Abstract
Three formulations including methylene blue solution (MB-S), MB water-in-oil microemulsion (MB-ME), and MB multiple microemulsion (MB-MME) were prepared with the aim to evaluate whether the three formulations can carry MB target to regional lymph nodes and show lymphatic tropism after subcutaneous administration. The pseudo-ternary phase diagrams were also studied. The morphology of MB-ME and MB-MME was examined by transmission electron microscopy to characterize the microstructure. The particle size and viscosity were also measured. MB concentrations in plasma, lymph nodes, and limb soles were quantitatively analyzed using HPLC. Results show that MME can target MB to regional lymph nodes, and can be employed as a potential lymph tracer in sentinel lymph node biopsy.
Introduction
The presence of lymph node metastases is an independent prognostic factor for overall and disease-specific survival and local recurrence. Most of the cancer patients were detected to suffer from lymph node metastases. Even after the surgical operation, lymph node metastases are still a serious problem. As a result, the elective lymph node dissection (ELND) is the most important means which improves the quality of patients’ life and prolongs their life span. The significant change occurred in the early 1990s when sentinel lymph node biopsy (SLNB) was introduced for the management of cutaneous melanoma patients without clinical evidence of regional lymph node metastases (Morton et al., Citation1992; CitationAlex et al., 1993). SLNB is proposed as an alternative technique to ELND, which is minimally invasive, with less morbidity, and is able to identify patients at high risk who may benefit from additional therapies such as complete lymphadenectomy or adjuvant interferon. Currently, there are three methods for SLNB, including radio nucleotide tracers assisted SLNB, dye assisted SLNB, and a combination of the two (CitationReitsamer et al., 2003; CitationKhalil et al., 2007). Since CitationGiuliano et al. (1994) used 1.0% isosulfan blue (IB) for breast SLNB, doctors from different countries have tried various dyes with a view to achieve the best results of SLNB.
The microemulsion concept was introduced as early as the 1940s by CitationHoar and Schulman (1943), who generated a clear single-phase solution by titrating a milky emulsion with hexanol. CitationSchulman et al. (1959) subsequently coined the term microemulsion. Microemulsions are thus defined as ‘a system of water, oil and amphiphile which is a single optically isotropic and thermodynamically stable liquid solution’. Currently, microemulsions as a novel Drug Delivery System (DDS) has been widely used, which can change drug absorption, distribution, and pharmacokinetic parameters, delay drug release, and play a role in drug targeting. Multiple water-in-oil-in-water microemulsions are complex liquid dispersions in which oil globules, containing small droplets of water, are dispersed in an aqueous continuous phase (CitationFlorence and Whitehill, 1982; CitationBenichou et al., 2004). In the last 15 years, these emulsion systems have proved their interest for the encapsulation of various active compounds such as hormones (CitationCole and Whateley, 1997; CitationCournarie et al., 2004), steroids (CitationLaugel et al., 1998), and antiseptics (CitationTedajo et al., 2005).
The emulsion can promote and prolong lymphatic transport of ontazolast (CitationHaus et al., 1998). It is suggested that emulsion may have potential as lymphotropic delivery systems that to date has not been fully exploited. Preferential lymphatic transport of mitomycin C has been demonstrated following the injection of W/O or O/W emulsions via the intraperitoneal and intramuscular routes (CitationHashida et al., 1997). It was reported that selective uptake after injection into the regional lymphatics occurred in the order of O/W, W/O, aqueous solution. CitationHashida et al. (1977; Citation1997) developed gelatin spheres in oil (S/O) emulsion for minimizing the instability of the W/O emulsion. The nanoparticle-in-oil emulsion system, containing anti-filarial drug in gelatin nanoparticles, was studied for enhancing lymphatic targeting (CitationKarajgi and Vyas, 1994), and it was suggested that this colloidal system held excellent potential as a lymphotropic carrier system. More recently, an emulsion formulation consisting of an anticancer drug, Pirarubicin, and Lipiodol was developed to treat cancer and metastatic lymph nodes (CitationYoshimura and Nunomura, 1996). After endoscopic injection of the Pirarubicin–Lipiodol emulsion, the drug was retained over 7 days at the injection site and in the regional lymph node.
