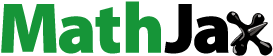
Abstract
We describe a biodegradable amphiphilic polyurethane (PU) with disulfide bonds in the main chain [PEtOz-b-PU(SS)-b-PEtOz]. This multi-block PU was synthesized using poly (ε-caprolactone) diol (PCL-SS-PCL) and poly (2-ethyl-2-oxazoline) (PEtOz-OH) as soft segments, and bis (2-isocyanatoethyl) disulfide as the hard segment. Acid-sensitive PEtOz-OH was used as a hydrophilic segment for pH sensitivity. And reduction sensitivity was induced via disulfide bonds incorporated into the hydrophobic poly (ε-caprolactone) segment of the amphiphilic PUs. The system can self-assemble to form micelles responsive to pH and reducing conditions. The properties of the micelle were studied with dynamic light scattering and scanning electron microscopy. Doxorubicin (DOX) was chosen as a model drug. The in vitro release studies showed that PEtOz-b-PU(SS)-b-PEtOz micelle could degrade more rapidly and completely in a reductive and acidic environment [10 mM dl-Dithiothreitol, pH 5.0]. The methyl tetrazolium (MTT) assay and fluorescent microscopy confirmed the cytotoxicity of the DOX-loaded micelles. This work provides a promising dual-responsive drug carrier based on amphiphilic PU to achieve efficient drug delivery.
Introduction
Anticancer drug delivery systems such as liposomes, micelles, or nanoparticles can overcome some limitations of anticancer drugs including poor solubility in water, high toxicity to normal cells, poor stability in circulation, etc. Although such drug carriers have good biocompatibility, drugs loaded into carrier can be released very slowly. This can result in poor outcomes. Recently, researchers have exploited the features of cancer to design stimuli-responsive nanocarriers for targeted release including in response to reducing conditions or low pH. Drug delivery systems with disulfide (–SS–) bridges can release drugs under reducing conditions (Meng et al., Citation2009; Huo et al., Citation2014), and pH-response systems could be constructed by introducing segments that are unstable under acidic conditions (De Leo et al., Citation2018; Z. Wang et al., Citation2018). However, single responsive systems have had limited success (Chen et al., Citation2015) with incomplete release of drug. Thus, polymer nanocarriers sensitive to multiple stimuli might solve this problem (Guan et al., Citation2017; John et al., Citation2017).
Polyurethanes (PUs) are an important multi-function material that were first synthesized and commercialized in 1930 (Guelcher, Citation2008). Since then, PU materials were successfully incorporated into biomaterials. PUs have been widely used in the manufacture of cardiac valves, catheters, artificial blood vessels, artificial limbs, and other implantable devices (Ren et al., Citation2016; Fu et al., Citation2017). Biodegradable PUs have also been used for drug delivery and tissue engineering (Zhang et al., Citation2012; Sulistio et al., Citation2017). PU offers excellent biocompatibility, perfect biodegradability (Feng et al., Citation2017; Taraghi et al., Citation2017), simple synthetic processing, and multifunctional blocks (Ding et al., Citation2013; Chen et al., Citation2015; Han et al., Citation2015). These advantages have caused PU materials to be useful in drug delivery (Cheng et al., Citation2011; He et al., Citation2016; Yao et al., Citation2016).
Drug-loaded PU micelles have been designed to response to pH (Kumari et al., Citation2010) or reducing conditions (H. Kim et al., Citation2011; Shao et al., Citation2013), and enzyme (Cerritelli et al., Citation2007) environment of tumor cells (Kausar, Citation2017). Lu et al. (Citation2015) reported multiblock PU micelles with redox reactivity via the introduction of disulfide bonds on PU main chain. These drug-loaded micelles released 74% of the drug in the presence of 10 mM GSH within 12 h. However, nearly 30% of the drug was not released from this micelle. Incomplete release of drug from the polymer micelle is common in other reports (Ding et al., Citation2012).
He et al. (Citation2014) reported reduction-sensitive multi-block PU micelles with different amounts of disulfide linkages in the polymer backbone. The amount of disulfide bonds was controlled by the excellent molecular tunability of PUs with paclitaxel (PTX) as the model anticancer drug. The PTX-loaded micelle could only release 60% of PTX under reducing conditions within 48 h. Li et al. (Citation2015) optimized the release profiles of redox-sensitive PU based micelles by incorporating different amount of disulfide linkages in hard segment of polymer. Polymers with the largest number of disulfide bonds only released 72% of Doxorubicin (DOX) after 96 h making the drug release process slow and incomplete (John et al., Citation2015; Yao, et al., Citation2016). This limits the efficacy or could lead to drug resistance (Perez-Herrero & Fernandez-Medarde, Citation2015).
Dual responsive and shell-detachable micelles have been developed to release the cargo completely and rapidly (Chen et al., Citation2017; John et al., Citation2017). The pH- and redox-active carriers can avoid the accumulation of hydrophobic cores that often leads to incomplete release of drugs in hydrophobic aggregates (Guan et al., Citation2017; John et al., Citation2017). Shell-detachable micelles with a disulfide-linked hydrophilic shell and a hydrophobic core were self-assembled via disulfide-linked biodegradable multi-block copolymers. Thus, shell-detachable micelles could detach from the shell material under reducing environments resulting in rapid drug release with destruction of the micellar structure. For example, Wang et al. (Citation2013) reported a novel micelle with detachable PEG corona: the PEG corona of the micelle was detached due to the cleavage of disulfide bonds in the intracellular environment followed by collapse of the micelle. The micelle drastically accelerated drug release at the cytoplasmic GSH level inhibiting the growth of HeLa cells.
