Abstract
In this work an amperometric glucose biosensor based on surface immobilization method was developed. Glutaraldehyde was used as cross-linker to establish the immobilization of glucose oxidase onto gelatin (carrier/coating reagent). In order to increase the porosity of coating material, immobilization media was further treated by polyacrylamide. Although this treatment increased the performance of biosensor to a large extent with respect to current densities obtained, it negatively affected the long-term stability. Our biosensor showed linear response in the physiological range of blood glucose (0.05 to 6 mM), had an acceptable response time (60 seconds) and was stable for 17 repeated usages in 51 days. We obtained best results with pH values very close to physiological pH and our biosensor could work efficiently in the tested temperature range 15 to 65°C.
1. INTRODUCTION
Biosensors are analytical systems that combine the specific properties of biologic and physicochemical compounds. Biosensor systems produced by using immobilized glucose oxidase as biological active material are called glucose biosensors.
Since in medical treatment of diabetic patients specific, accurate, simple and fast measurement of glucose levels in blood is vital, glucose biosensors are becoming more and more important.
Glucose oxidase (GOD) from Aspergillus niger is a robust and stable enzyme. The enzyme is composed of two polypeptide chains that are of approximately equal molecular weight and are held together by disulfide bonds. Two active sites (FAD) are entrapped by polypeptide chains. GOD is a flavine adenine dinucleotide FAD-based enzyme with a molecular weight of ca. 160,000. The redox center of GOD, i.e. FAD, is reduced by β-D-glucose as follows:
The H2O2 can be detected at the electrode surface in accordance with the equations:
The oxidation current of H2O2 is normally proportional to the concentration of glucose in the solution. Biosensor monitors either O2 consumption or H2O2 production.
In recent years the development of cheap and reliable enzyme electrodes for industrial and medical applications has become a major research area. Most widely used immobilization techniques in glucose biosensor production are entrapment or adsorption of enzyme in an electrochemically generated polymer matrix on the surface of electrode [Citation[1], Citation[2], Citation[4], Citation[7], Citation[8], Citation[9], Citation[11], Citation[14]] and enzyme immobilization on the surface of electrode, which was coated with a polymer or not [Citation[3], Citation[5], Citation[6], Citation[10], Citation[12], Citation[13]]. Depending on the polymer matrix most of the techniques gave fast response with low stability or slow response with high stability. Of course there are some exceptions of this general trend, such as the work reported by Xu, J.J. et al. [Citation[14]] (response in 2 seconds and stable up to 90 days) and the work reported by Singhal, R. et al. [Citation[1]] (response in 120 seconds and stable for 10 days). In this research we did not prefer to work with electrochemical polymerization method and tried to develop a simpler and cheaper procedure. When we focused on the surface immobilization techniques we saw that low stability is a common problem [Citation[3], Citation[6], Citation[10]] and we could not easily find reports about surface immobilization with high stability such as the work of Wang, B. et al. (stable up to 150 days) [Citation[13]].
In this work we aimed to develop a glucose-biosensor by coating its electrode with GOD immobilized gelatin (G). GOD was immobilized onto gelatin by cross-linking using glutaraldehyde.
Gelatin is a natural polymer that is obtained by the partial hydrolysis of collagen. The most characteristic physical property of gelatin and the basis for many of its uses is the ability of gelatin to form reversible elastic gels when its aqueous solutions are chilled. In the process of cooling, the viscosity of gelatin solutions increases sharply but there does not seem to be any particular point at which gelation occurs. Reactive groups present in gelatin are primarily hydroxyl, carboxyl, and amino functions. Their abundance is approximately 100, 75, and 50 milliequivalents (per 100 g high quality gelatin), respectively. Inorganic as well as organic compounds can harden gelatin. Among the former, which react in general with the carboxyl groups of the gelatin through their divalent metallic ions, the most often encountered are alum and chromium salts, which cross-link to the ionized carboxyl groups. Among the organic hardeners formaldehyde and glutaraldehyde are the most commonly used compounds. Hardening with aldehydes occurs by the formation of cross-links between the amino groups of gelatin. In our study gelatin was used as a carrier system and glutaraldehyde (GA) [OHC(CH2)3CHO] has been used as the hardener [Citation[15-17]].
In addition to this work, in order to obtain a better response from the biosensor a modification on the immobilization procedure was planned. Polyacrylamide (pAA) was added to the immobilization mixture as filling material and washed out in later stages to increase the porosity of the carrier system. Gelatin reacts and hardens with glutaraldehyde by making cross-linkages, whereas polyacrylamide does not harden under the experimental conditions used [Citation[18]]. These two facts were combined to develop a new immobilization procedure for glucose oxidase. By this modification a relative activity increase of 16% was achieved.
