Abstract
A three-dimensional biodegradable porous scaffold plays a vital role in a tissue engineering approach. Collagen, chitosan and hyaluronan (HA) are natural extracellular matrix (ECM) or similarity, and may provide appropriate environment for the generation of cartilage-like tissue. In this study, we prepared a collagen/chitosan/HA tri-copolymer porous scaffold by freezing and lyophilization to evaluate physico-chemical properties of the tri-copolymer scaffold and its capacity to sustain chondrocytes proliferation and differentiation in vitro. The results show that the mechanical strength, the resistance to enzymatic degradation, and the waterblinding capacity were improved when chitosan and hyaluronan were incorporated into a collagen scaffold. After 21 days of culture, the porous scaffold had been surfaced with cartilaginous tissue. DNA and glycosaminoglycan (GAG) contents were significantly higher during culture periods in collagen/chitosan/hyaluronan matrix compared to collagen alone matrix, and most seeded cells preserved the chondrocytic phenotype during culture within the scaffold. The collagen/chitosan/hyaluronan tri-copolymer scaffold has potential applications in a cartilage tissue engineering scaffold field.
INTRODUCTION
Adult articular cartilage defect is a major problem in orthopedic surgery due to the limited capability for repair and self-regeneration, so minor injury of particular cartilage may lead to progressive damage and degeneration. Most of the current therapies of repair damaged cartilage have focused on the abrasion arthroplasty, excission, and the use of perichondreal or periosteal autografts. But the results are variable, these techniques have some limitations, and all fail to produce long-lasting repair tissue [Citation[1-4]].
Recently, tissue engineering has emerged as a potential alternative to tissue or organ transplantation, involving the combining of chondrocytes or multipotential chondral progenitor cells and a biodegradable porous scaffold, and it has been proved to be one of the most promising approaches for cartilage repair [Citation[5], Citation[6]].
A three-dimensional biodegradable porous scaffold plays a vital role as an alternative cell seeding and cell delivery vehicle in a tissue engineering approach. An optimal scaffold should be able to provide the necessary support for cells to proliferate and maintain their differentiation function [Citation[7]]. Biological friendliness and biomechanical strength were two critical factors for biomaterials in cartilage tissue engineering [Citation[8]]. These characteristic may provide a biochemically and biomechanically appropriate environment, which is necessary for chondrocytes within matrices to regenerate a long-lasting cartilage tissue in the defect site.
Traditionally, cartilage tissue engineering studies have used PGA, poly-l-Lactic acid (PLA), or a copolymer of PGA and PLA (PLGA) to make a scaffold [Citation[9]]. However, these materials have certain shortcomings in that they are non-biological and lack informational structure for cell attachment, and their hydrophobic property hinders cell seeding. In addition, their degradation products, e.g., glycolic acid and lactic acid, are acidic and lower the pH around tissue after in vivo implantation, which may cause severe inflammation [Citation[10]].
The ideal cell porous scaffold should be similar to the natural environment in the cartilage matrix. It is a key factor in the development of an engineered tissue to use biomaterial that is similar to the target tissue's extracellular matrix to repair or substitute tissue.
Collagen is a natural extracellular matrix (ECM) of many tissues and has a great potential in tissue engineering for its low antigenicity, biodegradability and good cell-blinding properties [Citation[11]]. In addition, the fibrous structure of the collagen is favorable to cell growth. Chitosan is a natural polysaccharide that is structurally similar to glycosaminoglycan (GAG). Furthermore, the incorporation of chitosan into a collagen scaffold is known to increase its mechanical strength, as it forms an ionic complex between the positively charged chitosan and the negatively charged with anionic polymers such as collagen and HA [Citation[12]]. Besides, chitosan shares some characteristics with various GAGs. Owing to this similarity to GAGs, chitosan has been attempted as a scaffold for cartilage repair [Citation[13]]. Hyaluronan is an important component of the ECMs and has been found to play a role in enhancing cell adhesion, cell proliferation and cell differentiation. Hyaluronan also can stimulate chondroitin sulfate synthesis by rabbit articular chondrocytes [Citation[14], Citation[15]].
