Abstract
The amount of lipase from Mucor miehei adsorption on ultrafiltration polysulfone hollow fiber membrane chips has been determined using different lipase concentrations at three different temperatures, namely 30, 35, and 40°C. It was experimentally shown that adsorption of lipase increases with temperature. The results were used to evaluate the constants found in the Langmuir adsorption isotherm model coupled with the Van't Hoff's relationship. A temperature dependence correlation for the amount of adsorbed lipase activity, alip,ads, and that present in the supernatant solution, alip,free was determined. The effect of varying the concentration on a cross-linking agent, namely, glutaraldehyde, to the membrane chips was also tested. It was found that, under the same operating conditions, the amount of lipase adsorbed on polysulfone membranes was increased dramatically after pre-treating the membrane with 1% Glutaraldehyde. However, increasing the concentration of the cross-linking agent has a low effect on the amount of lipase adsorbed.
1. INTRODUCTION
The applications, importance and significance of lipase in oleochemical industry have been thoroughly demonstrated in literature [Citation[1-12]]. Lipases are spontaneously soluble in aqueous solutions as a result of their globular protein nature [Citation[3]], but their natural substrates (i.e., lipids) are not. Using a proper organic solvent or an emulsifier helps to overcome the problem of intimate contact between substrate and enzyme [Citation[1], Citation[2]]. However, the practical use of lipase in such psuedohomogeneous reaction systems poses technological difficulties such as contamination of the products with residual enzymatic activity and economic difficulties such as the use of enzyme for a single reactor pass [Citation[4]]. Hence, part of the overall potential enzymatic activity is lost. If the lipase is immobilized, then it becomes an independent phase within the reaction system, which may be easily retained in the reactor with concomitant advantages in preventing contamination of the products and extending its useful active life [Citation[4]]. Further, increasing temperature generally increases the rate of lipase-catalysed reaction per unit amount of active enzyme; however, increasing temperature also leads to a higher thermal deactivation rate of the lipase itself, thus yielding decreasing amounts of active enzyme [Citation[1], Citation[5]]. Because immobilization provides a more rigid external backbone for lipase molecules, the temperature optima are expected to increase [Citation[2]], which results in faster rate of reaction.
Among all immobilization methods, adsorption has been clearly elected by most researchers [Citation[4]]. The ease of physical adsorption, absence of expensive and toxic chemicals, ability to retain the specific activity and selectivity of the lipase virtually unchanged with respect to its soluble form, and feasibility of regeneration (based on partial reversibility of the immobilization technique) may partially account for this realization. On the other hand, poor adsorption of enzyme results in its leaching off the support surface. This favors other means of enzyme immobilization such as covalent bonding, entrapment and encapsulation. Nevertheless, physical adsorption remains the most industrially attractive method because of its simplicity and economical effectiveness [Citation[6]].
It is possible to strengthen the attachment between the water-soluble enzyme and the water-insoluble membranes by using multifunctional agents that are all bifunctional in nature and have low molecular weight, such as glutaraldehyde [Citation[3], Citation[4]]. The employment of glutaraldehyde results in establishment of intermolecular (and possible intramolecular) cross-links at amino groups [Citation[4]].
The use of immobilized lipase in membrane reactors with simultaneous separation of the two different phases has been reported in the literature [Citation[3-6], Citation[9-14]]. However, the study of adsorption characteristics of lipase on these membranes is still lacking. The objective of this study is to investigate the immobilization of lipase from Mucor miehei on polysulfone microporous hollow fiber ultrafiltration membrane chips. The effect of lipase bulk concentration, temperature and the use of cross-linking agent are examined.
2. ADSORPTION MODEL
The classical model that governs the adsorption is the Langmuir adsorption isotherm, which has successfully been used in the literature to fit equilibrium protein adsorption data [Citation[2], Citation[11]].
The equation above governs the relation between the amount of adsorbed lipase activity, aads, and that present in the supernatant solution, afree, at equilibrium. The adsorption equilibrium constant, Kads and aads,max the maximum amount of lipase that can be adsorbed per unit area of membrane, are temperature dependent parameters [Citation[11]]. A convenient way of expressing this temperature dependence takes advantage of Van't Hoff's relationship between the equilibrium constant and the standard enthalpy change associated with the process under consideration [Citation[11]]. This leads to the relation:where, Δhads is the standard enthalpy change accompanying the reversible adsorption of protein on the membrane surface, and α, β and ε are constants. A linearized relationship between the amount of adsorbed lipase activity, alip,ads, and that present in the supernatant solution, alip,free, is derived by substituting Eqs. (Citation[2], Citation[3]) into Eq. (1) and rearranging to get:
where,
The constants found in Eqs. (1-3) can be determined experimentally by measuring the amount of lipase adsorbed on the membranes membrane chips for different enzyme bulk concentrations at different temperatures.