2. MATERIALS AND METHODS
2.1. Materials
Glucose oxidase (E.C.1.1.3.4), peroxidase (POD), o-dianosidine were purchased from Sigma Chemical Co. (USA). Granular photographic gelatin was obtained from Croda Gelatin Co. (UK). Polyacrylamide and glutaraldehyde was purchased from Aldrich Chemical Co. (UK). Glucose was purchased from Merck (Germany). Chemicals used in preparation of buffers were purchased from Sigma Chemical Co. (USA).
2.2. Methods
2.2.1. Preparation of Glucose Oxidase Electrode
Gelatin (0.075 g) was added to 0.05 M acetate buffer (pH 5.1) to obtain a final volume of 1 mL. The mixture was allowed to rest for 30 min at 25°C and then heated to 50°C in a water bath and cooled to 32°C after gelatin had been dissolved. Enzyme (10–50 U) was added to the immobilization solutions at 32°C. Solutions were stirred vigorously for 1 min then glutaraldehyde with concentrations varying from 0.002 to 0.015 M was added. Cross-linker addition solutions were then stirred again for 1 min to accelerate the reaction. Platinum plates (3 cm2) were drop-coated with 0.2 ml gel using 0.1 ml for each surface. The prepared electrodes were allowed to rest for 48 hours at 25°C. The electrodes were washed 3 times with acetate buffer to release the unbounded enzyme. To increase the surface area 0.004 M glutaraldehyde and the same amount of gelatin and glucose oxidase were used. Different amounts (0.050, 0.100, 0.125, 0.150, 0.200 pAA/G, g/g) of polyacrylamide were added to the solution. After cross linking was established, polyacrylamide was dissolved by washing with acetate buffer (pH 5.1). Washing operation was performed three times and each lasted for 1 h.
2.2.2. Determination of Glucose Oxidase Activity
The free enzyme and immobilized enzyme electrode activities were determined using chemical (spectrophotometric method) and electrochemical methods. In the chemical method, the activities were determined according to the Sigma Method using Jenway 6105 UV/Vis model spectrophotometer [Citation[19]].
One unit of enzyme was defined as the quantity of enzyme that catalyzes the reaction of 1 µmol glucose to produce gluconic acid in one minute at pH 5.1 at 35°C.
A hot water bath was used in order to adjust temperature of the reaction medium for temperature stability determination experiments. pH was adjusted between 4–9 with appropriate buffers for pH stability determination experiments.
% Relative activities were calculated according to the following formula:
Relative activity (ra): [activity of complex/(total activity of free enzyme used for coupling − activity loss by enzyme leakage)]
Maximum activity (ma): maximum value of ra in a series of experiments
% Relative activity: ra × 100
% Maximum activity: ra × 100/ma
2.2.3. Electrochemical Measurement of Glucose Oxidase Activity
Determination of glucose oxidase activity by amperometric method was accomplished by using a three-electrode system, consisting of the working enzyme electrode, a platinum counter electrode and an Ag/AgCl reference electrode. The current generated at working electrode was held at +0.6 V. All measurements were made at 25°C temperature in a cell containing acetate buffer. (0.05 M, pH 5.1) by using Potentio-Galvanoscan Wenking PGS 95 model (Germany) Potentio-Galvonoscan analyzer equipped with x-y-t recorder. Developed system was based on the measurement of H2O2 produced by the reaction.
3. RESULTS AND DISCUSSION
3.1. Enzyme Immobilization
Cross-linker glutaraldehyde concentrations were changed from 0.002 to 0.015 M and the highest relative activity was obtained with glutaraldehyde concentration 0.004 M as 61%. These results were verified by using amperometric analysis. Working electrodes were prepared with all of the cross-linker concentrations tested and results obtained are presented in . As seen in the figure both results were compatible and best values were obtained by using 0.004 M glutaraldehyde.
A negligible amount of leakage was observed in the enzyme leakage tests. Relative activities around 61% were obtained with this method before polyacrylamide treatment.
3.2. Activity Enhancement Using Polyacrylamide
Polyacrylamide was used as a temporary filling material. It was aimed to enhance diffusion by increasing the porosity of gelatin. Mixed polyacrylamide (which does not harden when used with glutaraldehyde under predefined immobilization conditions) was removed by washing at the end of the hardening process of gelatin. Different ratios of polyacrylamide-gelatin (0.050–0.200, g/g) were tested. As the ratio of polyacrylamide increased (up to 0.100 pAA/G (g/g)) a relative activity increase due to the enlarged surface area of the support system was obtained. After that ratio relative activity began to decrease. This fact was attributed (and confirmed by enzyme leakage tests) to the increased amount of enzyme that was not cross-linked and removed by dissolving polyacrylamide. Thus 0.100 pAA/G (g/g), was selected as optimum pAA/G (g/g) ratio. By this modification to immobilization procedure relative activity was increased to 71%.
