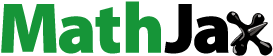
Abstract
Purpose
S-propargyl-cysteine (SPRC), an excellent endogenous hydrogen sulfide (H2S) donor, could elevate H2S levels via the cystathionine γ-lyase (CSE)/H2S pathway both in vitro and in vivo. However, the immediate release of H2S in vivo and daily administration of SPRC potentially limited its clinical use.
Methods
To solve the fore-mentioned problem, in this study, the dendritic mesoporous silica nanoparticles (DMSN) was firstly prepared, and a sustained H2S delivery system consisted of SPRC and DMSN (SPRC@DMSN) was then constructed. Their release profiles, both in vitro and in vivo, were investigated, and their therapeutical effect toward adjuvant-induced arthritis (AIA) rats was also studied.
Results
The spherical morphology of DMSN could be observed under scanning Electron Microscope (SEM), and the transmission electron microscope (TEM) images showed a central-radiational pore channel structure of DMSN. DMSN showed excellent SPRC loading capacity and attaining a sustained releasing ability than SPRC both in vitro and in vivo, and the prolonged SPRC releasing could further promote the release of H2S in a sustained manner through CSE/H2S pathway both in vitro and in vivo. Importantly, the SPRC@DMSN showed promising anti-inflammation effect against AIA in rats was also observed.
Conclusions
A sustained H2S releasing donor consisting of SPRC and DMSN was constructed in this study, and this sustained H2S releasing donor might be of good use for the treatment of AIA.
Introduction
Rheumatoid arthritis (RA) is a chronic complex inflammatory disease, which might cause severe joint swelling, destruction and disability, (Smolen and Steiner Citation2003; Stamp and Cleland Citation2007; McInnes and Schett Citation2011) with subsequent high morbidity and mortality, and it is reported that RA affects about 1% of the world population (Rutherford et al. Citation2017). Although the cause of RA remains unclear for now, the immune mediators (inflammatory cytokines) is thought to induce the joint damage that begins at the synovial membrane (Low et al. Citation2008; Thomas et al. Citation2011; Davignon et al. Citation2013). Recently, various therapeutic agents have been applied for the treatment of RA, such as tofacitinib, methotrexate, monoclonal antibody and glucocorticoids (Chan et al. Citation2007; Bossaller and Rothe Citation2013; Lee et al. Citation2014; Lopez-Olivo et al. Citation2014; Ferreira et al. Citation2016). However, the toxicity adverse effects of these agents are unignorable, somehow limiting their clinical use (Chan et al. Citation2007).
For a long period, hydrogen sulfide (H2S) was considered as toxic compound. However, recently, H2S has been found to play an important function in physiological processes as the third endogenous gasotransmitter together with nitric oxide (NO) and carbon monoxide (CO). Moreover, the functions include neuromodulation, (Eto et al. Citation2002; Chen et al. Citation2007; Hu et al. Citation2010; Davoli et al. Citation2015) protection against cardiovascular system (Papapetropoulos et al. Citation2009; Mustafa et al. Citation2011; Bełtowski and Jamroz-Wis¨niewska Citation2014; Katsouda et al. Citation2016) and regulation of inflammation (Ishibashi Citation2013; Burguera et al. Citation2017).
Due to the significant contributions of H2S in many physiological processes, researchers often use H2S releasing agents (H2S donors) to modulate the H2S levels in vitro and in vivo. Commonly, sodium hydrosulfide (NaHS) and sodium sulfide (Na2S), as exogenous H2S donors, are mostly studied (Streng et al. Citation2008; Lu et al. Citation2010). Whereas, these exogenous H2S donor could release H2S upon addition to water in an uncontrolled and rapid manner, making it hard to precisely maintain H2S level both in vitro and in vivo (Lee, Zhou, et al. Citation2011; Lougiakis et al. Citation2016). To solve this problem, a series of endogenous H2S donor has been proposed, such as diallyl trisulfide (DATS), S-allyl cysteine (SAC), S-propargyl-cysteine (SPRC, also known as ZYZ-802). Our previous studies revealed that SPRC, an endogenous H2S modulator, exerted protective effects against inflammation by elevating the expression of cystathionine γ-lyase (CSE) (Wang et al. Citation2010; Liang et al. Citation2014). Recently, our group reported that SPRC could alleviate inflammatory symptoms in adjuvant-induced arthritis via Nrf2-ARE signaling pathway, which firstly reported the possibility of SPRC for the treatment of RA. However, when using high dosage of SPRC (for example, around 150 mg kg−1 according to rats’ body weight), its instant release of H2S in vivo might bring unacceptable side effects. Herein, how to attain a sustained and controllable H2S release remains a challenging problem for the further development of SPRC. Intended to solve the so-mentioned problems of SPRC, a series delivery system for SPRC has been established. However, the sustained promotion of in vivo H2S still remains a challenge (Huang et al. Citation2013; Tran et al. Citation2015, Citation2019a).
Nanoparticles have been widely used to conduct more specific and efficient treatments for complex diseases, (Kumari et al. Citation2010; Sahni et al. Citation2011; Mieszawska et al. Citation2013) thereinto, the conventional mesoporous silica nanoparticles (CMSN) has proved to be effective treatment for various diseases, (Balas et al. Citation2006; Li et al. Citation2015; Mccarthy et al. Citation2016) and not surprisingly, for inflammatory disease (Lee, Yun, et al. Citation2011; Braz et al. Citation2016). CMSN, with large surface area, pore volume, adjustable pore sizes, good biocompatibility, and easily modified pore surface, has become an ideal drug carrier. However, the clinical use of CMSN is limited partially due to its potential cellular toxicity. Herein, a novel silica-based nanocarriers with dendritic center-radial oriented mesopores (DMSN) was fabricated, (Shen, Yang, et al. Citation2014; Yang et al. Citation2015; Liu et al. Citation2018) and its use for loading of SPRC was investigated in this study.
Here in this passage, we hypothesized that DMSN could effectively load and sustained release SPRC in vitro and in vivo, further, attaining the sustained release of H2S. Adjuvant-induced arthritis (AIA) model in rats was successfully established to mimic RA in rats, and the use of SPRC@DMSN for the alleviation of AIA in rats was also studied. To the best of our knowledge, this is the first report about using DMSN as a delivery system on SPRC.