Dye-directed SLNB has been widely accepted, due to its high accuracy and convenience in clinical practice without radioactive contamination or expensive instruments. Until now, several vital dyes have been developed, like IB, patent blue (PB) (CitationKoller et al., 1998; CitationNoguchi et al., 1999), and methylene blue (MB). IB and PB with a weak combination of protein will be quickly absorbed into lymphatic vessels after being subcutaneously injected, and rarely spread to the surrounding tissues. However, the relatively rapid washout from sentinel lymph node (SLN) and the expensive price limit their use in SLNB (CitationLiu et al., 2000). As the most commonly used dye, MB can reside in lymph nodes for a long time for a cheaper price (CitationYuan et al., 2000). MB is a reductant-oxidant, which is often used as an antidote in clinical cyanide poisoning (CitationSun et al., 1995; CitationSteffen, 2007). Recent studies show that it possesses some analgesic effects (CitationKumar et al., 1995; CitationTang et al., 1996). MB can also significantly destruct various types of human lung cancer cells, and inhibit the proliferation of Ehrlich ascites carcinoma cells. Excessive doses of MB used in SLNB could cause lymphatic vessels, tissue spaces, and blood capillaries to be stained blue, and small doses could not obviously stain regional lymph nodes, resulting in a low detection rate of SLNB.
In this study, we developed three MB formulations including MB solution (MB-S), MB water-in-oil microemulsion (MB-ME), and MB multiple microemulsion (MB-MME), and used them for the lymph node delivery in mice. The main aim of the study was to evaluate whether the three formulations carrying MB can target to regional lymph nodes and show lymphatic tropism after subcutaneous administration. To the best of our knowledge, the MB lymph node delivery system was studied for the first time.
Materials and methods
Materials
Methylene blue was purchased from Shenyang agent Co. (Shenyang, China). The internal standard Chloropromazine was obtained from the Institute for Drug Control of Liaoning Province (China), and Crodamol GTCC, Span 80, Span 85, and Tween 80 were kindly provided by Croda Co. (England). Cremophor® RH 40 and Solutol® HS 15 was from BASF and transcutol P was from Gattefosse. Other reagents of HPLC or analytical grade were from Yuwang Reagent Co. (Shandong, China).
Animals
Female KM mice (20 ± 2 g) were supplied from the Laboratory Animal Center, Shenyang Pharmaceutical University (Shenyang, China). The mice were housed in room temperature and humidity, and had access to water and food ad libitum.
Preparation of MB-S, MB-ME and MB-MME
MB-S was prepared by dissolving MB in 5% glucose injection to obtain the concentration of 1% w/w.
After microemulsion regions in phase diagrams were identified, the microemulsion was prepared using Crodamol GTCC (oil phase), Span 80-Tween 80 of HLB = 8 (surfactant), transcutol P (co-surfactant), and 5% glucose injection (aqueous phase) at the ratios of 5.4:3.6:0.54:0.36. Briefly, MB and transcutol P were solubilized in the aqueous phase, followed by adding oil phase and surfactant with magnetic stirring at 45 ± 3°C. Then the mixture was allowed to cool to room temperature. The water-in-oil microemulsion containing MB was obtained at the level of 1% w/w.
MB-MME was prepared at room temperature by using a two-step emulsification procedure (CitationBibette et al., 1997; CitationGoubault et al., 2001). The primary microemulsion containing MB at the level of 8% was prepared using ethyl oleate, Span 80-Tween 80 of HLB = 8, transcutol P, and 5% glucose injection at the ratios of 6.6:1.6:0.55:0.45. MB-MME was obtained by a combination of the primary microemulsion, HS 15, ethanol, and the aqueous phase at the ratios of 1.25:3.3:1.1:4.35. The final MB-loaded multiple microemulsion was also at the level of 1% w/w.