Figure 1 Effect of glutaraldehyde (cross-linker) concentration on the response of glucose biosensor (10 U GOD, %7.5 G (g/mL)).
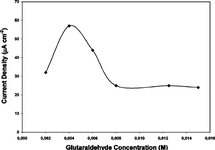
Working electrodes were prepared by coating platinum electrodes by immobilized GOD samples obtained with different ratios of pAA/G (0.050–0.200, g/g). Amperometric detections were performed and results are presented in .
As seen from the figure, maximum current densities were obtained with the same pAA-G ratio where maximum % relative activity was observed (0.100 (g/g)). Although maximums were the same, positive effect of pAA on electrochemical assay was more visible. A current density increase of 60% could be obtained with a pAA-G ratio of 0.100. As seen from and , with same glutaraldehyde concentrations (0.004 M) better yields could be obtained with PAA added samples.
3.3. Enzyme Loading
A series of electrodes containing different concentrations of GOD enzyme (0.5–40 U) were prepared by the modified immobilization method with a pAA-G ratio of 0.100 (g/g). Results are presented in .
Figure 2 Effect of pAA-G ratio on the response of glucose biosensor (10 U GOD, 0.004 M glutaraldehyde).
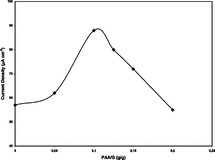
Figure 3 Effect of enzyme concentration on the response of glucose biosensor (pAA-G ratio 0.100 g/g, 0.004 M glutaraldehyde).
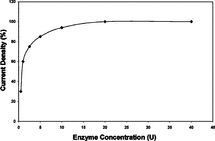
Figure 4 Effect of gel quantity on the response of glucose biosensor (10 U GOD, 0.004 M glutaraldehyde, pAA/G 0.100 g/g).
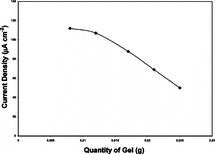
As seen from , current density increases above 10 U (up to 40 U) enzyme loading were only 6.4%, so 10 U enzyme was selected and used in the experiments as the enzyme quantity in immobilizations.
3.4. Gel Quantity
To investigate the effect of gelatin +pAA quantity used to coat the surface of electrode (3 cm2), a series of experiments were performed using 10 U enzyme. Results are given in .
As seen from , increasing gel quantity caused a smooth drop of current density between 0.0083 g to 0.0124 g and a fast drop after 0.0124 g. This result was expected because a current density drop due to thickening of coating material is a typical behavior. Considering current density yield and mechanical stability 0.0165 g gel quantity was selected to coat 3 cm2 electrode.
3.5. Calibration Curve
Calibration curve for the developed biosensor obtained for glucose levels 0.05 to 100 mM is presented in . We observed a linear response between 0.05 to 6 mM of glucose concentrations, which covers the physiological range of blood glucose. Our results fit well with the work published by Wu, Z. et al. [Citation[12]], in which GOD was immobilized to dimyristoylphosphatidylcholine (DMPG), tetrathiafulvalene (TTF) covered electrode with the aid of grafting polymer. When compared with electrochemically produced sensors with respect to linear range our results are better than Singhal, R. et al., Zheng, H. et al., Xu, J.J. et al. and Lleixa, C.P. et al. [Citation[1], Citation[2], Citation[4], Citation[11]], about the same with Fabiano, S. et al. [Citation[7]] and worse than Xue, H. et al. and Xu, J.J. et al. [Citation[8], Citation[14]]. Same comparison with sensors produced by chemical immobilization showed that we obtained better results than Counto, C.M. et al. and Campuzano, S. et al. [Citation[5], Citation[6]], about the same with Wu, Z. et al. [Citation[12]] and worse than Wang, B. et al. [Citation[13]].
Figure 5 Calibration curve of the developed glucose biosensor (10 U GOD, 0.004 M glutaraldehyde, pAA/G 0.100 g/g).
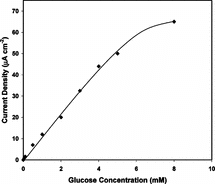
Steady state response time was about 60 seconds and 80% of steady state response was obtained within 40 seconds. Our response time was slow compared to Zheng, H. et al., Xu, J.J. et al., Fabiano, S. et al., Wu, Z. et al., Wang, B. et al., Xu, J.J. et al. [Citation[2], Citation[4], Citation[7], Citation[12], Citation[13], Citation[14]].