Material and methods
Chemical materials
SPRC was synthesized as we reported previously (Wang et al. Citation2009). Tetraethyl orthosilicate (TEOS) was purchased from Aladdin Reagent Co., Ltd (Shanghai, China). Cetyltrimethylammonium chloride (CTAC) solution (25 wt % in H2O), 3-aminopropyltriethoxysilane (APTES), triethanolamine (TEA) and decahydronaphthalene (DHA) were all purchased from Sigma Aldrich (St Louis, USA). Complete Freund’s Adjuvant (CFA), propargylglycine (PAG), BMDM medium (Iscove’s modified Dulbecco’s medium supplemented with 10% fetal calf serum, 100 μ mL−1 penicillin, 100 μg mL−1 streptomycin, 10 mM thioglycerol), human macrophage-colony stimulating factor (M-CSF), sodium hydroxide, hydrochloric acid, acetonitrile, methanol, toluene, anhydrous ethanol, sodium sulfide, monobromobimane (MBB), diethylenetriaminepentaacetic acid (DTPA), sulfosalicylic acid and hydrocortisone were purchased from Macklin Industrial Corporation (Shanghai, China). Elisa kit of TNF-α, IL-1β, IL-6 and IL-10 were purchased from MultiSciences (Hangzhou, China).
Synthesis of dendritic mesoporous silica nanoparticle
The DMSN was prepared via one-pot synthesis as reported (Shen, Yang, et al. Citation2014) with little modification. 50 mL CTAC solution (25 wt%) and 0.4 g of TEA were added to 80 mL of deionized water with stirring at 60 °C for 1 h. 40 mL TEOS-DHA solution (20 v/v%) was then dropped into the mixture and the reaction was kept magnetic stirring for another 12 h in 60 °C water bath. The products were centrifugated, and the precipitation was washed with ethanol for several times and calcined at 550 °C to remove the template and residual reactants. FITC-conjugation was done by adding FITC in the synthesis step to create inherently fluorescent DMSN as reported (Huang, Huang, et al. Citation2011; Huang, Li, et al. Citation2011; Luo et al. Citation2011; Desai et al. Citation2016).
Physicochemical characterization
The particle size and ζ potentials of the nanoparticles were measured by Malvern Zetasizer Nano Series (Malvern, USA). The SEM (Nova Nano SEM, Philips, Netherlands) and TEM (JEM2100F High Resolution JEOL, Japan) were used to observe the morphology and pore structure. The nitrogen adsorption-desorption was performed using a Micromeritics Tristar 3000 pore analyzer (Micromeritics, USA) to determine the pore size, pore volume, and specific surface area. Surface area was determined using the Brunauere Emmere Teller (BET) model, and the pore size distributions was calculated from the adsorption branch of the isotherm using the Barrette Joynere Halenda (BJH) model.
SPRC loading into dendritic mesoporous silica nanoparticles
500 mg of prepared DMSN was suspended in different volume (5–20 mL) of SPRC saturated solution (50 mg mL−1), and the mixture kept magnetic stirring for 24 h at 25 °C. The resulting suspensions were centrifugated and the precipitates were collected, washed with distilled water for 3 times. The loading efficiency (LE) and encapsulation efficiency (EE) were determined using Agilent 1200 series HPLC system (Agilent Technologies, Palo Alto, CA). The SPRC loaded DMSN were named as SPRC@DMSN. Each sample was conducted in triplicate, and calculated as the following equation:
In vitro SPRC release study
To study the in vitro releasing ability, 20 mg of SPRC powder and SPRC@DMSN were firstly dispersed in 3 mL of phosphate buffer saline (PBS, pH = 7.4) and then transferred into a dialysis bag (cut off molecular weight 12–14 kDa), which were sealed and immersed in 47 mL of PBS at 37 °C under stirring. At predetermined time intervals, 1 mL of sample solution was taken out and immediately replenished with an equal volume of PBS. The sample solution was centrifugated (10,000 rpm, 10 min) and further analyzed by HPLC.
Macrophages culture
Bone marrow derived monocytes were isolated from healthy mice. Mice were firstly sacrificed and after cut the epiphyses of the bones, further flushing the marrow into a 50 mL centrifuge tube using a 5 mL syringe and a 23 G needle. The cell suspension was then filtered and centrifuged. The supernatant was then discarded and resuspend the cell pellet in 3 mL of BMDM medium. Dilute the cells at a concentration of 3.5 × 105 cells mL−1, then added M-CSF at a final concentration of 25 ng mL−1 and seeded 10 mL per 10 cm cell culture-treated petri dish. Finally, incubated at 37 °C with 5% CO2 for 3 days, and replaced the medium and incubated for another 3 days (Assouvie et al. Citation2018).
Cytotoxicity toward macrophages
Macrophages were seeded into 96-well plates at a density of 1 × 104 per well and incubated in 5% CO2 atmosphere at 37 °C for 24 h. The original medium was then replaced with 200 μL of solutions containing various concentrations of SPRC, and SPRC@DMSN for 48 h incubation. The numbers of living cells were determined using the CCK-8 assay as reported.(Zheng et al. Citation2012) To verify the cellular uptake of DMSN by macrophages, cells were seeded in confocal microscopy 20 mm2 Petri dish for 12 h, then added the FITC-labeled DMSN (10 mg mL−1) to medium and co-incubated for 1, 6, and 12 h followed by PBS washing for three times. The cell nucleus was stained using DAPI.
In vitro H2S release study
Samples of macrophages were transfected with SPRC and SPRC@DMSN (equivalent to 10 μM SPRC) for 48 h. Supernatant was collected at predetermined intervals for the detection of H2S release.
Anti-inflammation in vitro
To investigate whether transfection with supplementations could inhibit the inflammation, LPS-stimulated macrophages were transfected with SPRC and SPRC@DMSN (equivalent to 10 μM SPRC) for 6 h. To prove the anti-inflammation effect was through the release of endogenous H2S, PAG (2 mM) was added to co-incubate with SPRC or SPRC@DMSN.
Animal experiment design
The Animal Care and Use Committee of Municipal Affairs Bureau of Macau approved all studies described herein (approval number AL010/DICV/SIS/2018), and experiment was conducted under the guidance of NIH Guide for the Care and Use of Laboratory Animals (8th edition).
Pharmacokinetics study
Samples of SPRC powder and SPRC@DMSN were dissolved or dispersed in saline for oral administration, each sample contains same amount of SPRC, and dosage were calculated through the weight of rats (100 mg kg−1). The rats’ serum was collected at predetermined time (0, 0.5, 1, 1.5, 2, 3, 6, 12, 24, 48, and 72 h) into heparin sodium tubes and analyzed.