Here, we develop a novel shell-detachable micelle with pH- and redox-sensitivity based on an amphiphilic triblock polymer polyoxazoline-block-polyurethane-block-polyoxazoline [PEtOz-b-PU(SS)-b-PEtOz] for delivery of antitumor drugs to C6 cells (Scheme 1). The triblock amphiphilic polymer PEtOz-b-PU(SS)-b-PEtOz was easily synthesized from poly(caprolactone) diol containing disulfide bonds in the main chain (PCL-SS-PCL), bis (2-isocyanatoethyl) disulfide (CDI), and polyoxazoline with hydroxyl end groups (PEtOz-OH). PEOz-OH is a pH-sensitive polymer with good biocompatibility that can be protonated at the pH of the endo/lysosome (Li et al., Citation2015; X. Wang et al., Citation2011). Thus, PEtOz-b-PU(SS)-b-PEtOz micelles can quickly escape from endo/lysosomes. The disulfide bonds in the backbone of the PU were formed via the condensation reaction of PCL-SS-PCL and CDI created sensitivity to high levels of GSH in the cytoplasm. These disulfides also affect the degradation speed of the nanoassembly (Yin et al., Citation2016; Zhang et al., Citation2018), and we also synthesized another triblock polymer PEtOz-b-PU-b-PEtOz from PCL-SS-PCL, 1,6-diisocyanatohexane (HDI) and PEtOz-OH: This system has a similar structure but fewer disulfide bonds in the backbone; it serves as a control system.
Experimental
Materials
ε-caprolactone (ε-CL, 99%) and Stannous octoate [Sn (Oct)2] were obtained from Alfa Aesar (Shanghai, China). CDI and HDI were obtained from Energy-Chemical (Shanghai, China). 2-Ethyl-2-oxazoline (EtOz-OH, 99%) were purchased from Alfa Aesar (Shanghai, China), and distilled prior to use. N,N-dimethylformamide (DMF), dichloromethane, and toluene was purchased from Sinopharm Chemical Reagent Co. Ltd. (Shanghai, China). 1, 4-dithio-d, l-threitol (DTT) was obtained from Merck (Shanghai, China). Doxorubicin hydrochloride (DOX⋅HCl) was obtained from Beijing ZhongShuo Pharmaceutical Technology Development Co., Ltd. (Beijing, China). While the Roswell Park Memorial Institute medium (RPMI-1640, Thermo Fisher Scientific), fetal bovine serum (FBS, Gibco, Australia), and 96-well plates were obtained from Corning Costar (Shanghai, China). 3-(4, 5-dimethylthiazolyl-2-yl)-2, 5-diphenyltetrazolium bromide (MTT) was purchased from Biosharp (Hefei, China). 4′, 6-diamidino-2-phenylindole (DAPI) was purchased from Roche (Shanghai, China). All other chemicals were used as received.
Characterization
The 1H NMR spectra were recorded on a Bruker 400 MHz (Bruker, Billerica, MA, USA) apparatus using deuterated chloroform (CDCl3) as the solvent. The molecular weight and polydispersity of copolymers were determined by a PL GPC 50 instrument (Agilent, Santa Barbara, CA, USA) equipped with Jordi GPC columns (10E4, 2M) following a differential refractive-index detector (PL-RI). The measurements were performed using DMF as the eluent at a flow rate of 1 mL/min at 50 °C and a series of narrow polystyrene standards for the calibration of the columns. The size and zeta-potential of micelles were measured with a Nano-ZS from Malvern Instruments. The morphologies of PEtOz-b-PU(SS)-b-PEtOz micelles and PEtOz-b-PU(SS)-b-PEtOz micelles were performed by scanning electron microscope (SEM) SU-8010 (Hitachi, Tokyo, Japan). The cellular uptake and intracellular release behaviors of DOX-loaded micelles were observed by fluorescence microscope (Nikon eclipse Ts2R, Tokyo, Japan). OD values for each well in the assay were measured using a Synergy H4 hybrid multi-well plate reader (BioTek, Winooski, VT, USA) in MTT experiments. The fluorescence of DOX was measured by F-4500 FL Spectrophotometer (Hitachi,Tokyo, Japan) at 298 K.
Synthesis of PEtOz-b-PU(SS)-b-PEtOz and PEtOz-b-PU-b-PEtOz
The amphiphilic polymer of PEtOz-b-PU(SS)-b-PEtOz and PEtOz-b-PU-b-PEtOz were synthesized from the PEtOz-OH, PCL-SS-PCL, and CDI or HDI (). The PCL-SS-PCL and PEtOz-OH copolymers were synthesized using a similar method as reported (Lee et al., Citation1999; Kim et al., Citation2000). PEtOz-b-PU(SS)-b-PEtOz was synthesized as following routes. First, PCL-SS-PCL (3.00 g, 3.00 mmol) was dissolved in 25 mL DMF under a dry nitrogen atmosphere, then CDI (0.67 g, 3.30 mmol) was added into the DMF solution of PCL-SS-PCL in the presence of 1% Sn (Oct)2 (36 mg) at 65 °C with stirring. And this solution was reacted at 65 °C for 24 h. After that, the polymer solution was cooled to room temperature and a solution of PEtOz-OH (3.00 g, 0.60 mmol) in 15 mL DMF was slowly dropped into the above solution under ice-cooling. Then the mixture was stirring for 48 h at 25 °C. The resulting product was obtained by twice precipitation from mixture of ether and methanol (V:V/10:1) to remove the unreacted PEtOz-OH. The polymer was harvested and dried at r.t. under reduced pressure for 24 h to get the final product. Yield: 63.4%.