Characterization of MB-ME and MB-MME
The morphology of MB-ME and MB-MME was examined using transmission electron microscopy (TEM). A drop of MB-ME and MB-MME were applied respectively to a carbon-coated copper grid and left for 1 min to allow some of the particles to adhere to the carbon substrate. The excess of dispersion was removed by adsorbing the drop with a piece of filter paper. A drop of 1% phosphotungstic acid solution was applied and again excess solution was removed by adsorbing the liquid with the tip of a filter paper, and the sample was air-dried. The sample was then observed under a transmission electron microscope (JEM-1200EX, Japan).
The particle size and polydispersity of MB-ME and MB-MME were measured by Zetasizer nano ZS90 (Malvern, England). The viscosity was also measured.
Animal experiment
Fifty-four KM mice were randomly assigned to three groups. Each group contained 18 mice. Separately, MB-S, MB-ME, and MB-MME were administered subcutaneously to the right hind limb sole of the mice with the dose of 10 ng/g.
At each time point (10, 20, 30, 60, 90, 120 min after dosing), blood samples (0.5 ml) were obtained from fossa orbitalis vein of three mice of each group. Then blood samples were put into heparinized micro- centrifuge tubes and followed by centrifuging at ~5000 × g for 10 min. The resulting plasma layers were separated and stored in micro centrifuge tubes at −20°C until analysis.
After blood samples were got, the mice were sacrificed. Right popliteral lymph nodes and right hind limb soles of mice were harvested immediately. The tissue samples were rinsed with saline solution, blotted-dry with filter paper, and weighed, then stored at −20° until analysis.
Sample preparation
Plasma sample preparation
Frozen plasma samples were thawed. An aliquot (100 μl) of plasma sample was measured into a glass tube with a teflonlined cap, followed by the addition of 1.5 mg/ml chloropromazine solution (10 μl) as the internal standard and 1.0 ml acetonitrile. The mixture was vortexed for 15 min and centrifuged at 5000 × g for 15 min. Supernatants were obtained through this procedure, and it was dried under a stream of nitrogen and re-dissolved in 100 μl mobile phase, vortexed for 3 min, and centrifuged at 5000 × g for 5 min, and then 20 μl of the supernatant was injected to HPLC analysis of MB under the conditions described below.
Lymph nodes sample preparation
Frozen lymph node samples were thawed, and homogenized in saline (4°C) with a ratio of 1:2 (mg/ml) using a handheld glass homogenizer. The homogenates were mixed with 10 μl chloropromazine solution and 1.0 ml acetonitrile. The supernatant was extracted as described above.
Injection site tissues sample preparation
Frozen right hind limb sole samples were thawed, and homogenized in saline (4°C) with a ratio of 1:20 (g/ml) using a homogenizer. An aliquot (100 μl) of the homogenates was mixed with 10 μl chloropromazine solution and 1.0 ml acetonitrile. The supernatant was extracted as described above.
HPLC assay
HPLC conditions
The MB concentrations in all samples were determined by reversed phase HPLC using Jiangshen series (Dalian, China). The HPLC system consisted of an LC-10AT pump, and an SPD-10A UV detector. Separation was performed using a prepacked stainless steel column (200 × 4.6 mm i.d.) with Hypersil C18 5 μm silica. The mobile phase was a pH 2.0 phosphate buffer–acetonitrile–triethylamine (70:30:0.3, v/v/v) mixture with a flow rate of 1.0 ml/min. The detection wavelength was set at 292 nm, and the system was operated at room temperature.
Method validation
Chloropromazine, as the internal standard, was added to each standard containing known amounts of MB. Each sample was prepared in duplicate. The peak-area ratios (MB to Chloropromazine) were determined in duplicate. The calibration curves were obtained by a least-squares linear fitting of the peak-area ratios vs the amounts of MB. Intra- and inter-run precision were assessed from the results of QCs. The mean values and R.S.D. for QCs at three concentration levels were calculated over three validation runs. Six replicates of each QC level were determined in each run. The data was then used to calculate the intra- and inter-run precision (R.S.D.) by a one-way analysis of variance. The accuracy of the method was determined by calculating the percentage deviation observed in the analysis of QCs and expressed as the relative error.
Statistical analysis
All the experimental data was subjected to statistical analysis, using one-way analysis of variance (ANOVA), and a p-value of 0.05 was considered to be statistically significant.