Alleviation of inflammation in rats
The AIA rat model was established via a subcutaneous injection of 100 μL of CFA (10 mg mL−1) at the base of the tail, while control group received 100 μL of saline. Totally 24 of rats were randomly divided into four groups as follows: Control group (n = 6), no intervention; AIA group (n = 6), injection of 100 μL of CFA; SPRC group (n = 6), after injection of 100 μL of CFA, further oral administrated with 2 mL of SPRC solution every 3 days (equivalent to 100 mg kg−1 of SPRC) for 30 days; SPRC@DMSN group (n = 6), after injection of 100 μL of CFA, further oral administrated with 2 mL of SPRC@DMSN suspension every 3 days (equivalent to 100 mg kg−1 of SPRC) for 30 days.
The paw volume was measured by a ugo basile 7140 plethysmometer (Ugo Basile, Gemonio VA, Italy) and body weight was measured at the 0, 5th, 15th, 20th, 25th, and 30th day post the injection of CFA. Arthritis index was scored from 0 to 4 per limb, for 0 = no sign of inflammation, 1–4 = increasing degrees of inflammatory, with a maximum score of 16 per rat (Chuang et al. Citation2019).
At day 30, blood sample was collected from rats in each group, the pro-inflammatory cytokines (TNF-α, IL-1β and IL-6) and anti-inflammatory cytokine (IL-10) levels in serum were measured using ELISA kits according to the manufacturer’s instructions.
Histopathological analysis
Safranin-O staining, which stains proteoglycan in cartilage, was carried out by previously described methods (Ray et al. Citation2004; Chen et al. Citation2020; Shin et al. Citation2020). In brief, dewaxed tissue sections were stained with Weigert’s hematoxylin working solution composed of 1% hematoxylin and 30% ferric chloride (anhydrous) for 10 min. After extensive washing, sections were counter-stained with fast green solution for 5 min. After rinsing with 1% acetic acid, sections were then stained with 0.1% safranin-O solution for another 5 min.
Micro-CT analyses
The hind limb of each rat was harvested after sacrifice and imaged with 3 D microcomputed tomography (micro-CT, Siemens Inveon MM Gantry CT, Germany) at a voltage of 70 kV and an electric current of 400 µA. The exposure time was 800 ms, and the scan area was 26.42 mm × 26.42 mm × 30 mm around the metatarsal bone articulations.
Safety evaluations
The white blood cell (WBC), red blood cell (RBC), and hemoglobin (HGB) were assayed using standard methods (Sysmex KX-21, Japan). Major organs including heart, liver, spleen, lung, and kidney were dissected and fixed with 4% paraformaldehyde and further stained with H&E.
Measurement of H2S concentration
Concentration of H2S was measured as reported with little modification (Zhu et al. Citation2007). Briefly, 15 μL serum or culture medium, 25 μL MBB acetonitrile solution, and 35 μL 0.3% diethylenetriaminepentaacetic acid (DTPA) containing Tris-HCl buffer (pH 9.5) were mixed and incubated in hypoxia incubator for 30 min. Subsequently, 25 μL sulfosalicylic acid was added to stop reaction and then centrifugated at 12,000 rpm for 10 min. Finally, 30 μL supernatant, 267 μL acetonitrile and 3 μL internal standard (hydrocortisone methanol solution) were mixed and analyzed with LC-MS.
Samples were analyzed using an Agilent 1200 series HPLC system (Agilent Technologies, Palo Alto, CA) coupled with an Agilent 6460 Triple Quadrupole (Agilent Technologies, Palo Alto, CA). ZORBAX Eclipse Plus 95 C18, 2.1 × 50 mm, 1.8 μm column was used and temperature was set at 35 °C. The mobile phase consisted of water (A) and acetonitrile (B) and was delivered gradient at 0–0.5 min, 5% B; 0.5–0.6 min, 5–20% B; 0.6–5.0 min, 20–47.5% B; 5.0–5.1 min, 47.5–95% B; 5.1–6.0 min, 95% B, at a flow rate of 0.3 mL min−1. The mass spectrometer was operated in positive ion mode. Scan type was chosen MRM with gas temperature at 325 °C and gas flow at 10 L min−1. Scan time was 500 ms and start-stop mass was 100–1000. The sample injection volume was 5 μL.
Western blot analysis
Western blot analysis was performed as previously described (Pan et al. Citation2011). 50 μg of proteins from cell or tissue were separated and transferred to polyvinyl difluoride membrane, with further blocking with 5% nonfat dried milk. The membranes were then probed with antibodies against CSE and GAPDH and incubated with either horseradish peroxidase-conjugated goat anti-rabbit or anti-mouse antibody. Immunoreactive proteins were visualized by enhanced chemiluminescence and signal intensity was detected and quantified by Alpha Imager (San Leandro, USA).
Statistical analysis
Each experiment was performed at least triplice and the data are presented as the mean ± SD. Statistical significance was determined using one-way analysis of variance (ANOVA) test and p < 0.05 was considered significant unless otherwise indicated.
Results
Characterization of SPRC@DMSN
The spherical morphology of DMSN could be observed under SEM (), while the TEM images showed the central-radiational pore channel structure of the prepared DMSN (). According to the N2 adsorption − desorption isotherms, the prepared DMSN () exhibited a typical type-IV isotherm containing H1-type hysteresis, suggesting the mesoporous structure. Meanwhile, a capillary condensation step around 0.2 < P P0−1 < 0.4 could be observed, which indicated a clear evidence for their narrow pore size. The influence of different amounts of SPRC used on the preparation of SPRC@DMSN was investigated, and the results of particle size, ζ potential, pore size, pore volumes, surface area, loading efficiency (LE), and encapsulation efficiency (EE) of the prepared DMSN and different SPRC@DMSN were listed in . Interestingly, the diameter of DMSN was around 100 nm calculated under TEM while the hydrodynamic diameter for DLS test showed a particle size around 150 nm (), which might be resulted from the aggregate property of nanoparticles in water. (van Grieken et al. Citation2009). Both the pore volume and surface area showed a decreasing tendency before 15 mL of SPRC saturated solution used, indicated a successfully loading of SPRC into DMSN. Though an increase tendency of LE could be observed when the SPRC saturated solution volume increased, a ‘loading capacity peak’ would be observed when the SPRC saturated solution volume went beyond 15 mL, hence above all, the volume was chosen 15 mL for further study, and for a convenient expression, the SPRC@DMSN (15 mL) was named as SPRC@DMSN. The Tyndall effect could be observed in . Moreover, both DMSN and SPRC@DMSN exhibited excellent in vitro stability, with negligible changes of particle size during storage under room temperature (25 °C) for a week (). In the in vitro SPRC releasing experiment (), the SPRC powder showed immediately and complete dissolve within few minutes, while SPRC@DMSN showed prolonged and sustained SPRC release within 96 h, which indicated a sustained releasing ability of SPRC@DMSN.