Figure 1. (a) Synthesis route of PEtOz-b-PU (SS)-b-PEtOz. (b) Synthesis route of PEtOz-b-PU-b-PEtOz.
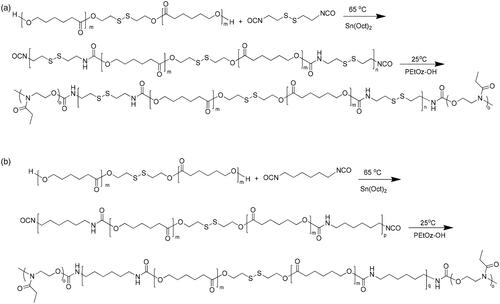
The copolymer PEtOz-b-PU-b-PEtOz was synthesized similarly as PEtOz-b-PU(SS)-b-PEtOz with a yield of 52.1%.
Preparation and characterization of PEtOz-b-PU (SS)-b-PEtOz and PEtOz-b-PU-b-PEtOz micelle
Dialysis method was used to prepare corresponding micelles of polymer PEtOz-b-PU(SS)-b-PEtOz and PEtOz-b-PU-b-PEtOz. Briefly, polymer (2 mg) was completely dissolved in 1 mL DMSO. Then, 1.5 mL water was slowly added into the solution under vigorous stirring. Subsequently, the mixture was transferred into a dialysis bag (MWCO 3500 Da) and dialyzed against water for 24 h.
The size and zeta-potential of micelles were measured with a Nano-ZS from Malvern Instruments. The morphologies of PEtOz-b-PU(SS)-b-PEtOz micelles and PEtOz-b-PU-b-PEtOz micelles were prepared by dropping 10 uL of micelle (0.1 mg/mL) on monocrystalline silicon, and then dried at room temperature.
The critical micelle concentration (CMC) of PEtOz-b-PU (SS)-b-PEtOz micelles was determined by fluorescence spectroscopy using pyrene as fluorescent probe. The concentration of polymer was varied from 1.0 × 10−4 to 0.1 g/L and the final pyrene concentration was fixed at 0.6 μM. The fluorescence of micelle solution was measured by fluorescence spectrophotometer at wavelength range from 340 to 500 nm with the excitation wavelength of 330 nm.
Stability of PEtOz-b-PU(SS)-b-PEtOz micelle in different condition
The stability of PEtOz-b-PU(SS)-b-PEtOz micelles was determined by the dynamic light scattering (DLS) measurement at 25 °C. We assessed the degradation behavior of PEtOz-b-PU(SS)-b-PEtOz micelles by monitoring the size change of micelle under reductive and acidic environment (mimic intracellular environment). Briefly, micellar solution in acetate buffer with DTT (10 mM, pH 5.0) under N2 atmosphere. The solution was stirred by shaker at 37 °C for 24 h. During this period, we measured the size change of PEtOz-b-PU(SS)-b-PEtOz micelle. In this experiment, we used micelle in different media, such as phosphate buffer (PB; 10 mM, pH 7.4), PB (10 mM DTT, pH 7.4), or acetate buffer (10 mM, pH 5.0) as control.
Drug loading content (DLC) and drug loading efficiency (DLE) of DOX
The DOX-loaded PU micelles were prepared by dialysis method. Before loading DOX to the PU micelle, DOX·HCl (2 mg, 0.0034 mmol) was taken off HCl by triethylamine (TEA, 0.99 mL, 6.8 mmol) in DMSO (0.4 mL) overnight in the dark. Then, PEtOz-b-PU(SS)-b-PEtOz or PEtOz-b-PU-b-PEtOz (5 mg) and DOX (Theoretical DLC = 10, 20 wt.%) was completely dissolved in 2 mL DMSO. Then, 3 mL of water was slowly added into the resulting solution under vigorous stirring in the dark. Subsequently, the micelle solution was transferred to the dialysis bag (MWCO, 3500 Da) and dialyzed against distilled water for 24 h to remove the unloaded DOX and DMSO. DLC was determined by fluorescence measurement (excitation at 480 nm). For determination of DLC, DOX-loaded micelles were lyophilized and dissolved in DMSO and analyzed with fluorescence spectroscopy, wherein calibration curve was obtained with DOX/DMSO solutions with different DOX concentration. DLC and DLE were calculated according to the following formula.
In vitro release of DOX
The drug release behaviors of DOX-loaded micelles were investigated in a dialysis bag (MWCO 12,000 Da) which was stirred by shaker at 37 °C. The DOX-loaded micelle was released in four different media, that is, acetate buffer (10 mM, pH 5.0) with 10 mM DTT, acetate buffer (10 mM, pH 5.0), PB (10 mM, pH 7.4) with 10 mM DTT or PB (10 mM, pH 7.4). In order to acquire the sink conditions, drug release studies were performed at a low DLC of 2.5 wt. % and with 0.5 mL of micelle against 25 mL dialysis of appropriate medium. At desired time intervals, 9.0 mL of the release media was taken out and refreshed with an equal volume of fresh medium. The amount of DOX released was measured by fluorescence measurements (excitation at 488 nm, emission at 560 nm).