We assumed that the collagen/chitosan/hyaluronan tri-copolymer would provide chondrocytes with a familiar and supportive environment due to its similarity to the GAG-rich ECM of the cartilage, and thus allow the cells to maintain many of the characteristics of differentiated chondrocytic phenotypes in vitro.
A tri-copolymer scaffold was produced from collagen, chitosan and sodium hyaluronate in this study, and the preliminary results that chondrocytes were cultured in the scaffold in vitro were also described.
MATERIALS AND METHODS
Fabrication of Collagen-Chitosan-Hyaluronan Porous Scaffolds
Native insoluble type I collagen was isolated from bovine achilles tendon and treated by the method of enzyme digestion described by Zhang et al. [Citation[16]].
The blended collagen/chitosan/sodium hyaluronate (HA) scaffold was made by the freeze-drying method. A 0.6% (w/v) collagen solution (0.3% dilute acetic media) was blended with a 1% (w/v) chitosan solution (2% acetic acid solution), and 1% HA (0.3% dilute acid media), resulting in 20% chitosan/mixture solution (w/w), 1% sodium hyaluronate/mixture solution (w/w), respectively. The mixture was homogenized in a high-speed stirrer and was defoamed for 10 min in a freeze centrifuge (2000 rpm, 4°C) to form a homogeneous solution. These blends were then frozen at − 40°C and lyophilized for 72 h in a freeze dryer. The same method has been used to prepare collagen matrices without chitosan and HA, and collagen/chitosan matrices from collagen and chitosan without HA, respectively.
These scaffolds were cross-linked respectively by 1-ethyl-3-(3-dimethyl inaminopropyl)carbodiimide (EDC). Matrices of 50 mg dry weight were incubated for 0.5 h in 20 ml of 40% ethanol containing 50 mmol/L 2-morpholinoethanesulphonic acid (MES) (pH 5.5). Subsequently, the matrices were cross-linked by immersion in the 19.5 ml of 40% ethanol containing 50 mM MES (pH 5.0), 33 mM EDC and 8 mM NHS. After reaction for 4 h, the matrices were washed in 0.1 M Na2HPO4 (pH 9.1) for 1 h. Finally, the matrices were washed with 1 M NaCl and 2 M NaCl for 2 h and 1 day, respectively, followed by washing with distilled water and lyophilization.
Matrix morphology and porosity were analyzed using scanning electron microscopy.
Determination of Physico-Chemical Characteristics
Water-Blinding Capacity
Matrix samples of about 5 mg dry weight were incubated in 3 ml PBS (pH 7.2) at 20°C for 1 h.The wet weight was determined and the water-blinding capacity calculated using the equation: water-blinding capacity=(wet weight − dry weight)/(dry weight).
Matrix Degradation by Collagenase
220 U/mg bacterial collagenase was dissolved in 0.1 M Tris-HCl (pH 7.4), containing 50 mM CaCl2. Matrices of about 10 mg dry weight were incubated in the 5 ml enzyme solution for 5 h at 37°C. Subsequently, matrices were washed with distilled water 3 times and lyophilized. Matrix degradation was determined from the weight of the residual matrix, and expressed as a percentage of the original weight.
Mechanical Properties
The ultimate broken strength of the matrices was measured with an HE-HOUNSFIELD at room temperature with a drawing speed of 100 mm/min. The matrices were rectangular disks 1 cm × 5 cm × T. T is the thickness of the matrices.