Figure 1. The morphology study of prepared DMSN and SPRC@DMSN. The SEM of (A) and TEM of (B) DMSN (Scale bar = 50 nm). (C) The typical type-IV isotherm containing H1-type hysteresis indicated the preparation of mesopore structure in DMSN. (D) The Tyndall effect could be observed when DMSN and SPRC@DMSN dispersed in water and (E) the 7-day stability of DMSN and SPRC@DMSN in water. (F) The cumulative release of SPRC in PBS (pH = 7.4) within 96 h (n = 3, mean ± SD).
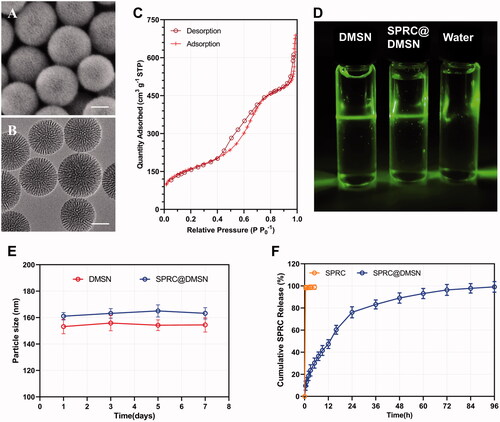
Table 1. The characterization of DMSN and SPRC@DMSN (n = 3, mean ± SD).
Cytotoxicity study
A time-depend high green fluorescence could be observed in macrophages, which indicated the cellular uptake of the FITC labeled DMSN (), and cell viability remained around 95% or higher when the concentration of SPRC changed from 1 to 10 μM in SPRC or SPRC@DMSN groups (). However, the SPRC group showed a decrease of cell viability at concentration of 20 and 40 μM. Interestingly, the SPRC@DMSN group showed negligible cytotoxicity at even high SPRC concentration (equal to 20 and 40 μM SPRC), which revealed the lower cytotoxicity of SPRC@DMSN group.
Supplementations exerted anti-inflammation effects through elevation of H2S level via CSE/H2S pathway in vitro
CSE was an important endogenous H2S producing enzyme, and SPRC was reported to elevate the H2S concentration via CSE/H2S pathway. A significant increase of expression of CSE could be observed in groups treated with SPRC or SPRC@DMSN, while the latter showed a stronger elevating effect. PAG, a CSE inhibitor, was then co-incubated. Not surprisingly, all group showed significant inhibition of CSE expression ().
Figure 3. Supplementations exerted anti-inflammation effects via CSE/H2S pathway in vitro. (A, B) Changes of CSE expression after co-incubation with different supplementations. Significant different compared with control group indicated as (*), and significant different compared with LPS group indicated as (&) (n = 3, mean ± SD). (C) The H2S release in vitro after co-incubation with different supplementations (n = 3, mean ± SD). The pro-inflammatory cytokines levels of (D) TNF-α, (E) IL-1β, (F) IL-6, and anti-inflammatory cytokine level of (G) IL-10 were measured. Significant different compared with LPS treated group indicated as (*) (n = 3, mean ± SD).
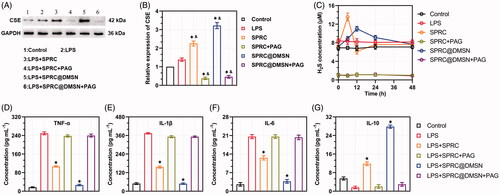
To further prove the change of CSE expression could influence the release of H2S, we then detected the H2S release within 48 h. The control group showed stable H2S concentration around 7.22 μM, while treated with LPS could slightly increase the H2S level to around 8.31 μM as shown in . Meanwhile, co-incubation with SPRC and SPRC@DMSN could significantly further increase the H2S level, while treated with SPRC showed an instant increase and decrease of H2S, and SPRC@DMSN group showed sustained release manner of H2S. However, the release of H2S was hardly observed in any group co-treated with PAG, which further proved that SPRC release H2S via CSE/H2S pathway.
As reported, macrophages could promote the release pro-inflammatory cytokines (such as TNF-α, IL-1β and IL-6) during the progression of AIA, while the release of anti-inflammatory cytokines (such as IL-10) was inhibited, and this abnormal homeostasis of cytokines might further initiate and perpetuate inflammation and bone destructive in the joint (Kinne et al. Citation2007; Szekanecz and Koch Citation2007; Udalova et al. Citation2016). As shown in , 10 μM of SPRC suppressed the expression of LPS-induced pro-inflammatory cytokines (TNF-α, IL-1β and IL-6) release and increased the release of IL-10 detected by ELISA. Similarly, the SPRC@DMSN showed similar trend while the SPRC@DMSN showed better anti-inflammatory effect. However, their anti-inflammatory effects were all inhibited after co-incubation with PAG, the CSE inhibitor.
Supplementation facilitated the production of endogenous H2S in vivo
The 3-day SPRC plasma concentration profile after single administration of supplementations was shown in , and the pharmaceutical parameters were listed in . The CMAX of SPRC@DMSN (∼43.61 μg mL−1) was slightly lower than the SPRC (53.66 μg mL−1) though, the SPRC@DMSN group (17.09 h) showed dramatically prolonged t1/2 than the SPRC group (1.56 h), while meantime, the TMAX was also increased from 1 h (SPRC group) to 3 h (SPRC@DMSN group). Notably, the SPRC@DMSN showed higher AUC than the SPRC.
Figure 4. The supplementations promoted endogenous H2S release in plasma through CSE/H2S pathway after single administration. (A) The plasma concentration of SPRC within 72 h (above) and first 12 h (below). (B, C) Supplementations could elevate the CSE expression in heart and liver. (D) Administration of DMSN did not influence the H2S level in vivo, but (E) the administration of SPRC or SPRC@DMSN could elevated the plasma H2S concentration within 72 h (image of the first 12-h H2S plasma concentration was shown at bottom). SPRC or SPRC@DMSN were single oral administrated at dosage of 100 mg kg−1 according to rats’ body weight (n = 6, mean ± SD).
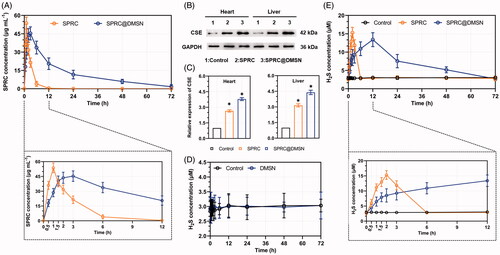
Table 2. Pharmacokinetic parameters of SPRC and SPRC@DMSN (n = 6, mean ± SD).