Cell viability assay
The in vitro cytotoxicity of PEtOz-b-PU(SS)-b-PEtOz and PEtOz-b-PU-b-PEtOz micelles were evaluated by MTT assay using C6 cells. The C6 cells were plated in a 96-well plate (5 × 103 cells/well) in RPMI 1640 media supplemented with 10% FBS, 1% l-glutamine, antibiotics penicillin (100 IU/mL) and streptomycin (100 μg/mL). After 24 h, the medium was removed and replaced by 80 μL of fresh medium and 20 μL of blank or drug-loaded PU micelle was added to make final micelle concentrations of 0.1, 0.2, 0.4, 0.8, and 1.0 mg/mL. Then we incubated the cell at 37 °C with 5% CO2 for 48 h. At last, the medium in 96-well plate was replaced by 100 μL of fresh medium and 10 μL of MTT solution (5 mg/mL) was added. After 4 h, we aspirated medium and dissolved MTT-formazan in 150 μL of DMSO. The relative cell viability (%) was determined by comparing the absorbance at 490 nm with control wells.
The in vitro cytotoxicity of drug loaded PEtOz-b-PU(SS)-b-PEtOz and PEtOz-b-PU-b-PEtOz micelles were evaluated by the similar method as PEtOz-b-PU(SS)-b-PEtOz and PEtOz-b-PU-b-PEtOz micelles. And the final concentration of DOX in the well was varied from 0.01 μg/mL to 40 μg/mL.
Cellular uptake and intracellular release
The cellular uptake of micelle was observed by fluorescent inverted microscope (Nikon eclipse Ts2R) in C6 cells. DOX (red fluorescence) was loaded by PU micelles. The red fluorescence of DOX could label the nanocarriers and track the internalization and intracellular localization of DOX in C6 cells. The nuclei of C6 cells were stained by DAPI, which presented blue fluorescence to showed localization of nuclei. Briefly, C6 cells were plated in a confocal dish (8 × 104 cells/well) in 1 mL RPMI 1640 media supplemented with 10% FBS, 1% l-glutamine and antibiotics penicillin (100 IU/mL) and streptomycin (100 μg/mL) for 24 h. The media were aspirated and replaced by 1 mL of fresh medium. Fifty microliters of DOX-loaded micelle or free DOX·HCl (10.0 μg/mL) was added. The cells were incubated at 37 °C for 2 h or 4 h with DOX-loaded micelle or free DOX·HCl in a humidified 5% CO2 atmosphere. The culture medium was removed and the cells were washed three times with phosphate buffered saline (PBS, pH 7.4, 10 mM). The cells were fixed with 4% paraformaldehyde for 20 min and washed three times with PBS. Then cells were stained with DAPI for 20 min and washed with PBS for three times. At last, we used fluorescent inverted microscope (Nikon eclipse Ts2R) to obtain fluorescence images of cells.
Results and discussion
Characterization of PU copolymers
PEtOz-b-PU(SS)-b-PEtOz and PEtOz-b-PU-b-PEtOz were conveniently synthesized via the condensation reaction of poly (ε-caprolactone) diol (PCL-SS-PCL) and diisocyanate (CDI or HDI), which was subsequently reacted with PEtOz-OH. Finally, we washed and repeated precipitation in the mixture of ether and methanol (V:V/10:1) to remove the unreacted PEtOz-OH. The molecular weight of the polymers was calculated via 1H NMR. The GPC analyses showed that PEtOz-b-PU(SS)-b-PEtOz had a unimodal distribution with a moderate PDI of 1.19 and Mn value was 2.9 kg/mol (Table S1). Similar as PEtOz-b-PU(SS)-b-PEtOz, PEtOz-b-PU-b-PEtOz also had a narrower distribution with a PDI of 1.17 and a Mn value was 2.4 kg/mol (Table S1).
The 1HNMR spectrum also showed that besides signals attribute to PEtOz [1.111 (s), 2.368 (d), 3.444 (s)] and PCL [1.383 (s), 1.674 (s), 2.368 (d), 4.355 (t)], there were also resonances from CDI at 2.829 (s) and 2.905–2.978 (m) (). These results suggest successful synthesis of amphiphilic polymer of PEtOz-b-PU(SS)-b-PEtOz. The 1H NMR spectra of PEtOz-b-PU-b-PEtOz showed signals attributable to PEtOz [1.111 (s), 2.368 (d), 3.444 (s)] and PCL [1.383 (s), 1.674 (s), 2.368 (d), 4.327 (t)] as well as resonances attributable to HDI at 3.166 (s), 2.905–2.978 (m) (). End group analysis of 1H NMR showed that the Mn value of PEtOz-b-PU(SS)-b-PEtOz is 21.8 kg/mol (Table S1). The difference between Mn of GPC and Mn of 1H NMR is due to the fact that the PUs we synthesized have an obviously different structure from the polystyrenes used as GPC calibration samples (Figure S1).
Characterization of PU micelles
We used DLS and SEM to monitor the size and surface charge of the PU micelles. Both micelles showed modest negative surface charges (−32.3 mV for PEtOz-b-PU-b-PEtOz and −31.8 mV for PEtOz-b-PU(SS)-b-PEtOz, respectively; ). The modest negative surface charges of these micelles facilitate longer blood circulation in vivo. The PU micelles had lower average diameters of approximate 152.1 nm for PEtOz-b-PU-b-PEtOz and 109.9 nm for PEtOz-b-PU(SS)-b-PEtOz, respectively (Figure S2(a)). Smaller size of micelle was observed in SEM images (Figure S2(b,c)). The size of the micelle in SEM is less than that of DLS because the micelle shrinked after drying, while the DLS measured in the aqueous solution (Wang et al., Citation2011; Li et al., Citation2017). The smaller size of the PU micelles means that these micelles could be easily uptaken by cells. The CMC of these micelles was determined via fluorescence spectroscopy using pyrene as a fluorescence probe. The concentration of micelle varied from 1.0 × 10−4 to 0.1 g/L, and the concentration of pyrene was fixed at 0.6 μM. The fluorescence spectra of the micelles were measured at an excitation wavelength of 330 nm. The CMC was obtained as the cross-point by extrapolating the intensity ratio I372/I383 (I1/I3) at low and high concentration regions (Figure S3). We then got a lower CMC of 2.14 mg/L from the micelles based on PEtOz-b-PU-b-PEtOz, and 1.99 mg/L from micelles based on PEtOz-b-PU(SS)-b-PEtOz in PB (10 mM, pH 7.4) (). These lower CMC values indicate that these polymers can form micelles at very low concentrations. Therefore, the micelles are stable even upon dilution in body fluids after in vivo administration.