Chondrocytes Isolation and Seeding
Chondrocytes were obtained from the articulars of three–week–old New Zealand White rabbits. The cartilage was minced and washed three times in phosphate-buffered saline (PBS; pH 7.4). The minced cartilage was digested with 0.25% trypsin for 45 minutes, and then digested with 0.2% collagenase for 4 h in 37°C. The digestion solution was filtrated through a sterile 70 µm nylon mesh to remove any undigested fragments, and the chondrocytes were subsequently collected by centrifugation and washed twice with PBS. The cells' number and viability were determined by using a hemocytometer and the Trypan bule dye exclusion. The collected cells were suspended in culture medium (DMEM containing 10% fetal bovine serum, 4500 mg/l glucose, 584 mg/l glutamine, 100 U/ml penicillin, 100 µg/ml streptomycin, 10 mM HEPES, and 50 mg/l ascorbic acid), seeded in a 25-cm2 flask at a cell density of 1 × 105 cells/cm2 and subcultured at an atmosphere of 5% CO2 at 37°C. After being subcultured twice, the chondrocytes were collected with trypsin treatment.
The third passage chondrocytes were trypsinized and re-suspended at a concentration of 1 × 107 cells/ml DMEM. Crosslinked collagenous matrices were sterilized by γ. Matrices, 14 mm in diameter and approximately 1.5-mm thick, were placed in 24-well plates, and about 100 µl of cell suspension was injected into each scaffold, then were cultured at 37°C, 5% CO2, and 95% humidity up to 21 days. The medium (1 ml) in plates was changed every day. Samples were collected on 1st, 3rd, 7th, 14th, and 21st days, respectively.
DNA and Glycosaminoglycan (GAG) Content
The DNA content of the matrices at each time period was evaluated using the Hoechst 33258 fluorescent dye assay [Citation[17]]. The cell-seeded matrices were digested in 250 µl 0.2 M NaCl, 0.1 M NaAc, 0.01 M L-cysteine-HCl, 0.05 M EDTA-Na2 (pH 6.0) containing 16 U papain for 18 h at 65°C. To 100 µl papain digest, 100 ul 0.02 M Tris-HCl (pH 8.0) containing 0.1% sodium dodecyl sulphate was added. The mixture was incubated for 30 min at 60°C. A 100 µl aliquot was analyzed for total DNA by the addition of 1 ml Hoechst solution (0.2 µg Hoechst 33258/ml). The fluorescence of the samples was measured at an excitation of 365 nm and an emission of 458 nm. Calf thymus DNA was used as a standard.
The GAG content of the matrices was assessed by modification of the dimethylene blue method [Citation[18]]. Aliquots of 25 µl of the papain digest were assayed for GAG after the addition of 1.2 ml l,9-dimethyl methylene bule dye solution. The absorbance at 535 nm was measured with a spectrophotometer. To obtain the amount of GAG per sponge, the results were extrapolated from a standard curve using shark chondroitin sulfate.
For GAG and DNA analyses, the values of the unseed controls (n = 4) were subtracted from the seeded samples.
Scanning Electron Microscopy (SEM)
Samples were prepared for SEM, cell-seeded matrices (n = 2) were fixed in 0.1 M phosphate Buffer (pH 7.4) containing 2% (v/v) glutaraldehyde for at least 24 h at 4°C. Matrices were studied using a PHILIPS XL-30 environment scanning electron apparatus.
Histological
For light microscopy (LM), the cell-seed matrices were fixed in a 0.1 M phosphate-buffer solution (pH 7.4) containing 4% paraformaldehyde, dehydrated in alcohol and embedded with paraffin. Sections (7 µm) were stained with haematoxylin-eosin (HE).
Reverse Transcriptase (RT)-PCR
To obtain information about the differentiation status of chondrocytes in the cell-seeded matrices, mRNA levels of types I, II collagen were assessed using RT-PCR. On day 14, the chondrocytes were released from the scaffold using the papain solution and samples of the cell suspension were stored at −80°C for RNA isolation. Total cellular RNA was extracted from the chondrocytes using 1 ml of Trizol reagent. Collected cells were lysed with 1 ml Trizol solution, added with 200 µl chloroform, and centrifuged at 12,000 rpm for 15 minutes. The reverse transcription (RT) reaction was performed with 1 µg pure RNA. Isolated RNA was transcribed into cDNA with moloney-murine leukemia virus reverse transcriptase, and oligo(dT) 15 primers. cDNA was amplified using Taq DNA polymerase. The reaction contained 2.5 µl cDNA with 1 mmol/l MgCl2, 250 mmol deoxy nucleotide triphosphates (dNTPs), two units of Taq polymerase, and 1.25 mmol/l reverse primers in 1 × reaction buffer. The PCR products were electrophoresed in 2% agarose gels containing ethidium bromide. Glyceraldehyde 3-phosphate dehydrogenase (GAPDH) mRNA levels were used as an internal control.