3. MATERIALS AND METHODS
3.1. Materials and Equipment
Liquid lipase (EC 3.1.1.3) from Mucor miehei (claimed activity 100,000 LU ml−1) was obtained from Novo Nordisk, Denmark. Analytical grade tributyrin and glycerol was obtained from Merck Co., Spain. Gum Arabic, NaCl, KH2PO4, NaOH and glutaraldehyde in water (electron-microscopy-grade 25 % wt/wt) were obtained from Sigma Chemicals Co., Germany. Auto titrator (Metlor Tolido, Swaziland) was used to determine the enzyme activity. The hollow fiber membranes used in this research were asymmetric hydrophobic microporous ultrafiltration hollow fiber membranes used for kidney dialysis, purchased from ASAHI, Japan. The material of the fibers was polysulfone that has an internal diameter of 200 µm and a wall thickness of 45 µm. As illustrated in , the inner side of the fibers is a thin layer supported by the spongy layer from the outside. This structure is believed to achieve high retention of molecules with weight up to 66,000 Daltons, which makes it suitable to use with lipase having molecular weight between 40,000 to 50,000 Daltons [Citation[12]].
3.2. Experimental Methods
Two identical beakers were used in each run of the experiment. One beaker contains 1 g of the hollow fiber membranes cut into small pieces, 1 cm long, in 250 ml enzyme solution of known concentration prepared in buffer and 18% v/v glycerol solution as enzyme stabilizer at pH 7.0. The other, used as a blank solution, contains the same concentration and volume of enzyme solution but without any membrane chips. The beakers were placed on a magnetic stirrer and stirred gently. Samples of enzyme solutions from each beaker were withdrawn at desired time intervals and analyzed for the amount of lipase activity. The amount of lipase adsorbed on the membranes, at any time, was calculated from the difference in lipase activities of the blank beaker and the beaker that contains the chips. This accounts for temperature and shear inactivation of lipase throughout the course experiment. The method of enzyme assay is based on the hydrolysis of tributyrin and titrating the butyric acids produced with 0.05 M NaOH in distilled water [Citation[15]]. The alkali consumption is registered as a function of time under standard conditions of 30°C and pH 7.0, using auto-titrator. From the amount of alkali consumed, the equivalent amount of butyric acid in the samples was calculated and the enzyme activity was determined. The enzyme activity is expressed in Lipase Units (LU), where 1 LU is defined as the amount of enzyme that liberates 1 µmol of butyric acid per min at 30°C. The experiment was carried out at three different initial enzyme activities, 500, 333 and 200 LU ml−1. For each case the experiment was repeated at three different temperatures, namely 30, 35 and 40°C.
The effect of pre-treating the chips with different concentrations glutaraldehyde on the adsorption of lipase was also investigated. This was achieved by socking predetermined concentration of glutaraldehyde solution (1, 2, and 3%) in phosphate buffer for five hours at constant temperature of 40°C and pH 7.0. After the membrane had undergone coupling with lipase, it was washed three times using distilled water to remove all the traces of the cross-linking agent followed by removing the moisture by vacuum filtering using 0.45 µm filter. After that, the same procedure mentioned earlier for lipase immobilization was carried out to obtain chemical adsorption.
4. RESULTS AND DISCUSSION
To determine the constants found in the adsorption model equations (Eq. 4-6), the amount of lipase adsorbed on the surface of the polysulfone membrane chips was determined for different equilibrium lipase activities at different temperatures. For each pre-specified operating condition the changes in the enzyme activity with time were recorded. shows the enzyme activity profile during the adsorption experiment at initial bulk activity of 333 LU ml−1 at 40°C. The difference in enzyme activity from the two beakers reflects the amount of enzyme adsorbed on the surface of the membrane. It can be seen from that the amount of lipase adsorbed increases as time proceeds, then at around 300 min no more adsorption is recorded. Hence, 300 min. is taken as the system equilibrium time. Adsorption of Candida cylindricea lipase enzyme on a polypropylene hollow fiber membrane reactor was reported earlier [Citation[14]]. The observed trends were similar to the ones obtained in this study with an equilibrium time of 180 min.