Table 1. Characterization of PEtOz-b-PU-b-PEtOz micelles and PEtOz-b-PU(SS)-b-PEtOz micelles.
The stability of PEtOz-b-PU(SS)-b-PEtOz micelles in reductive and/or acidic condition was also studied by DLS in PB (10 mM, pH 7.4) at 37 °C. Notably, the size of PEtOz-b-PU(SS)-b-PEtOz micelles did not change much within 24 h in PB (10 mM, pH 7.4) indicating that PEtOz-b-PU(SS)-b-PEtOz micelles have adequate stability in body fluids environment. This guarantees the stability of drug-loaded micelles in blood; however, the size of PEtOz-b-PU(SS)-b-PEtOz micelles obviously changed under reducing conditions (10 mM DTT) or acidic environment (pH 5.0) (Figure S4(a,b)). As we can see in Figure S4, the PEtOz-b-PU(SS)-b-PEtOz micelles swelled to over 500 nm in 4 h under a reducing environment (10 mM DTT). Figure S4(c) shows that PEtOz-b-PU (SS)-b-PEtOz micelles swelled to over 400 nm in 4 h and split from a single peak into multiple peaks in a few hours under reducing and acidic environments (10 mM DTT, pH 5.0). In contrast, the PEtOz-b-PU-b-PEtOz micelles did not change as much as PEtOz-b-PU-b-PEtOz micelles under the same condition (Figure S4(d)). These results confirm that PEtOz-b-PU(SS)-b-PEtOz micelles could respond to either reductive or acidic intracellular conditions more quickly than PEtOz-b-PU-b-PEtOz micelles. This is because the PEtOz-b-PU(SS)-b-PEtOz micelles could not only swell in acidic conditions but also could detach from the outer shell of PEtOz in reducing environments. These findings also verified that the PEtOz-b-PU (SS)-b-PEtOz micelle and PEtOz-b-PU-b-PEtOz micelle can degrade rapidly in the intracellular environment of cancer cells.
In vitro release of DOX
DOX was selected as a model hydrophobic anticancer drug to evaluate the drug-loading efficiency of the obtained PU micelles. The DLC and DLE were calculated according to the standard DOX curve. Table S2 shows the drug-loading efficiency of PEtOz-b-PU(SS)-b-PEtOz micelles decreased from 52% to 43% with the drug-loading capacity increasing from 5.2% to 8.7%. In contrast to blank micelles of PEtOz-b-PU(SS)-b-PEtOz, the size of the DOX-loaded PEtOz-b-PU(SS)-b-PEtOz micelles increased by 14–90 nm, and the distribution became slightly wider. The drug-loaded micelles of this size can be easily phagocytosed by cancer cells to improve transport of DOX into cancer cells.
The drug release behavior of the DOX-loaded PU micelles was investigated at 37 °C in PB (pH 7.4) or acetate buffer (pH 5.0) with or without 10 mM DTT. shows that 76% DOX was quickly released from DOX-loaded PEtOz-b-PU(SS)-b-PEtOz micelles in acetate buffer (pH 5.0, 10 mM DTT) within 4 h, and 95% of DOX was released in 24 h. However, in acetate buffer (pH 5.0) without DTT, only 49% of the drug was released within 4 h and 78% of DOX was released in 24 h. At 10 mM DTT with PB (pH 7.4), 62% was released in 4 h and 83% DOX in 24 h. In addition, in PB (pH 7.4) without DTT, DOX-loaded PEtOz-b-PU(SS)-b-PEtOz micelles only released 38% DOX in 4 h and 52% DOX within 24 h. For DOX-loaded PEtOz-b-PU-b-PEtOz micelles, only 65% of the drug was released at 10 mM DTT with PB (pH 7.4) within 24 h. We also noticed that the burst release of DOX-loaded PEtOz-b-PU(SS)-b-PEtOz micelles was nearly 40%. The possible reason may be that, hydrophilic shell of our assembled micelles consists of PEtOz, the PEtOz shell may has some hydrophobicity under the physiological conditions for its pKa was lower than 7.4. It might result in some drugs adsorption to the PEtOz shell during drug loading. Then during the swelling process of the PEtOz shell, these drugs are released. These reasons led to the drug premature release for PEtOz-b-PU(SS)-b-PEtOz drug-loaded micelles. We also observed the similar phenomenon in following references, in these references, micelles were prepared from polymers with similar structure of PEtOz hydrophilic segment (Gao et al., Citation2015; Li et al., Citation2015).
Figure 3. In vitro DOX release profile of DOX-loaded PEtOz-b-PU(SS)-b-PEtOz micelles at pH 7.4 and 5.0 in the presence and absence of 10 mM DTT at 37 °C for 24 h. DOX-loaded PEtOz-b-PU-b-PEtOz micelles was used as control. Error bars represent standard deviation (SD) for n = 3.