Results and discussion
Phase diagram studies
In this study, with the criteria of microemulsion region, factors affecting the formation of MB-ME and MB-MME were studied. The construction of phase diagrams makes it easy to find out the concentration range of components for microemulsions.
As to MB-ME, soybean oil, castor oil, efamol, oleum fructus bruceae, and crodamol GTCC were investigated as the oil phase, and results showed that crodamol GTCC was the most ideal candidate to make low viscosity and stable microemulsion. It is generally accepted that low HLB (3–8) surfactants are favored for the formation of water-in-oil microemulsion system. Different HLB values of Span 80-Tween 80 and Span 85-Tween 80 system have been studied, which shows that the microemulsion region increased as the HLB value increased, and low viscosity and large microemulsion region were obtained when Span 80-Tween 80 (HLB = 8) was used (). The pseudo-ternary phase diagrams of Span 80-Tween 80 (HLB = 8) and different co-surfactants were described in . It is suggested that glycerol, PEG200, and PEG400 were less effective for microemulsion formation, while ethanol, propylene glycol, and transcutol P can significantly increase the microemulsion region. Incorporation of co-surfactants increased the maximum amount of incorporated water in the oil–surfactant system, with the microemulsion region being increased in all cases compared to the co-surfactant free system. Ethanol, propylene glycol, and transcutol P are amphiphilic small molecules, which can improve the HLB value of surfactants and increase the curvature of the oil–water interface membrane, then lower the oil–water interfacial tension. The co-surfactant can more easily penetrate to the polyoxyethylene chain and the hydrophobic carbon chain of the surfactant, forming a mixed adsorption layer, enhancing the mobility and flexibility of the interface membrane, avoiding the formation of liquid crystal gel, and ultimately forming microemulsions. The optimized microemulsion system was GTCC/Span 80-Tween 80 (HLB = 8)/60% Transcutol P system.
Figure 1. Effects of HLB value and co-surfactants on the pseudo-ternary phase diagrams of MB-ME. (The grey region represents microemulsions.) (a) Effect of HLB value; (b) effect of co-surfactants.
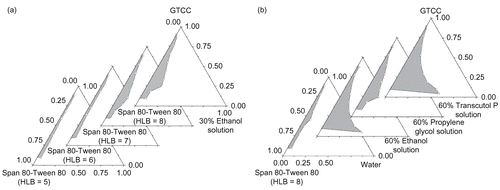
In order to prepare stable MB-MME, ethyl oleate, which is a smaller molecular and can easily penetrate to the interface membrane, was employed as the oil phase to prepare the primary water-in-oil microemulsion. HS 15 and ethanol were optimized as the surfactant and the co-surfactant ().
Characterization of MB-ME and MB-MME
The transmission electron microscopic images of MB-ME and MB-MME are presented in . The emulsion drops are spherical in shape and well dispersed. The average diameters of MB-ME and MB-MME were 74.11 nm and 100.7 nm, with the polydispersity of 0.058 and 0.159. Their viscosities were 102.75 mPa·S and 14.93 mPa·S, respectively.
Method validation tests
Calibration curves for MB in plasma, right popliteral lymph nodes, and right hind limb soles were obtained. Calibration equations, linear range, and correlation coefficients are presented in . The results indicated that there was a good linear relationship between peak-area ratio (Y) of MB to I.S. and drug concentration (X) for the concentration range. The limit of quantification (LOQ) of plasma, lymph nodes, and limb soles were 80 ng/ml, 8 ng/mg, and 0.4 ng/mg, respectively. The stability, recovery, linearity, accuracy, and specificity of the method were evaluated in agreement with the criteria widely accepted. The intra-day and inter-day precisions of plasma, lymph nodes, and limb soles of high, medium, and low concentrations were within 15%, which indicated that the method was reproducible. The extraction recoveries of MB in plasma, lymph nodes, and limb soles were 94.3%, 95.5%, and 91.0%, respectively. Therefore, this new method for the analysis of biological samples exhibited good precision and accuracy, coupled with a high recovery.
Table 1. Calibration curves for blood and tissues.
