudragit RL-100 on the mechanical and mucoadhesion properties of polycarbophil dosage forms. J Contr Rel, 45, 57–64.
- Tiwari, S.B., Krishna Murthy, T., Pai, R.M., Mehta, P.R., Chowdary, P.B. (2003). Controlled release formulation of tramadol hydrochloride using hydrophilic and hydrophobic matrix system. AAPS PharmSciTech, 4 (3) Article 31. Available online at: http://www.pharmscitech.org, Oct 31, 2008.
- Valizadeh, H., Nokhodchi, A., Qarakhani, N., Zakeri-Milani, P., Azarmi, S., Hassanzadeh, D., Löbenberg, R. (2004). Physicochemical characterization of solid dispersions of indomethacin with PEG 6000, Myrj 52, lactose, sorbitol, dextrin, and Eudragit E100. Drug Dev Ind Pharm, 30, 303–17.
- Wu, B., Deng, D., Lu, Y., Wu, W. (2007). Biphasic release of indomethacin from HPMC/pectin/calcium matrix tablet: II. Influencing variables, stability and pharmacokinetics in dogs. Eur J Pharm Biopharm, 67, 707–14.