Figure 2 Changes in the enzyme activity with time for the adsorption of lipase on the polysulfone membrane at 40°C using lipase solution with an initial activity of 333 LU ml−1.
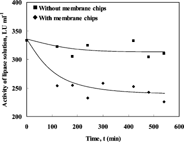
Similar graphs to were produced for different initial lipase activities and temperatures to determine the effect of temperature. shows the amount of adsorbed enzyme on the polysulfone membrane at three constant temperatures 30, 35 and 40°C using lipase solution of 200 LU ml−1. It has been reported that at these temperatures the enzyme is thermally stable [Citation[1], Citation[5], Citation[12]]. It is clearly shown that increasing the temperature leads to an increase in the equilibrium amount of enzyme adsorbed on the membrane surface. This contradicts to the general behavior for physical adsorption, where the amount of adsorbed materials decreases with increasing temperature [Citation[2], Citation[11]]. On the other hand, Basri et al. [Citation[17]] reported an increase in adsorption of enzyme on polymer beads with temperature, similar to the result found in this study. This increase in adsorption with temperature is explained in two ways: Firstly, assuming a pure physical adsorption of lipase, the diffusion coefficient of lipase molecule increases and the viscosity of the solution reduces as temperature increases. Hence, at higher temperatures, a larger portion of the membrane micropore structure is rendered accessible to the enzyme solution and penetration of enzyme inside the membrane is enhanced. The increase in the amount of lipase adsorbed, at higher temperatures, is also due to the increase in hydrophobic interaction between the membrane and the enzyme molecures at higher temperatures [Citation[17]].
Figure 3 Amount of adsorbed enzyme on the polysulfone membrane at three constant temperatures, 30, 35 and 40°C, using lipase solution of 200 LU ml−1.
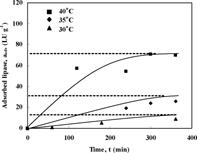
Secondly, assuming chemisoption of lipase on polysulfone membrane material plays a significant role, like any other chemical reaction, chemisorption reaction rate increases with temperature. Then, a higher amount of enzyme is adsorbed.
shows the amount of lipase adsorbed, aads, versus equilibrium free lipase concentration, afree, in the range of initial lipase concentration and temperature considered, i.e., 200–500 LU ml−1 and 30–40°C, respectively. The data shown in were fitted to the Langmuir adsorption isotherm model (Eqs. 4-6) and the estimated model parameters are given in . The resultant temperature dependence correlation for the amount of adsorbed lipase is given by Eq. (7),
Figure 4 Comparison between experimental results and the proposed correlation for the amount of lipase adsorbed, aads, versus equilibrium free lipase concentration, afree. (▪, ♦, and ▴ are experimental results and – is the correlation curve at each temperature.)
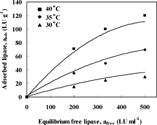
Table 1. Langmuir adsorption model parameters
On the other hand, the effect of pre-treating the chips with three different concentrations of glutaraldehyde, namely 1, 2 and 3%, on the adsorption of lipase was also investigated. This experiment was performed using initial enzyme activity of 500 LU ml−1 in 18% glycerol solution at 40°C. shows that the amount of lipase adsorbed on polysulphone membranes was increased by 83.3% after pre-treating the fibers with 1% of the cross-linking agent, from 120 to 220 LU g−1. However, increasing the concentration of the cross-linking agent in the pre-treated membrane solution reagent has little effect on the amount of lipase adsorbed. The percentage increase by using 2% was 108%, equivalent to a further increase of 25%, while the percentage increase by using 3% was 112.5, which is equivalent to a further increase of only 2%.
5. CONCLUSION
Lipase enzyme has been successfully immobilized on hollow fiber membrane chips. This finding strengthens the idea of using hollow fiber tubular membranes as a bioreactor. It was experimentally shown that the adsorption of lipase on polysulfone membrane increases with concentration of lipase in the supernatant solution and/or temperature in the ranges considered in this study. The results were used to evaluate the constants found in the Langmuir adsorption isotherm model coupled with the Van't Hoff's relationship. A temperature dependence correlation for the amount of adsorbed lipase activity, alip,ads, and that present in the supernatant solution, alip,free was determined. This expression is useful for predicting the amount of adsorbed lipase on polysulfone hollow fiber membrane at different temperatures and initial enzyme activities and for determining the optimum operating conditions. It was also found that, under the same operating conditions, the amount of lipase adsorbed on polysulphone membranes was increased dramatically by pre-treating the membrane with glutaraldehyde as a cross-linking agent due to chemical adsorption.