Study on MB concentration in plasma
According to the data got from HPLC analysis, MB can be easily detected in plasma after MB-S administration (), while MB can not be detected after MB-ME and MB-MME subcutaneously injected, respectively. As is known, the gap between blood capillaries is 30–50 nm, while the lymphatic wall is made of monolayer endothelial cells, imbricated-arranged and loosely connected, and the gap is 500 nm, which could reach 5 μm when pathological changes appeared. Consequently, MB-S can easily enter the blood capillary, and then participate in the blood circulation. Whereas MB-ME and MB-MME can only penetrate the lymphatic capillary but not the blood capillary, owing to the particle size (larger than 50 nm), then stained regional lymph nodes to play a role in lymphatic tracing. The superiority of MB-ME and MB-MME is that they can’t enter the blood circulation, and thereby avoid blood capillaries and surrounding tissues to be blue-colored, resulting in more accurate and effective tracing.
Table 2. MB concentration in plasma after subcutaneous administration with the dose of 10 ng/g. The data are the arithmetic mean values ± SD, n = 3.
Uptake and retention of MB in lymph nodes
The lymph node distributions of MB given in various formulations were measured in right popliteal lymph nodes. As shown in , MB can be detected in popliteal lymph nodes, 10 min after subcutaneously administration to the right hind limb sole. As to MB-ME, the concentration of MB increased with time, reaching the maximum at 90 min, and then decreased due to the high viscosity of the microemulsion (102.75 mPa·S). As a result, its removal speed was small in vivo, and the max concentration was obtained at 90 min. When the time continued to increase, the microemulsion had transferred to the next lymph node via the lymphatic circulation. And, therefore, the concentration of MB reduced. However, in the MB-S and MB-MME groups, the biggest concentration of MB was observed at the first time point (10 min). After that, the concentration began to decrease. It is suggested that MB-S and MB-MME possess a high transferring speed, and a large amount of MB reaches popliteal lymph nodes within 10 min, then moves to next lymph nodes. In addition, the max concentration of MB in MB-S and MB-MME groups was over 3-fold higher than that in the MB-ME group. It was observed that right popliteal lymph nodes of the mice in MB-S and MB-MME at any time point were obviously blue-colored, while the blue-stained lymph nodes can be seen after 60 min in the MB-ME group. The results indicate that the colored lymph nodes could be observed, only when the concentration of MB reached 0.456 μg/mg. Conclusions can be drawn that MB-S and MB-MME show better lymphatic tropism compared with MB-ME (p < 0.01). However, there is no significant difference between MB-S and MB-MME (p > 0.05).
Figure 4. The concentration of MB in popliteal lymph nodes after subcutaneous administration (the data are the arithmetic mean values ± SD, n = 3).
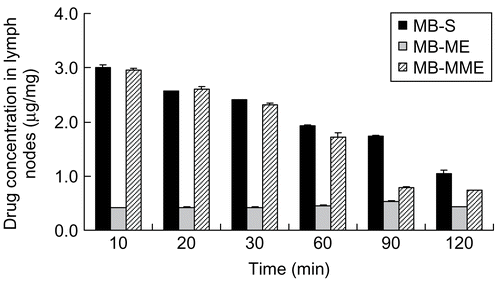
The non-compartmental pharmacokinetic parameters are shown in . AUC(0–120 min) values for mice treated with 1% (w/w) MB-S, MB-ME, and MB-MME were 5020, 3819, and 5188 mg·min/g, respectively. It indicated a higher amount of MB in MB-S and MB-MME disposed in lymph nodes than MB-ME did. Separately, MRT(0–120 min) of MB-S, MB-ME, and MB-MME were 151.96, 332.11, and 198.46 min. Conclusions can be drawn that MB resided in lymph nodes for a long time after MB-ME administration compared with the other two formulations.
Table 3. Pharmacokinetic parameters after 10 ng/g subcutaneous administration of MB-S, MB-ME, and MB-MME.
There are more lipophilic components in emulsions (CitationLiang, 2005) which have a certain affinity with lymph resulting in the character of natural lymphatic targeting. The lymphatic uptake of various types of the emulsion was in the following order: the multiple emulsions, water-in-oil emulsion, oil-in-water emulsion, which is consistent with our results.