While SPRC was reported to promote H2S release in vivo through increasing the expression of CSE, which reported to be a main H2S releasing enzyme widely distributed in heart and liver, (Xin et al. Citation2016; Wang et al. Citation2017; Tran et al. Citation2019b) and oral administration of SPRC was found to increase the SPRC plasma concentration in an instant manner while the SPRC@DMSN in a sustained manner as illustrated above. The expression of CSE in liver and heart was firstly investigated, and results revealed that both SPRC and SPRC@DMSN could increase the expression of CSE (), while the SPRC@DMSN showed higher expression potentially due to its sustained releasing manner.
We then compared the H2S levels in plasma after single administration of SPRC, SPRC@DMSN, together with DMSN without SPRC loading. As shown in , the rats in control group or group treated with DMSN showed constant concentration of H2S in plasma around 3 μM within 72 h, which indicated the stable plasma H2S concentration in vivo. After administration with SPRC, the plasma H2S concentration showed a significant instant increase and peaked at the 2-hour post administration (). Different from the immediately release of H2S promoted by of SPRC, the H2S production from SPRC@DMSN was relative slow compared with the SPRC group, which was potentially due to the sustained release manner of SPRC from SPRC@DMSN.
Therapeutic efficacy of supplementation toward AIA in rats
As a long-term autoimmune disorder, the pro-inflammatory cytokines (TNF-α, IL-1β, and IL-6) and anti-inflammatory cytokine (IL-10) were important parameters to assess the therapeutic effects in AIA rats. As shown in , SPRC showed negligible decrease of pro-inflammatory cytokines and increase of anti-inflammatory cytokine. Meantime, the SPRC@DMSN showed significant anti-inflammatory effect. The alleviation of inflammation in AIA rats was studied through the paw volume and arthritis index (). From the results, no dramatic increase of paw volume and arthritis index in all groups before 10th day, while starting from 10th day, the AIA group and SPRC group showed a significant increase tendency, which indicated low therapeutic effect of SPRC. However, in SPRC@DMSN group, decrease level of paw volume and arthritis index could be observed. The SPRC@DMSN-treated rats had a marked reduction in cartilage degradation () and bone erosion () in comparison with the AIA group or SPRC-treated group.
Figure 5. Supplementations mitigated AIA symptoms. The pro-inflammatory cytokines levels of (A) TNF-α, (B) IL-1β, and (C) IL-6 and anti-inflammatory cytokine level of (D) IL-10 in rats were measured. Significant different compared with AIA group indicated as (*) (n = 6, mean ± SD). (E) The arthritis index and (F) paw volume were used to evaluate the severity of swollen symptoms (n = 6, mean ± SD). (G) Safranin-O staining examination was conducted to evaluate the articular cartilage destruction. (Scale bar = 100 μm). (H) The micro 3 D analysis images of rats’ paws, 3 D reconstructions of paws from rats used the Mimics software. The erosions sites of bones indicated by the white arrow.
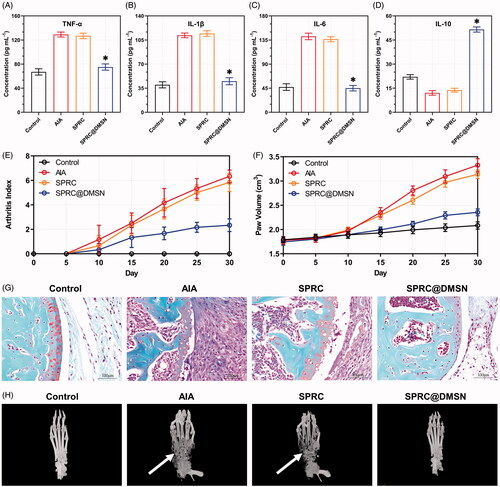
Safety evaluations
Chronic administration of high doses of SPRC generally leads to side effects such as body weight loss and hematologic abnormalities, which might limit the use of SPRC (Zheng et al. Citation2012). During the progression of arthritis, the body weight changes of AIA rats presented in . Compared with the control group, all other groups showed a decrease tendency in body weight might be due to the strong impact of AIA inflammation, especially starting from 10th day after induction of AIA model. At day 30, the body weight of control group (380.7 ± 12.32 g) was about 53 g heavier than the AIA group (327.1 ± 16.80 g) and 50 heavier than the SPRC group (330.6 ± 16.07 g), while the SPRC@DMSN (381.5 ± 10.86 g) showed a dramatic up-regulated of body weight loss at 30th day possibly because of the alleviation of inflammation symptoms. It was reported that a long-term exposure to even acceptable concentrations of H2S at workplace is a potential risk for human health (Saeedi et al. Citation2015), the hematologic study was conducted to evaluate the side effects of supplementations on rats, and results were shown in . Except the white blood cell (WBC) count showed a slight increase in the AIA and SPRC groups compared with other groups, no significant difference observed in any other groups’ hematologic studies, neither in red blood cell (RBC) count nor the hemoglobin (HGB) levels. In addition, to evaluate possible side effects of supplementations, the heart, liver, spleen, lung, and kidney were resected from rats and examined by H&E staining. Compared with the control group, no tissue damage could be observed in any experimental groups, which implied the low toxicity of supplementations in vivo ().
Figure 6. Evaluation of potential adverse effects of supplementations in AIA rats. (A) The body weight of rats was recorded every 5 days. After 30-day experiment, rats were sacrificed and (B) red blood cell (RBC), (C) white blood cell (WBC) count and (D) hemoglobin (HGB) were measured. (n = 6, mean ± SD). (E) H&E staining was carried out for the examination of heart, liver, spleen, lung, and kidney after 30 days’ experiment. Images were acquired at 200 × magnification (Scale bar = 100 μm).
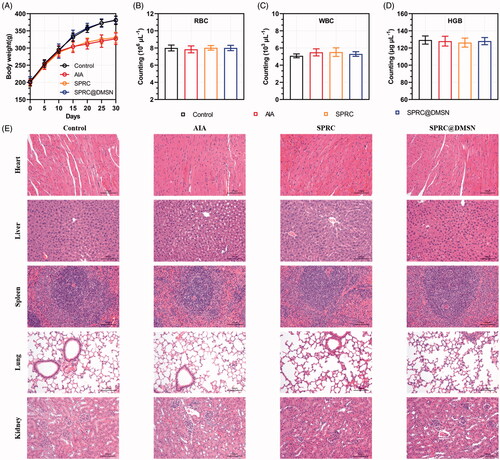
Discussions
SPRC, an excellent hydrophilic endogenous H2S donor, was reported to significantly mitigate the symptoms in diseases such as acute myocardial ischemia, (Wang et al. Citation2009) acute pancreatitis, (Tamizhselvi et al. Citation2010; Sidhapuriwala et al. Citation2012) and Alzheimer’s disease (Gong et al. Citation2011). However, SPRC might promote the release of H2S in an instant manner which could potentially cause neuronal toxicity with high dosage, thus limiting its further clinical use. Intended to solve the above problem, SPRC was successfully absorbed into DMSN in this study.