The following primers were used in the amplification reactions. Rabbit type II pro-collagen: 5′-GCACCCATGGACATTGGAGG-3′, and the antisense primer 5′-AGCCCCGCACGGTCTTGCTT-3′; 323 bp. The sense primer for rabbit type I pro-collagen: 5′-CCGAAGGTTCCCCTGGACGA-3′, and the antisense primer 5′-CGCCCTGTTCGCCTGTCTCA-3′; 251 bp. For rabbit GAPDH: 5′-CGG AGC CAA AAG GGT CAT CAT-3′ for sense and 5′-TTT CTC CAG GCG GCA GGT CAG-3′ for antisense; 411 bp.
RESULTS
Matrix Characterization
Scanning electron microscopy revealed similar morphologies for the collagen (Col), collagen/chitosan (Col/Chi) and collagen/chitosan/HA (Col/Chi/HA) scaffolds and a cross-section of scaffolds showed interconnecting pores throughout the scaffolds, as well as on their surface (). The Col/Chi/HA scaffolds contained interconnected pores with diameters ranging from 80 to 150 µm and maintained their structural integrity during culture (). The physico-chemical characteristics of these scaffolds are shown in .
Figure 1 (a) SME micrograph of the porous collagen/chitosan/HA tri-copolymer scaffold. (b) SME micrograph of the porous collagen/hyaluronan/chitosan tri-copolymer scaffold incubation in DMEM medium after 21 days.
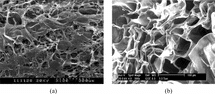
Table 1. Physico-chemical characteristics of various scaffolds
Proteoglycan and DNA Synthesis in the Scaffolds
Proliferation of, and extra-cellular matrix production by, chondrocytes were assessed by determination of the DNA content and GAG content between 3, 7, 14 and 21 days of culture in Col, Col/Chi, and Col/Chi/HA matrices. All matrices revealed a similar increase of the total amount of GAGs during the period. On day 21, the GAG-values in the scaffolds were 323.5 µg (±18.2) and 420.8 µg (±16.8) for Col and Col/Chi/HA scaffold, respectively. It appeared that the Col/Chi/HA scaffold contained significantly more (p < 0.01) newly synthesized GAGs than Col scaffold alone after 21 days in culture ().
Figure 2 (a) GAG content of chondrocytes in collagen, collagen/chitosan and collagen/chitosan/HA scaffolds as a function of time of culture. Results are expressed as µg GAG per scaffold. Values are mean ± SD (n = 4); (b) DNA content of chondrocytes in collagen, collagen/chitosan and collagen/chitosan/HA scaffolds as a function of time of culture. Results are expressed as µg DNA per scaffold. Values are mean ± SD (n = 4).
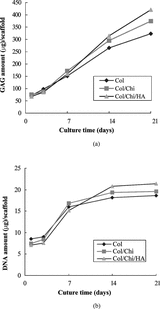
All matrices also revealed a gradual increase in the total amount of DNA during the culture period from the 3rd day to the 14th day, reaching a plateau after 14 days, indicating proliferation of chondrocytes. The higher DNA increase in the Col/Chi/HA scaffold than in the Col scaffold indicates a higher proliferation rate in the Col/Chi/HA scaffold ().