Clearance from the injection site
As shown in , 22% of MB in MB-ME was cleared from the injection site within 10 min, while ~50% of MB in MB-S and MB-MME was done. MB in MB-ME disappeared from the injection site slower than MB in MB-S and MB-MME. At 120 min after administration, nevertheless, ~45% in MB-ME, 27% in MB-MME, and 27% in MB-S of MB remained at the injection site, respectively. The higher clearance of MB in MB-MME and MB-S compared with that in MB-ME in the initial phase from the injection site might result from the different outer phases and the viscosities. On the one hand, the outer phase of the multiple microemulsion was water, which was compatible with lymphatics, and can be easily absorbed. On the other hand, the different viscosities lead to different moving speeds in lymph circulation. It is can be concluded that MB-S and MB-MME could be cleared away from the injection site faster than MB-ME (p < 0.01). However, no significant difference can be observed between MB-S and MB-MME (p > 0.05).
Conclusions
Results indicate that MB-S and MB-MME showed good lymphatic tropism. However, MB-S can enter the blood circulation, blue-staining capillaries and surrounding tissues, resulting in inaccurate SLNB. Thus, MB-MME can be employed as a potential lymph tracer in SLNB.
Acknowledgements
Declaration of interest: The authors report no conflicts of interest. The authors alone are responsible for the content and writing of the paper.
References
- Alex, J. C., Weaver, D. L., Fairbank, J. T., Rankin, B. S., and Krag, D. N. (1993). Gamma-probe-guided lymph node localization in malignant melanoma. Surg Oncol, 2, 303–8.
- Benichou, A., Aserin, A., and Garti, N. (2004). Double emulsions stabilized with hybrids of natural polymers for entrapment and slow release of active matters. Adv Colloid Interf, 108–9, 29–41.
- Bibette, J., Leal Calderon, F., and Gorria, P. (1997). Emulsion double polydisperse, émulsion double monodisperse correspondante et procédé de préparation de l’émulsion monodisperse. French Patent, CNRS 97101154, August 2007.
- Cole, M. L., and Whateley, T. L. (1997). Release rate profiles of theophylline and insulin from stable multiple w/o/w emulsions. J Contr Rel, 49, 51–8.
- Cournarie, F., Savelli, M. P., Rosilio, V., Bretez, F., Vauthier, C., Grossiord, J. L., and Seiller, M. (2004). Insulin-loaded W/O/W multiple emulsions: Comparison of the performances of systems prepared with medium-chain-triglycerides and fish oil. Eur J Pharm Biopharm, 58, 477–82.
- Florence, A. T., and Whitehill, D. (1982). The formulation and stability of multiple emulsions. Int J Pharm, 11, 277–308.
- Giuliano, A. E., Kirgan, D. M., and Guenther, J. M. (1994). Lymphatic mapping and sentinel lymphadenectomy for breast cancer. Ann Surg, 220, 391–8.
- Goubault, C., Pays, K., Olea, D., Gorria, P., Bibette, J., Schmitt, V., and Leal-Calderon, F. (2001). Shear rupturing of complex fluids: Application to the preparation of quasi-monodisperse water-in-oil-in water double emulsions. Langmuir, 17, 5184–8.
- Hashida, M., Egawa, M., Muranishi, S., and Sezaki, H. (1997). Role of intra-muscular administration of water-in-oil emulsions as a method for increasing the delivery of anticancer agents to regional lymphatics, J Pharmacokin Biopharm, 5, 223–39.
- Hashida, M., Muranishi, S., and Sezaki, H. (1977). Evaluation of water in oil and microsphere in oil emulsions as a specific delivery ystem of 5-fluorouracil into lymphatics. Chem Pharm Bull, 25, 2410–8.
- Haus, D. J., Fogal, S. E., Ficorilli, J. V., Price, C. A., Roy, T., Jayara, A. A., and Keirns, J. J. (1998). Lipid-based delivery systems for improving the bioavailability and lymphatic transport of a poorly water-soluble LTB4 inhibitor. J Pharm Sci, 87, 164–9.