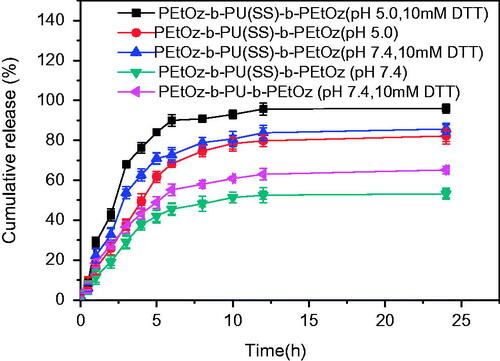
Versus prior work, the cumulative drug release of the DOX-loaded PEtOz-b-PU(SS)-b-PEtOz micelles was increased by ca. 20% in 24 h at pH 5.0 in the presence of DTT. The enhanced DOX release is due to the structure of the PU micelle: The disulfide bonds of the PU micelles can rapidly break under reducing conditions, and PEtOz can quickly swell under acidic environments. These features give PEtOz-b-PU(SS)-b-PEtOz micelles excellent drug release into cancer cells.
Cell viability assay
The in vitro cytotoxicity of PEtOz-b-PU(SS)-b-PEtOz and PEtOz-b-PU-b-PEtOz micelles were evaluated with an MTT assay using C6 cells. The results revealed that PEtOz-b-PU(SS)-b-PEtOz and PEtOz-b-PU-b-PEtOz micelles had no significant toxic effect on C6 cells even at a high concentration of 1.0 mg/mL for 48 h (). This phenomenon suggested that PEtOz-b-PU(SS)-b-PEtOz and PEtOz-b-PU-b-PEtOz micelles have excellent biocompatibility. In contrast, DOX-loaded PEtOz-b-PU(SS)-b-PEtOz and PEtOz-b-PU-b-PEtOz micelles had toxicity on C6 cells. The half-maximal inhibitory concentration (IC50) of DOX-loaded PEtOz-b-PU(SS)-b-PEtOz micelles was 18.1 μg DOX equiv./mL in C6 cells; the IC50 of DOX-loaded PEtOz-b-PU-b-PEtOz micelles was 39.7 μg DOX equiv./mL in C6 cells (). However, the IC50 of free DOX•HCl was only 3.5 μg/mL. The IC50 value of DOX-loaded micelles was higher than free DOX•HCl because of the different drug efficacy of micelles and inefficient cellular uptake.
Figure 4. (a) Cytotoxicity of PEtOz-b-PU (SS)-b-PEtOz and PEtOz-b-PU-b-PEtOz micelles, against C6 cells after 24 h incubation using MTT assay. All data represent mean ± SD (n = 6). (b) Antitumor activities of DOX-loaded PEtOz-b-PU (SS)-b-PEtOz micelles in C6 cells. DOX-loaded PEtOz-b-PU-b-PEtOz micelles were used as controls. The cells were treated with DOX-loaded micelles or free DOX for 48 h. Data are presented as mean ± SD (n = 6).
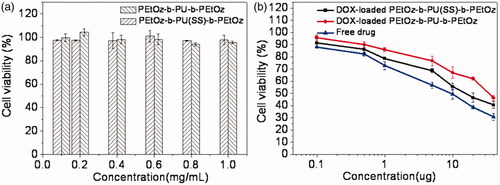
DOX-loaded PEtOz-b-PU (SS)-b-PEtOz micelles were more toxic than DOX-loaded PEtOz-b-PU-b-PEtOz micelles against C6 cells. This is because the DOX-loaded PEtOz-b-PU(SS)-b-PEtOz micelle could release the loaded drug more thoroughly than the DOX-loaded PEtOz-b-PU-b-PEtOz micelle – these micelles were prepared from two amphiphilic polymers with different numbers and locations of disulfide bonds, which were cleaved in an intracellular reducing environment. The in vitro cytotoxicity also confirms that the PEtOz-b-PU(SS)-b-PEtOz micelle could deliver more drug to tumor cells. The PEtOz-b-PU(SS)-b-PEtOz has more disulfide bonds than PEtOz-b-PU-b-PEtOz, but PEtOz-b-PU(SS)-b-PEtOz has disulfide bonds between the hydrophilic segment and hydrophobic segment. This disulfide increases release. Such micelles could release loaded drug more rapidly and completely. In contrast, the PEtOz-b-PU-b-PEtOz micelle with disulfide bonds only in the hydrophobic segment had a weaker ability to release encapsulated drug and thus displayed weaker toxicity to tumor cells.
Cellular uptake and intracellular release
The cellular uptake of the drug-loaded PEtOz-b-PU(SS)-b-PEtOz and PEtOz-b-PU-b-PEtOz micelles were observed with fluorescent microscopy (Nikon eclipse Ts2R) in C6 cells (). The cells incubated with DOX-loaded PEtOz-b-PU(SS)-b-PEtOz micelles showed DOX fluorescence in the cytoplasm and nucleus after 2 h or 4 h of incubation. In contrast, cells with DOX-loaded PEtOz-b-PU-b-PEtOz micelles had weaker DOX fluorescence. This further confirms that DOX-loaded PEtOz-b-PU(SS)-b-PEtOz micelles release the loaded drug more thoroughly than DOX-loaded PEtOz-b-PU-b-PEtOz micelles. The system with disulfide bonds between hydrophilic and hydrophobic segments can form shell-detachable micelles that easily break down in the intracellular reductive environment resulting in more complete drug release. Due to inefficient cellular uptake at predetermined incubation times, we observed lower DOX fluorescence for cells cultured with DOX-loaded micelles than those with free DOX. Therefore, we concluded that DOX-loaded PEtOz-b-PU(SS)-b-PEtOz micelles have excellent and efficient ability to release DOX via pH and redox effects.