Histology
After 7 days of culture, cells at the Col/Chi/HA scaffold showed a round morphology (). This was confirmed by SEM (). The spherical morphology is indicative for the chondrocytic phenotype. The majority of the cells retained a round morphology during culture period. Environment scanning electron performed clearly revealed the clusters of Chondrocytes are present inside Col/Chi/HA matrices after 7 days cell-seeding (). After 21 days cell seeding, the porous matrices surface had almost completely been coated with cartilaginous tissue (, and ). The spherical morphology of the cells still could be detected within the matrix ( and ).
Figure 3 (a) Chondrocytes in Col/Chi/HA matrix 1 week after seeding. (b) chondrocytes in Col/Chi/HA matrix 3 weeks after seeding. H&E staining.
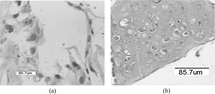
Figure 4 (a and b) Environment scanning electron micrographs in Col/Chi/HA scaffold, a week after cell seeding, and at two magnifications. Bar indicates 50 µm in a and 20 µm in b. (c and d) Environment scanning electron micrographs in Col/Chi/HA scaffold 3 weeks after seeding with chondrocytes. (d) Magnification from the same area as C.
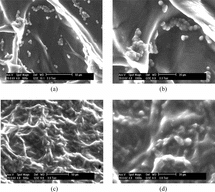
Reverse Transcriptase PCR
RT-PCR was performed against the collagen types I and II to confirm the preservation of the cartilage-specific phenotype of the cells during culture within the Col/Chi/HA scaffold. At 14 days, cells culture on the scaffold exhibited an intensive mRNA expression of collagen type II, and the collagen type I was detected hardly (), suggesting retainment of the chondrocytic phenotype for most cells.
Figure 5 Reverse transcriptase-polymerase chain reaction results against collagen types I and II of chondrocytes according to culture after 14 days within Col/Chi/HA scaffold. 1 = collagen type II and GAPDH; 2 = collagen I and GAPDH; M = 100 bp, 250 bp, 500 bp, 750 bp, 1000 bp and 2000 bp DNA ladder.
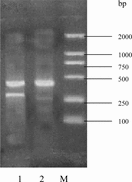
DISCUSSION
Collagen, chitosan and hyaluronan are natural biological materials, and as extracellular matrices of chondrocytes are thought to provide information for cell attachment, and have good biocompatibility. In this study, we prepared a tri-copolymer scaffold from collagen, chitosan and sodium hyaluronate. In the photos of SEM (), the pore size of collagen-chitosan-hyaluronan tri-copolymer is 80 ∼ 150 µm, and the porous structures were preserved well during culture periods.
Collagen was used as the scaffold material, which can aid chondrocytes proliferation, but the use of a collagen alone scaffold for cartilage defect repair has limitations due to its inherent poor mechanical stability and rapid biodegradation. Incorporation of chitosan into collagen scaffold improved its mechanical property and reduced the biodegradation rate against collagenase as demonstrated in .
Hyaluronan (HA) is a hydrophillic proteoglycan matrix component that provides a high binding water capacity as shown in ; the rich binding water capacity may have therefore influenced cell distribution and cluster formation, and preservation of chondrocytic. HA has been found to facilitate cell adhesion, cell proliferation, cell differentiation. Biochemical analysis also suggested that the Col/Chi/HA scaffold contained more DNA and GAG than collagen alone scaffold after culture period.
Reverse transcriptase PCR data on seeded cells from the scaffold indicated that the chondrocytes expressed cartilage-specific mRNA, as well as histology morphology observations, indicating the preservation of the chondrocytes phenotype for most cells in the Col/Chi/HA scaffold during culture period.
CONCLUSION
In this vitro study, results indicated that EDC crosslinked Col/Chi/HA tri-copolymer scaffold facilitated the formation of cartilaginous tissue, and may create an appropriate environment for culturing chondrocytes and for the generation of an engineered cartilage construct.
REFERENCES
- Johnson, L.L. (1989). Arthroscopic abrasion arthroplasty historical and pathological perspective: present status. Athroscopy 2: 54–69.