Interfering effect of uric acid and ascorbic acid was tested with coated and uncoated electrodes. Although immobilization decreased the interfering effects by 68% for uric acid and 42% for ascorbic acid, they still gave interference. So we propose the usage of nafion, which is a well known and widely used covering agent, which can remove the effect of interferants efficiently.
3.6. pH Stability
Our biosensor was tested in a pH range 4 to 9. Results are presented in . Maximum current densities were observed at pH 7 for both immobilization methods. Our best results fit with the results obtained by Fabiano, S. et al. and Wu, Z. et al. [Citation[7], Citation[12]] and closer to the physiological pH of human blood than the data presented by Zheng, H. et al., Counto, C.M. et al. and Lleixa, C.P. et al. [Citation[2], Citation[5], Citation[11]]. These results showed that our biosensor could be used very efficiently in blood glucose measurements.
3.7. Thermal Stability
Thermal stability of our sensor was investigated within a temperature range of 15 to 75°C. Results are presented in . Parallel results were obtained for both immobilization methods.
Figure 7 Thermal stability of glucose biosensor (10 U GOD, 0.004 M glutaraldehyde, pAA/G 0.100 g/g).
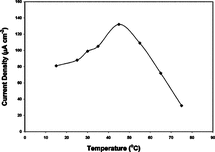
As seen from , we obtained maximum current density at 45°C, and our sensor seems to operate (of course calibration is required) well up to 65°C. Our results are suitable with Singhal, R. et al. and Counto, C.M. et al. [Citation[1], Citation[5]] and higher than Fabiano, S. et al. [Citation[7]] with respect to temperature of maximum activity. On the other hand, none of the investigators we analyzed gave information about stability of their sensor above 40°C except Counto, C.M. et al. [Citation[5]], who reported a serious drop after 42°C. With respect to stability in high temperatures, we can state that our sensor is the best among the reports we analyzed in this work.
3.8. Stability and Reusability of the Glucose Sensor
All of the electrodes prepared by immobilized samples could be used at least 17 times within a period of 51 days. Although current densities obtained by the sensor produced by pAA modified immobilization method were higher, this sensor retained only 70% of its activity after 51 days (stabilized after 7 usage). On the other hand, sensor produced with unmodified immobilization method retained almost all of its activity in 51 days of repeated usage. Results are presented in .
Figure 8 Stability and reusability of pAA-G and G coated glucose biosensors (10 U GOD, 0.004 M glutaraldehyde).
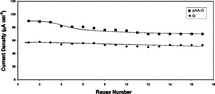
As seen from , our reusability results for unmodified method were as well as the results obtained by Wang, B. et al. and Xu, J.J. et al. [Citation[13], Citation[14]], and better than the results obtained by other investigators referenced in this work.
4. DISCUSSION
In this research it was aimed to develop a new biosensor to be used in medical treatment of diabetic patients and in food industry. Glucose oxidase was immobilized onto carrier gelatin by cross-linking. Glutaraldehyde was used as cross-linking agent. Applying polyacrylamide increased porosity of coating medium and increased current density by 60%. However, long-term stability was adversely affected by this modification. This negative effect was attributed to loss of the sticking power of gelatin to electrode surface due to pAA addition because of the positive (no leakage) results of leakage tests. A nafion cover over the gelatin coating may be helpful for this purpose, too. Finally, our glucose sensor can work in a wide range of temperatures, performs best for blood pH, has an acceptable response time (60 seconds), is very stable for the tested period (17 reuses within 51 days), and gives linear response within physiological range of blood glucose. Our method is cheap and simple, our reagents are cheap and our results are very promising. Compared to literature referenced in this work, our results are one of the best with respect to stability of the sensor. Some work has to be done about diminishing the effect of interfering materials, and we suggest nafion coverage.
The authors are grateful to Ankara University Research Fund (Project No. 2000-0705035) for financial support.