Figure 5. (a) Fluorescence microscope images of C6 cells incubated with DOX-loaded PEtOz-b-PU(SS)-b-PEtOz micelles, DOX-loaded PEtOz-b-PU-b-PEtOz micelles and free DOX (10 µg/mL) in 2 h. (b) Fluorescence microscope images of C6 cells incubated with DOX-loaded PEtOz-b-PU(SS)-b-PEtOz micelles, DOX-loaded PEtOz-b-PU-b-PEtOz micelles and free DOX (10 µg/mL) in 4 h. For each panel, images from left to right show DOX fluorescence in cells (red),cell nuclei stained by DAPI (blue) and overlays of two images. The scale bars correspond to 20 µm in all the images.
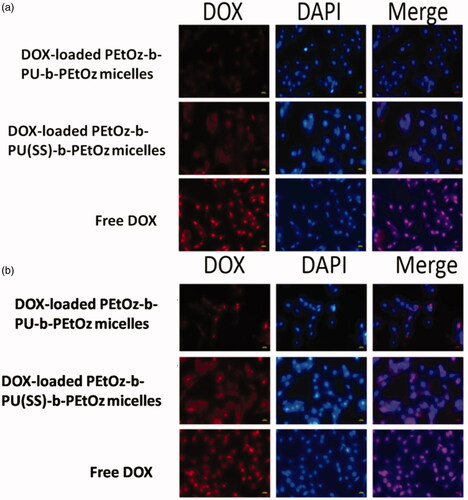
Conclusions
We developed pH- and redox-responsive micelles based on PU. These materials have a different number of disulfide bonds that efficiently deliver and release DOX into C6 cells resulting in superior antitumor activity. The polymer PEtOz-b-PU(SS)-b-PEtOz with disulfide bonds between the hydrophilic and hydrophobic segments had better drug release capabilities. The in vitro cytotoxicity of PEtOz-b-PU(SS)-b-PEtOz micelle showed good biocompatibility, which is important for biomedical applications. The micelles exhibit fast reduction and pH-triggered drug release, and DOX-loaded PEtOz-b-PU(SS)-b-PEtOz micelles significantly enhance DOX cytotoxicity against C6 cells. These dual-responsive biodegradable micelles are promising for glioma treatment, which will be evaluated in future work.
Supporting_Information_-revised_20190202.docx
Download MS Word (1.6 MB)Disclosure statement
There are no conflicts to declare.
Additional information
Funding
References
- Cerritelli S, Velluto D, Hubbell JA. (2007). PEG-SS-PPS: reduction-sensitive disulfide block copolymer vesicles for intracellular drug delivery. Biomacromolecules 8:1966–72.
- Chen GJ, Wang YY, Xie RS, et al. (2017). Tumor-targeted pH/redox dual-sensitive unimolecular nanoparticles for efficient siRNA delivery. J Control Release 259:105–14.
- Chen JJ, Ding JX, Xiao CS, et al. (2015). Emerging antitumor applications of extracellularly reengineered polymeric nanocarriers. Biomater Sci 3:988–1001.
- Cheng YY, Zhao LB, Li YW, et al. (2011). Design of biocompatible dendrimers for cancer diagnosis and therapy: current status and future perspectives. Chem Soc Rev 40:2673–703.
- De Leo V, Milano F, Mancini E, et al. (2018). Encapsulation of curcumin-loaded liposomes for colonic drug delivery in a pH-responsive polymer cluster using a pH-driven and organic solvent-free process. Molecules 23:15.
- Ding MM, Li JH, Tan H, et al. (2012). Self-assembly of biodegradable polyurethanes for controlled delivery applications. Soft Matter 8:5414–28.
- Ding MM, Song NJ, He XL, et al. (2013). Toward the next-generation nanomedicines: design of multifunctional multiblock polyurethanes for effective cancer treatment. Acs Nano 7:1918–28.
- Feng XP, Wang GR, Neumann K, et al. (2017). Synthesis and characterization of biodegradable poly(ether-ester) urethane acrylates for controlled drug release. Mater Sci Eng C Mater Biol Appl 74:270–8.
- Fu SX, Yang GQ, Wang J, et al. (2017). pH-sensitive poly(ortho ester urethanes) copolymers with controlled degradation kinetic: synthesis, characterization, and in vitro evaluation as drug carriers. Eur Polym J 95:275–88.
- Gao Y, Zhang C, Zhou Y, et al. (2015). Endosomal pH-responsive polymer-based dual-ligand-modified micellar nanoparticles for tumor targeted delivery and facilitated intracellular release of paclitaxel. Pharm Res 32:2649–62.
- Guan YY, Su YL, Zhao LL, et al. (2017). Biodegradable polyurethane micelles with pH and reduction responsive properties for intracellular drug delivery. Mater Sci Eng C Mater Biol Appl 75:1221–30.
- Guelcher SA. (2008). Biodegradable polyurethanes: synthesis and applications in regenerative medicine. Tissue Eng Part B Rev 14:3–17.
- Han N, Zhao QF, Wan L, et al. (2015). Hybrid lipid-capped mesoporous silica for stimuli-responsive drug release and overcoming multidrug resistance. ACS Appl Mater Interfaces 7:3342–51.
- He HZ, Ren YR, Wang ZF, et al. (2016). A pH-responsive poly(ether amine) micelle with hollow structure for controllable drug release. RSC Adv 6:91940–8.
- He XL, Ding MM, Li JH, et al. (2014). Biodegradable multiblock polyurethane micelles with tunable reduction-sensitivity for on-demand intracellular drug delivery. RSC Adv 4:24736–46.