The H2S exists in forms of hydrogen sulfide (H2S), hydrogen sulfide anion (HS−), and sulfide anion (S2−) (). However, the H2S/HS−/S2− might react quickly with MBB to produce SDB (), and meantime, SDB could remain stable approximately for a period both in vitro and in vivo (Shen et al. Citation2011; Shen, Chakraborty, et al. Citation2014). With LC-MS, two peaks were detected, while SDB was peak 1 and internal standard was peak 2 (). To calculate the real-time H2S concentration both in vitro and in vivo, the endogenous H2S concentration was calculated according to the calibration curve of SDB in the range of concentration from 0.625 μM to 20 μM ().
Figure 7. The detection of endogenous H2S via LC-MS. (A) The acid dissociation constant of H2S and (B) the mechanism of MBB react with HS− produce SDB at alkaline and hypoxia environment; (C) The chromatography of SDB, peak 1: SDB, peak 2: hydrocortisone (internal standard); (D) The calibration curve of SDB in different concentration (0.625 μM–20 μM).
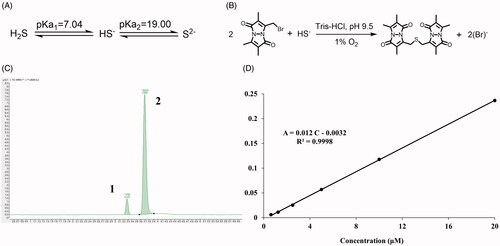
As reported, the released SPRC would elevate H2S levels via the CSE/H2S pathway in vivo, (MA et al. Citation2011; Zheng et al. Citation2011) and this pathway was also verified again in our study. The short half-life(Ma et al. Citation2015) of SPRC could result in the excretion before fully reacted with CSE, which lead to an incomplete H2S release in vivo. However, a longer and higher release of SPRC from SPRC@DMSN could be observed compared with SPRC powder in vivo (). Prolonged release of H2S was mainly because of the sustained release of SPRC from SPRC@DMSN. Besides, the central radially structure of pore channel enabled the perfusion of liquid medium, leading an accelerating degradation and higher biocompatibility of DMSN (Shen, Yang, et al. Citation2014).
Our group previously reported that daily administration of SPRC at dose of 100 mg kg−1 could alleviate the AIA symptoms in rats (Wu et al. Citation2016). However, in this study, the SPRC group showed low therapeutical effect (), which indicated an important role of maintaining H2S plasma concentration for the treatment of AIA. The SPRC@DMSN could elevate the H2S concentration for 3 days with single oral administration, while the SPRC lasted for only 6 h ().
Since bone erosion is also a key index for evaluation of AIA symptoms, the 3 D micro-CT was employed the effect on inhibition of bone erosion in AIA model after treatment (). The AIA model groups showed the severest bone erosion while the SPRC@DMSN-treated group significantly alleviate the symptom, successfully suggesting prevention of bone damage, and this therapeutic effect was potentially through the elevation of endogenous H2S.
As previous reported, (Ma et al. Citation2019; Alaaeldin et al. Citation2021) the decrease of body weight could be a sign of the establishment of AIA. At the 30th day, the body weight of control group was the highest among all groups, while AIA group showed the lowest body weight. At meantime, SPRC group showed the similar body weight change potentially due to its low therapeutic effect. However, the body weight of SPRC@DMSN was different with the AIA group, which potentially indicated its high therapeutic effect toward AIA in rats ().
Conclusions
In this study, DMSN was successfully prepared, and afterwards, the SPRC was successfully loaded into DMSN, which could sustain release SPRC, hence, attaining the sustained releasing manner of H2S both in vitro and in vivo. Potentially owing to sustained release of SPRC from SPRC@DMSN, the SPRC@DMSN not only showed a longer H2S release in vivo, but also a better therapeutic effect for AIA in rats. Taken together, DMSN could be a novel favorable drug carrier with low toxicity and high biocompatibility for the treatment of RA.
Disclosure statement
No potential conflict of interest was reported by the author(s).
Additional information
Funding
References
- Alaaeldin E, Abou-Taleb HA, Mohamad SA, et al. (2021). Topical nano-vesicular spanlastics of celecoxib: enhanced anti-inflammatory effect and down-regulation of TNF-α, NF-кB and COX-2 in complete Freund’s adjuvant-induced arthritis model in rats. IJN 16:133–45.
- Assouvie A, Daley-Bauer LP, Rousselet G. (2018). Growing murine bone marrow-derived macrophages. Methods Mol Biol 784:29–33.
- Balas F, Manzano M, Horcajada P, Vallet-Regí M. (2006). Confinement and controlled release of bisphosphonates on ordered mesoporous silica-based materials. J Am Chem Soc 128:8116–7.
- Bełtowski J, Jamroz-Wis¨niewska A. (2014). Hydrogen sulfide and endothelium-dependent vasorelaxation. Molecules 19:21183–99.
- Bossaller L, Rothe A. (2013). Monoclonal antibody treatments for rheumatoid arthritis. Expert Opin Biol Ther 13:1257–72.
- Braz WR, Rocha NL, De Faria EH, et al. (2016). Incorporation of anti-inflammatory agent into mesoporous silica. Nanotechnology 27:385103.
- Burguera EF, Meijide-Failde R, Blanco FJ. (2017). Hydrogen sulfide and inflammatory joint diseases. CDT 18:1641–52.
- Chan ESL, Fernandez P, Cronstein BN. (2007). Methotrexate in rheumatoid arthritis. Expert Rev Clin Immunol 3:27–33.
- Chen CH, Kuo SM, Tien YC, et al. (2020). Steady augmentation of anti-osteoarthritic actions of rapamycin by liposome-encapsulation in collaboration with low-intensity pulsed ultrasound. IJN 15:3771–90.
- Chen CQ, Xin H, Zhu YZ. (2007). Hydrogen sulfide: third gaseous transmitter, but with great pharmacological potential. Acta Pharmacol Sin 28:1709–16.
- Chuang CH, Cheng YC, Lin SC, et al. (2019). Atractylodin suppresses dendritic cell maturation and ameliorates collagen-induced arthritis in a mouse model. J Agric Food Chem 67:6773–84.
- Davignon JL, Hayder M, Baron M, et al. (2013). Targeting monocytes/macrophages in the treatment of rheumatoid arthritis. Rheumatology (United Kingdom) 52:590–8.