REFERENCES
- Singhal, R., Takashima, W., Kaneto, K., Samanta, S.B., Annapoorni, S., Malhotra, B.D. (2002). Langmuir-Blodgett films of poly(3-dodecyl thiophene) for application to glucose biosensor. Sensors and Actuators B: Chemical 86(1): 42–48. [CSA]
- Zheng, H., Xue, H., Zhang, Y., Shen, Z. (2002). A glucose biosensor based on microporous polyacrylonitrile synthesized by single rare-earth catalyst. Biosensors and Bioelectronics 17(6–7): 541–545. [PUBMED], [INFOTRIEVE], [CSA], [CROSSREF]
- Cosnier, S., Novoa, A., Mousty, C., Marks, R.S. (2002). Biotinylated alginate immobilization matrix in the construction of an amperometric biosensor: Application for the determination of glucose. Analytica Chimica Acta 453: 71–79. [CSA], [CROSSREF]
- Xu, J.J., Yu, Z.H., Chen, H.Y. (2002). Glucose biosensors prepared by electropolymerization of p-chlorophenylamine with and without nafion. Analytica Chimica Acta 463(2): 239–247. [CSA], [CROSSREF]
- Counto, C.M.C., Araujo, A.N., Conceicao, B.S.M., Rohwedder, M.J., Raimundo, I., Pasquini, C. (2002). Application of amperometric sol-gel biosensor to flow injection determination of glucose. Talanta 56(6): 997–1003. [CSA], [CROSSREF]
- Campuzano, S., Galvez, R., Pedrero, M., Villena, F.J.M., Pingarron, J.M. (2002). Preparation, characterization and application of alkanethiol self-assembled monolayers modified with tetrathiafulvalene and glucose oxidase at a gold disk electrode. J. Electroanal. Chem. 526(1–2): 92–100. [CSA], [CROSSREF]
- Fabiano, S., Tran-Minh, C., Piro, B., Dang, L.A., Pham, M.C., Vittori, O. (2002). Poly 3,4-etylenedioxythiophene as an entrapment support for amperometric enzyme sensor. Material Science and Engineering: C 21(1–2): 61–67. [CSA], [CROSSREF]
- Xue, H., Shen, Z., Li, Y. (2001). Polyaniline-polyisoprene composite film based glucose biosensor with high permselectivity. Synthetic Metals 124(2–3): 345–349. [CSA], [CROSSREF]
- Piro, B., Dang, L.A., Pham, M.C., Fabiano, S., Tran-Minh, C. (2001). A glucose biosensor based on modified-enzyme incorporated within electropolymerised poly(3,4-ethilenedioxythiophene) (PEDT) films. J. Electroanal. Chem. 512(1–2): 101–109. [CSA], [CROSSREF]
- Trojanowicz, M., Miernik, A. (2001). Bilayer lipid membrane glucose biosensors with improved stability and sensitivity. Electrochimica Acta 46(7): 1053–1061. [CSA], [CROSSREF]
- Lleixa, C.P., Jimenaz, C., Bartroli, J. (2001). Acrylated polyuretane-photopolymericmembrane for amperometric glucose biosensor construction. Sensors and Actuators B: Chemical 72(1): 56–62. [CSA], [CROSSREF]
- Wu, Z., Wang, B., Dong, S., Wang, E. (2000). Amperometric glucose biosensor based on lipid film. Biosensors and Bioelectronics 15: 143–147. [PUBMED], [INFOTRIEVE], [CSA], [CROSSREF]
- Wang, B., Li, B., Deng, Q., Dong, S. (1998). Amperometric glucose biosensor based on sol-gel organic-inorganic hybrid material. Anal. Chem. 70(15): 3170–3174. [PUBMED], [INFOTRIEVE], [CSA], [CROSSREF]
- Xu, J.J., Zhang, X.Q., Yu, Z.H., Fang, H.Q., Chen, H.Y. (2001). A stable glucose biosensor prepared by co-immobilizing glucose oxidase into poly(p-chlorophenol) at a platinum electrode. Fresenius J. Anal. Chem. 369: 486–490. [PUBMED], [INFOTRIEVE], [CSA], [CROSSREF]
- Sungur, S., Akbulut, U. (1994). Immobilisation of β-galactosidase onto gelatin by glutaraldehyde and chromium (III) acetate. J. Chem. Tech. Biotechnol. 59: 303–306. [CSA], [CROSSREF]
- Numanoglu, Y., Sungur, S. (2004). B-galactosidase from Kluyveromyces lactis disruption and enzyme immobilization using a cellulose-gelatin carrier system. Process Biochemistry 39(6): 705–711. [CSA], [CROSSREF]
- Kroschwitz, J.I., Howe-Grant, M. (1994). Kirk-Othmer Encyclopaedia of Chemical Technology, J. Wiley and Sons, Inc.: New York, 12, pp. 406–416.
- Kroschwitz, J.I., Howe-Grant, M. (1991). Kirk-Othmer Encyclopaedia of Chemical Technology, J. Wiley and Sons, Inc.: New York, 1, pp. 266–287.
- Sigma Chemical Co., Technical Bulletin 1983, No. 510.