- Huo M, Yuan J, Tao L, et al. (2014). Redox-responsive polymers for drug delivery: from molecular design to applications. Polym Chem 5:1519–28.
- John JV, Johnson RP, Heo MS, et al. (2015). Polymer-block-polypeptides and polymer-conjugated hybrid materials as stimuli-responsive nanocarriers for biomedical applications. J Biomed Nanotechnol 11:1–39.
- John JV, Uthaman S, Augustine R, et al. (2017). pH/redox dual stimuli-responsive sheddable nanodaisies for efficient intracellular tumour-triggered drug delivery. J Mater Chem B 5:5027–36.
- Kausar A. (2017). Review on technological significance of photoactive, electroactive, pH-sensitive, water-active, and thermoresponsive polyurethane materials. Polym Plast Technol Eng 56:606–16.
- Kim C, Lee SC, Shin JH, et al. (2000). Amphiphilic diblock copolymers based on poly(2-ethyl-2-oxazoline) and poly(1,3-trimethylene carbonate): synthesis and micellar characteristics. Macromolecules 33:7448–52.
- Kim H, Jeong SM, Park JW. (2011). Electrical switching between vesicles and micelles via redox-responsive self-assembly of amphiphilic rod-coils. J Am Chem Soc 133:5206–9.
- Kumari A, Yadav SK, Yadav SC. (2010). Biodegradable polymeric nanoparticles based drug delivery systems. Colloids Surf B Biointerfaces 75:1–18.
- Lee SC, Chang Y, Yoon JS, et al. (1999). Synthesis and micellar characterization of amphiphilic diblock copolymers based on poly(2-ethyl-2-oxazoline) and aliphatic polyesters. Macromolecules 32:1847–52.
- Li J, Zhou Y, Li C, et al. (2015). Poly(2-ethyl-2-oxazoline)-doxorubicin conjugate-based dual endosomal pH-sensitive micelles with enhanced antitumor efficacy. Bioconjugate Chem 26:110–9.
- Li S, Yi J, Li W, et al. (2017). Synthesis and characterization of three novel amphiphilic dextran self-assembled micelles as potential drug delivery system. J Mater Sci 52:12593–607.
- Lu WT, Wang XX, Cheng R, et al. (2015). Biocompatible and bioreducible micelles fabricated from novel alpha-amino acid-based poly(disulfide urethane)s: design, synthesis and triggered doxorubicin release. Polym Chem 6:6001–10.
- Meng FH, Hennink WE, Zhong Z. (2009). Reduction-sensitive polymers and bioconjugates for biomedical applications. Biomaterials 30:2180–98.
- Perez-Herrero E, Fernandez-Medarde A. (2015). Advanced targeted therapies in cancer: Drug nanocarriers, the future of chemotherapy. Eur J Pharm Biopharm 93:52–79.
- Ren H, Chen D, Shi Y, et al. (2016). Multi-responsive fluorescence of amphiphilic diblock copolymer containing carboxylate azobenzene and N-isopropylacrylamide. Polymer 97:533–42.
- Shao W, Miao K, Liu HH, et al. (2013). Acid and reduction dually cleavable amphiphilic comb-like copolymer micelles for controlled drug delivery. Polym Chem 4:3398–410.
- Sulistio A, Reyes-Ortega F, D'Souza AM, et al. (2017). Precise control of drug loading and release of an NSAID-polymer conjugate for long term osteoarthritis intra-articular drug delivery. J Mater Chem B 5:6221–6.
- Taraghi I, Paszkiewicz S, Grebowicz J, et al. (2017). Nanocomposites of polymeric biomaterials containing carbonate groups: an overview. Macromol Mater Eng 302:22.
- Wang K, Liu Y, Yi WJ, et al. (2013). Novel shell-cross-linked micelles with detachable PEG corona for glutathione-mediated intracellular drug delivery. Soft Matter 9:692–9.
- Wang X, Li X, Li Y, et al. (2011). Synthesis, characterization and biocompatibility of poly(2-ethyl-2-oxazoline)-poly(D,L-lactide)-poly(2-ethyl-2-oxazoline) hydrogels. Acta Biomater 7:4149–59.
- Wang Z, Deng XP, Ding JS, et al. (2018). Mechanisms of drug release in pH-sensitive micelles for tumour targeted drug delivery system: a review. Int J Pharm 535:253–60.
- Wang ZG, Wan PJ, Ding MM, et al. (2011). Synthesis and micellization of new biodegradable phosphorylcholine-capped polyurethane. J Polym Sci A Polym Chem 49:2033–42.
- Yao YC, Xu DQ, Liu C, et al. (2016). Biodegradable pH-sensitive polyurethane micelles with different polyethylene glycol (PEG) locations for anti-cancer drug carrier applications. RSC Adv 6:97684–93.
- Yao YC, Xu H, Liu C, et al. (2016). Biodegradable multi-blocked polyurethane micelles for intracellular drug delivery: the effect of disulfide location on the drug release profile. RSC Adv 6:9082–9.
- Yin TJ, Liu JY, Zhao ZK, et al. (2016). Smart nanoparticles with a detachable outer shell for maximized synergistic antitumor efficacy of therapeutics with varying physicochemical properties. J Control Release 243:54–68.
- Zhang P, Wu JL, Xiao FM, et al. (2018). Disulfide bond based polymeric drug carriers for cancer chemotherapy and relevant redox environments in mammals. Med Res Rev 38:1485–510.
- Zhang Q, Ko NR, Oh JK. (2012). Recent advances in stimuli-responsive degradable block copolymer micelles: synthesis and controlled drug delivery applications. Chem Commun 48:7542–52.