- Davoli A, Greco V, Spalloni A, et al. (2015). Evidence of hydrogen sulfide involvement in amyotrophic lateral sclerosis. Ann Neurol 77:697–709.
- Desai D, Prabhakar N, Mamaeva V, et al. (2016). Targeted modulation of cell differentiation in distinct regions of the gastrointestinal tract via oral administration of differently PEG-PEI functionalized mesoporous silica nanoparticles. Int J Nanomed 11:299–313.
- Eto K, Asada T, Arima K, et al. (2002). Brain hydrogen sulfide is severely decreased in Alzheimer’s disease. Biochem Biophys Res Commun 293:1485–8.
- Ferreira JF, Ahmed Mohamed AA, Emery P. (2016). Glucocorticoids and rheumatoid arthritis. Rheum Dis Clin North Am 42:33–46.
- Gong QH, Wang Q, Pan LL, et al. (2011). S-Propargyl-cysteine, a novel hydrogen sulfide-modulated agent, attenuates lipopolysaccharide-induced spatial learning and memory impairment: Involvement of TNF signaling and NF-κB pathway in rats. Brain Behav Immun 25:110–9.
- van Grieken R, Escola JM, Moreno J, Rodríguez R. (2009). Direct synthesis of mesoporous M-SBA-15 (M = Al, Fe, B, Cr) and application to 1-hexene oligomerization. Chem Eng J 155:442–50.
- Hu LF, Lu M, Tiong CX, et al. (2010). Neuroprotective effects of hydrogen sulfide on Parkinson’s disease rat models. Aging Cell 9:135–46.
- Huang C, Kan J, Liu X, et al. (2013). Cardioprotective effects of a novel hydrogen sulfide agent-controlled release formulation of S-propargyl-cysteine on heart failure rats and molecular mechanisms. PLoS ONE 8:e69205.
- Huang CC, Huang W, Yeh CS. (2011). Shell-by-shell synthesis of multi-shelled mesoporous silica nanospheres for optical imaging and drug delivery. Biomaterials 32:556–64.
- Huang X, Li L, Liu T, et al. (2011). The shape effect of mesoporous silica nanoparticles on biodistribution, clearance, and biocompatibility in vivo. ACS Nano 5:5390–9.
- Ishibashi T. (2013). Molecular hydrogen: new antioxidant and anti-inflammatory therapy for rheumatoid arthritis and related diseases. CPD 19:6375–81.
- Katsouda A, Bibli SI, Pyriochou A, et al. (2016). Regulation and role of endogenously produced hydrogen sulfide in angiogenesis. Pharmacol Res 113:175–85.
- Kinne RW, Stuhlmüller B, Burmester GR. (2007). Cells of the synovium in rheumatoid arthritis. Macrophages. Arthritis Res Ther 9:224.
- Kumari A, Yadav SK, Yadav SC. (2010). Biodegradable polymeric nanoparticles based drug delivery systems. Colloids Surf B Biointerfaces 75:1–18.
- Lee EB, Fleischmann R, Hall S, et al. (2014). Tofacitinib versus methotrexate in rheumatoid arthritis. N Engl J Med 370:2377–86.
- Lee S, Yun HS, Kim SH. (2011). The comparative effects of mesoporous silica nanoparticles and colloidal silica on inflammation and apoptosis. Biomaterials 32:9434–43.
- Lee ZW, Zhou J, Chen CS, et al. (2011). The slow-releasing Hydrogen Sulfide donor, GYY4137, exhibits novel anti-cancer effects in vitro and in vivo. PLoS ONE 6:e21077.
- Li L, Liu T, Fu C, et al. (2015). Biodistribution, excretion, and toxicity of mesoporous silica nanoparticles after oral administration depend on their shape. Nanomedicine 11:1915–24.
- Liang YH, Shen YQ, Guo W, Zhu YZ. (2014). SPRC protects hypoxia and re-oxygenation injury by improving rat cardiac contractile function and Intracellular calcium handling. Nitric Oxide 41:113–9.
- Liu Y, Huang B, Zhu J, et al. (2018). Dual-generation dendritic mesoporous silica nanoparticles for co-delivery and kinetically sequential drug release. RSC Adv 8:40598–610.
- Lopez-Olivo MA, Siddhanamatha HR, Shea B, et al. (2014). Methotrexate for treating rheumatoid arthritis. Cochrane Database Syst Rev 2014:CD000957.
- Lougiakis N, Papapetropoulos A, Gikas E, et al. (2016). Synthesis and pharmacological evaluation of novel adenine-hydrogen sulfide slow release hybrids designed as multitarget cardioprotective agents. J Med Chem 59:1776–90.
- Low PS, Henne WA, Doorneweerd DD. (2008). Discovery and development of folic-acid-based receptor targeting for imaging and therapy of cancer and inflammatory diseases. Acc Chem Res 41:120–9.
- Lu M, Liu YH, Goh HS, et al. (2010). Hydrogen sulfide inhibits plasma renin activity. J Am Soc Nephrol 21:993–1002.
- Luo Z, Cai K, Hu Y, et al. (2011). Mesoporous silica nanoparticles end-capped with collagen: Redox-responsive nanoreservoirs for targeted drug delivery. Angew Chem 123:666–9.
- Ma G, Zhang L, Zhang P, et al. (2015). Physicochemical characteristics and gastrointestinal absorption behaviors of S-propargyl-cysteine, a potential new drug candidate for cardiovascular protection and antitumor treatment. Xenobiotica 45:322–34.
- MA K, Liu Y, Zhu Q, et al. (2011). H2S donor, S-propargyl-cysteine, increases CSE in SGC-7901 and cancer-induced mice: evidence for a novel anti-cancer effect of endogenous H2S? PLoS ONE 6:e20525.
- Ma Z, Tao C, Sun L, et al. (2019). In situ forming injectable hydrogel for encapsulation of nanoiguratimod and sustained release of therapeutics. IJN 14:8725–38.
- Mccarthy CA, Ahern RJ, Dontireddy R, et al. (2016). Mesoporous silica formulation strategies for drug dissolution enhancement: a review. Expert Opin Drug Deliv 13:93–108.
- McInnes IB, Schett G. (2011). The pathogenesis of rheumatoid arthritis. N Engl J Med 365:2205–19.
- Mieszawska AJ, Mulder WJM, Fayad ZA, Cormode DP. (2013). Multifunctional gold nanoparticles for diagnosis and therapy of disease. Mol Pharm 10:831–47.
- Mustafa AK, Sikka G, Gazi SK, et al. (2011). Hydrogen sulfide as endothelium-derived hyperpolarizing factor sulfhydrates potassium channels. Circ Res 109:1259–68.