- Rehman, Q., Lane, N.E. (1999). Getting control of osteoarthritis pain. An update on treatment options. Postgrad. Med. 106: 127–134. [PUBMED], [INFOTRIEVE], [CSA]
- Minas, T., Nethrer, S. (1997). Current concepts in the treatment of particular cartilage defects. Orthopedics 20: 525–538. [PUBMED], [INFOTRIEVE], [CSA]
- Newman, A.P. (1998). Articular cartilage repair. Amer. J. Sports Med. 26: 309–324. [CSA]
- Langer, R., Vacanti, J.P. (1993). Tissue engineering. Science 260: 920–926. [PUBMED], [INFOTRIEVE], [CSA]
- Cao, Y., Vacanti, J.P., Paige, K.T. (1997). Transplantation of chondrocytes utilizing a human ear. Plast. Reconstr. Surg. 100: 297–302. [PUBMED], [INFOTRIEVE], [CSA]
- Hutmacher, D.W. (2000). Scaffolds in tissue engineering bone and cartilage. Biomaterial 21: 2529–2543. [CSA], [CROSSREF]
- Grande, D.A., Halberstadt, C., Naughton, G., Schwartz, R., Manji, R. (1997). Evaluation of matrix scaffolds for the tissue engineering of particular cartilage grafts. J. Biomed. Mater. Res. 34: 211–220. [PUBMED], [INFOTRIEVE], [CSA], [CROSSREF]
- Lu, L., Zhu, X., Valenzuela, R.G., Currier, B.L., Yazemski, M.J. (2001). Biodegradable polymer scaffolds for cartilage tissue engineering. Clin. Orthop. 391S: S251. [CSA]
- Bostman, O.M., Pihlajamaki, H.K. (2000). Adverse tissue reactions to bioabsorbable fixation devices. Clin. Orthop. 371: 216–227. [PUBMED], [INFOTRIEVE], [CSA], [CROSSREF]
- Zhang, Q.Q., Yao, K., Liu, L.R. (1999). Evaluation of porous collagen membrane in guided tissue regeneration. Artif. Cells Blood Substit. Immobil. Biotechnol. 27(3): 245–253. [PUBMED], [INFOTRIEVE], [CSA]
- Taravel, M.N., Domard, A. (1996). Collagen and its interaction with chitosan, III some biological and mechanical properties. Biomaterials 17: 451–455. [PUBMED], [INFOTRIEVE], [CSA], [CROSSREF]
- Francis Suh, J.K., Howard, W.T. (2000). Application of chitoson-based polysaccharide biomaterials in cartilage tissue engineering: a review. Biomaterial 21, 2589–2598. [CSA], [CROSSREF]
- Kawasaki, K., Ochi, M., Uchio, Y. (1999). Hyaluronic acid enhances proliferation and chondrocytes chondroitin sulfate synthesis in cultured embedded in collagen gels. J. Cell Physicol. 179: 142–148. [CSA], [CROSSREF]
- Solchaga, L.A., Yoo, J.U., Lundberg, M., Dennis, J.E., Huibregtes, B.A., Goldberg, V.M., Caplan, A.I. (2000). Hyaluronan-based polymers in the treatment of osteochondral defects. J. Orthop. Res. 18: 773–780. [PUBMED], [INFOTRIEVE], [CSA], [CROSSREF]
- Zhang, Q.Q., Liu, L.R., Ren, L., Wang, F.J. (1997). Preparation and characterization of collagen-chitosan composites. J. Appl. Polym. Sci. 64: 2127–2130. [CSA], [CROSSREF]
- Kim, Y.J., Sah, R.L., Doong, J.Y., Grodzinsky, A.J. (1988). Fluorometric assay of DNA in cartilage explants using Hoechst 33258. Anal. Biochem. 174: 168–176. [PUBMED], [INFOTRIEVE], [CSA], [CROSSREF]
- Farndale, R.W., Buttle, D.J., Barrett, A.J. (1986). Improved quantitation and discrimination of sulphated glycosaminoglycans by use of dimethylmethylene blue. Biochim. Biophys. Acta 883: 173–177. [PUBMED], [INFOTRIEVE], [CSA]