REFERENCES
- Al-Zuhair, S., Hasan, M., Ramachandran, K.B. (2003). Kinetic hydrolysis of palm oil using lipase. Proc. Biochem. 38: 1155–1163. [CSA]
- Knezevic, Z., Mojovic, L., Adnabjevic, B. (1998). Palm oil hydrolysis of lipase from candida cylindracea immobilised on zeolite Type Y. Enz. Microb. Technol. 22(4): 275–280. [CSA], [CROSSREF]
- Bjorkling, F., Godtfredsen, S.E., Kirk, O. (1991). The future impact of industrial lipases. Trens. Biotech. 9: 360–363. [CSA], [CROSSREF]
- Balcao, V.M., Paiva, A.L., Malcata, F.X. (1996). Bioreactors with immobilised lipases: state of the art. Enz. and Microb. Tech. 18: 392–416. [CSA], [CROSSREF]
- Shamel, M.M., Ramachandran, K.B., Hasan, M. (2002). Stability of lipase enzyme: effect of temperature and shear. Regional Symposium of Chemical Engineers RSCE 2002, Kuala Lumpur, Malaysia, 165–168.
- Guit, R.P.M., Kloosterman, M., Meindersma, G.W., Mayer, M., Meijer, E.M. (1991). Lipase kinetics: hydrolysis of triacetin by lipase from candida cylindracea in a hollow fibre membrane reactor. Biochem. and Bioeng. 38: 727–732. [CSA], [CROSSREF]
- Al-Duri, B., Robinson, E., McNerlan, S., Bailie, P. (1995). Hydrolysis of edible oils by lipases immobilized on hydrophobic support: effect of internal support structure. J.A.O.C.S. 72(11): 1351–1359. [CSA]
- Shamel, M.M., Ramachandran, K.B., Hasan, M. (2003). Effect of hollow fiber membrane material and operating conditions on lipase adsorption. IMSTEC ′03, The Fifth International Membrane Science & Technology Conference, Australia.
- Malcata, F.X., Hill, J.C., Amundson, C.H. (1992). Hydrolysis of butteroil by immobilised lipase using a hollow-fibre reactor. Part II. Uniresponse kinetic studies. Biotech. and Bioeng. 39: 984–1001. [CSA], [CROSSREF]
- Malcata, F.X., Hill, J.C., Amundson, C.H. (1992). Hydrolysis of butteroil by immobilised lipase using a hollow-fibre reactor. Part III. Multiresponse kinetic studies. Biotech. and Bioeng. 39: 1002–1012. [CSA], [CROSSREF]
- Malcata, F.X., Hill, J.C., Amundson, C.H. (1992). Hydrolysis of butteroil by immobilised lipase using a hollow-fiber reactor. Part I. Lipase adsorption studies. Biotech. and Bioeng. 39: 647–657. [CSA], [CROSSREF]
- Tsai, S.W., Shaw, S.S. (1998). Selection of hydrophobic membrane in the lipase-catalysed hydrolysis of olive oil. J. Membrane Sci. 146: 1–11. [CSA], [CROSSREF]
- Hoq, M., Tsuneo, Y., Shimizu, S., Funada, T., Ishida, S. (1985). Continuous hydrolysis of olive oil by lipase in microporous hydrophobic membrane. J.A.O.C.S. 62: 1016–1021. [CSA]
- Hoq, M., Koike, M., Tsuneo, Y., Shimizu, S. (1985). Continuous hydrolysis of olive oil by lipase in microporous hydrophobic hollow bioreactor. Agri. Bio. Chem. 49(11): 3171–3178. [CSA]
- Novo Industrials. (1995). Analytical Method Handout, Enzyme Process Division, Novo Industries: Denmark.
- Bruce, L., Henrik, K., Robert, L. (1991). Thermostable enzymes for industrial applications. Journal of Industrial Microbiology 8: 1–10. [CSA], [CROSSREF]
- Basri, M., Ampon, K., Yunus, W.M., Che Nyonya, A.R., Bakar, S. (1994). Immobilization of hydrophobic lipase derivatives on to organic polymer beads. J. Chem. Tech. Biotechnol. 59: 37–44. [CSA], [CROSSREF]