- Pan LL, Liu XH, Gong QH, Zhu YZ. (2011). S-Propargyl-cysteine (SPRC) attenuated lipopolysaccharideinduced inflammatory response in H9c2 cells involved in a hydrogen sulfide-dependent mechanism. Amino Acids 41:205–15.
- Papapetropoulos A, Pyriochou A, Altaany Z, et al. (2009). Hydrogen sulfide is an endogenous stimulator of angiogenesis. Proc Natl Acad Sci U S A 106:21972–7.
- Ray A, Kumar D, Shakya A, et al. (2004). Serum amyloid A-activating factor-1 (SAF-1) transgenic mice are prone to develop a severe form of inflammation-induced arthritis. J Immunol 173:4684–91.
- Rutherford A, Nikiphorou E, Galloway J. (2017). Rheumatoid arthritis. In: Comorbidity in rheumatic diseases. Cham: Springer, 53–79.
- Saeedi A, Najibi A, Mohammadi-Bardbori A. (2015). Effects of long-term exposure to hydrogen sulfide on human red blood cells. Int J Occup Environ Med 6: 20–5.
- Sahni JK, Doggui S, Ali J, et al. (2011). Neurotherapeutic applications of nanoparticles in Alzheimer’s disease. J Controlled Release 152: 208–31.
- Shen D, Yang J, Li X, et al. (2014). Biphase stratification approach to three-dimensional dendritic biodegradable mesoporous silica nanospheres. Nano Lett 14: 923–32.
- Shen X, Chakraborty S, Dugas TR, Kevil CG. (2014). Hydrogen sulfide measurement using sulfide dibimane: Critical evaluation with electrospray ion trap mass spectrometry. Nitric Oxide 41:97–104.
- Shen X, Pattillo CB, Pardue S, et al. (2011). Measurement of plasma hydrogen sulfide in vivo and in vitro. Free Radic Biol Med 50: 1021–31.
- Shin HJ, Park H, Shin N, et al. (2020). p66shc siRNA nanoparticles ameliorate chondrocytic mitochondrial dysfunction in osteoarthritis. IJN Volume 15:2379–90.
- Sidhapuriwala JN, Hegde A, Ang AD, et al. (2012). Effects of s-propargyl-cysteine (sprc) in caerulein-induced acute pancreatitis in mice. PLoS ONE 7:e32574.
- Smolen JS, Steiner G. (2003). Therapeutic strategies for rheumatoid arthritis. Nat Rev Drug Discov 2: 473–88.
- Stamp LK, Cleland LG. 2007. Rheumatoid arthritis. Optimizing Women’s Health through Nutrition.
- Streng T, Axelsson HE, Hedlund P, et al. (2008). Distribution and Function of the Hydrogen Sulfide-Sensitive TRPA1 Ion Channel in Rat Urinary Bladder. Eur Urol 53: 391–400.
- Szekanecz Z, Koch AE. (2007). Macrophages and their products in rheumatoid arthritis. Current Opinion in Rheumatology 19:289–95.
- Tamizhselvi R, Koh YH, Sun J, et al. (2010). Hydrogen sulfide induces ICAM-1 expression and neutrophil adhesion to caerulein-treated pancreatic acinar cells through NF-κB and Src-family kinases pathway. Exp Cell Res 316: 1625–36.
- Thomas TP, Goonewardena SN, Majoros IJ, et al. (2011). Folate-targeted nanoparticles show efficacy in the treatment of inflammatory arthritis. Arthritis Rheum 63: 2671–80.
- Tran BH, Huang C, Zhang Q, et al. (2015). Cardioprotective effects and pharmacokinetic properties of a controlled release formulation of a novel hydrogen sulfide donor in rats with acute myocardial infarction. Bioscience Reports 35: 1–12.
- Tran BH, Yu Y, Chang L, et al. (2019a). A novel liposomal S-propargyl-cysteine: a sustained release of hydrogen sulfide reducing myocardial fibrosis via TGF-β1/smad pathway. Int J Nanomed 14:10061–77.
- Tran BH, Yu Y, Chang L, et al. (2019b). A novel liposomal S-propargyl-cysteine: A sustained release of hydrogen sulfide reducing myocardial fibrosis via TGF-β1/smad pathway. IJN Volume 14:10061–77.
- Udalova IA, Mantovani A, Feldmann M. (2016). Macrophage heterogeneity in the context of rheumatoid arthritis. Nat Rev Rheumatol 12: 472–85.
- Wang M, Xin H, Tang W, et al. 2017. AMPK Serves as a Therapeutic Target Against Anemia of Inflammation. Antioxidants and Redox Signaling.
- Wang Q, Liu HR, Mu Q, et al. (2009). S-propargyl-cysteine protects both adult rat hearts and neonatal cardiomyocytes from ischemia/hypoxia injury: The contribution of the hydrogen sulfide-mediated pathway. Journal of Cardiovascular Pharmacology 54: 139–46.
- Wang Q, Wang XL, Liu HR, et al. (2010). Protective effects of cysteine analogues on acute myocardial ischemia: Novel modulators of endogenous H2S production. Antioxidants and Redox Signaling 12: 1155–65.
- Wu WJ, Jia WW, Liu XH, et al. (2016). S-propargyl-cysteine attenuates inflammatory response in rheumatoid arthritis by modulating the Nrf2-ARE signaling pathway. Redox Biol 10:157–67.
- Xin H, Wang M, Tang W, et al. 2016. Hydrogen Sulfide Attenuates Inflammatory Hepcidin by Reducing IL-6 Secretion and Promoting SIRT1-Mediated STAT3 Deacetylation. Antioxidants and Redox Signaling.
- Yang J, Shen D, Wei Y, et al. (2015). Monodisperse core-shell structured magnetic mesoporous aluminosilicate nanospheres with large dendritic mesochannels. Nano Res 8: 2503–14.
- Zheng Y, Liu H, Ma G, et al. (2011). Determination of S-propargyl-cysteine in rat plasma by mixed-mode reversed-phase and cation-exchange HPLC-MS/MS method and its application to pharmacokinetic studies. J Pharm Biomed Anal 54: 1187–91.
- Zheng YT, Zhu JH, Ma G, et al. (2012). Preclinical assessment of the distribution, metabolism, and excretion of S-propargyl-cysteine, a novel H 2S donor, in Sprague-Dawley rats. Acta Pharmacol Sin 33: 839–44.
- Zhu YZ, Zhong JW, Ho P, et al. (2007). Hydrogen sulfide and its possible roles in myocardial ischemia in experimental rats. Journal of Applied Physiology 102:261–8.