- Hoar, T. P., and Schulman, J. H. (1943). Transparent water-in-oil dispersions: the oleopathic hydro-micelle. Nature, 152, 102–3.
- Karajgi, J. S., and Vyas, S. P. (1994). A lymphotropic colloidal carrier system for diethylcarbamazine: preparation and performance evaluation. J Microencapsulation, 11, 539–45.
- Khalil, H. H., Elaffandi, A. H., Afifi, A., Alsayed, Y., Mahboub, T., and El-Refaie, K. M. (2008). Sentinel Lymph Node Biopsy (SLNB) in management of N0 stage T1–T2 lip cancer as a ‘same day’ procedure. Oral Oncology, 44, 608–612.
- Koller, M., Barsuk, D., Zipple, D., Engelberg, S., Ben-Ari, G., and Papa, M. Z. (1998). Sentinel lymph node involvement—a predictor for axillary node status with breast cancer—has the time come?. Eur J Surg Oncol, 24, 166–8.
- Kumar, A., Raghubir, R., and Dhawan, B. N. (1995). Possible involvement of nitric oxide in red nucleus stimulation-induced analgesia in the rat. Eur J Pharmacol, 279, 1–5.
- Laugel, C., Baillet, A., Youenang Piemi, M. P., Marty, J. P., and Ferrier, D. (1998). Oil–water–oil multiple emulsions for prolonged delivery of hydrocortisone after topical application: Comparison with simple emulsions. Int J Pharm, 160, 109–17.
- Liang, W. Q. (2005). Biopharmacy and pharmacokinetics. Beijing: People’s Health Press. p. 103.
- Liu, Q., Zuo, W. S., Wang, Y. S., Wang, L., Li, M., and Yu, Z. Y. (2000). The study of sentinel lymph nodes biopsy in breast cancer. J Qilu Oncol, 7, 217–53.
- Morton, D. L., Wen, D. R., Wong, J. H., Economou, J. S., Cagle, L. A., and Storm, F. K. 1992. Technical details of intraoperative lymphatic mapping for early stage melanoma. Arch Surg, 127, 392–9.
- Noguchi, M., Tsugawa, K., and Bando, E. (1999). Sentinel lymphadenectomy in breast cancer: identifcation of sentinel lymph node and detection of metastases. Breast Cancer Res Tread, 53, 97–104.
- Reitsamer, R., Peintinger, F., Prokop, E., Menzel, C., Cimpoca, W., and Rettenbacher, L. (2003). Sentinel lymph node biopsy alone without axillary lymph node dissection—follow up of sentinel lymph node negative breast cancer patients. Eur J Surg Oncol, 29, 221–3.
- Schulman, J. H., Stoeckenius, W., and Prince, L. M. (1959). Mechanism of formation and structure of micro emulsions by electron microscopy. J Phys Chem, 63, 1677–80.
- Steffen, C. (2007). The dilemma of approving antidotes. Toxicology, 233, 13–19.
- Sun, P., Borowitz, J. L., Kanthasamy, A. G., Kane, M. D., Gunasekar, P. G., and Isom, G. E. (1995). Antagonism of cyanide toxicity by isosorbide dinitrate: possible role of nitric oxide. Toxicology, 104, 105–11.
- Tang, G. H., Li, H. J., and Sun, S. J. (1996). The clinical application of methylene blue and its compound preparation. Shangdong Pharm Ind, 2, 42.
- Tedajo, G. M., Bouttier, S., Fourniat, J., Grossiord, J. L., Marty, J. P., and Seiller, M. (2005). Release of antiseptics from the aqueous compartments of a w/o/w multiple emulsion. Int J Pharm, 288, 63–72.
- Yoshimura, K., and Nunomura, M. (1996). Evaluation of endoscopic pibarubicin-lipiodol emulsion injection therapy for gastric cancer. Jpn J Cancer Chemother, 23, 1519–22.
- Yuan, Y. X., Lu, C. Y., Shen, Y. K., and Sun, P. R. (2000). The clinical application of identification and biopsy of sentinel lymph node in breast cancer. J Qilu Oncol, 7, 619–21.