Abstract
In order to prepare a biosensor for the determination of xanthine, electropolymerization of pyrrole on Pt surface was carried out with an electrochemical cell containing pyrrole, ferrocene (as a electron mediator) and tetrabutylamonium tetrafluoroborat in acetonitrile by cyclic voltammetry between 0.0 and 0.9 V (vs SCE) at a scan rate of 50 mV/s upon Pt electrode. Xanthine oxidase was immobilized by a glutaraldehyde/bovine serum albumin (BSA) crosslinking procedure on to polypyrrole film after the electropolymerization processes. The response of the biosensor against xanthine was measured after 3–4 min following the application of a constant potential of +0.7 V (vs SCE). The resulting biosensor exhibits excellent electrocatalysis for the xanthine. The amperometric determination is based on the electrochemical detection of H2O2, which is generated in enzymatic reaction of xanthine. The effect of various experimental conditions was examined for the determination of the analytical performance. The sensor responds to xanthine with a detection limit of 1.0 × 10−6 M. The response current increases linearly with xanthine concentration up to 4.0 × 10−4 M. The sensor remains relatively stable for 45 days.
INTRODUCTION
Xanthine is an intermediate of the purine metabolism, and is produced after adenosine triphosphate decomposition. The physiological conversion of xanthine by Xanthine oxidase is of increasing medical interest [Citation[1], Citation[2]]. In addition, it is determined as an estimate of the freshness of fish [Citation[3], Citation[4]]. Xanthine oxidase is a flavoprotein that contains molybdenum, nonheme iron and labile sulfur and which can catalyze the oxidation of xanthine to uric acid:
An excess of uric acid in human blood will result in gout and uric acid deposits in kidneys. Xanthine, an important endogenous molecule, can be detected by various methods, including amperometric biosensors [Citation[5-7]].
The thought for the construction of biosensor stemmed from the reaction of xanthine to give hydrogen peroxide as given above with the presence of xanthine oxidase [Citation[8]]. It is a well-known fact that hydrogen peroxide is oxidized as follows at a certain potential on Pt electrode [Citation[9], Citation[10]].
If a potential enough to create the above reaction is applied to the electrode, the anodic current passing from the circuit is proportional with the amount of hydrogen peroxide formed and thus the concentration of xanthine [Citation[5], Citation[8]]. In recent years, mediators and conducting polymers have been used as a matrix for immobilizing the enzymes [Citation[11], Citation[12]]. The mediators are also known to have an important role in biosensors [Citation[13]]. Although the working principles of the biosensor constructed by the immobilization of xanthine oxidase enzyme in a polypyrrolle film containing ferrocene are based upon the same reactions, there is no study found in the literature where ferrocene was used a mediator for xanthine biosensors.
Therefore, the purpose of this study was to develop a biosensor incorporating immobilized xanthine oxidase and ferrocene for the determination of xanthine. The optimum working conditions with respect to the buffer and substrate concentrations, the pH and temperature were investigated.
MATERIAL AND METHODS
Equipment and Reagents
The electrochemical studies were carried out using BAS 100 B/W electrochemical analyzer using a three-electrode cell. The working electrode was a Pt plate (0.5 cm2). The auxiliary and the reference electrodes were a Pt wire and saturated calomel electrode (SCE), respectively. The pH values of the buffer solutions are measured with ORION Model 720A pH/ionmeter. Temperature control was achieved with Grant LT DGG thermostat.
Xanthine oxidase (EC 1.1.3.22, purified from the microorganism and with an activity of 2.5 unit.ml−1) and xanthine were purchased from Sigma. Pyrrole and ferrocene was supplied from Fluka, tetrabutylammonium tetraflouroborate from Aldrich and acetonitrile from Merck. All other chemicals were obtained from Sigma. All the solutions were prepared by the use of bi–distilled water.
Preparation of Xanthine Biosensor
The surface of the Pt plate electrode cleaned according to [Citation[14]] was covered with polypyrrole by the electropolymerization of pyrrole [Citation[12], Citation[15]]. The electrode was immersed in a 5 mL solution of 0.2 M pyrrole, 0.1 M tetrabutylammonium tetraflouroborate, 10 mM ferrocene in acetonitrile. The solution was purged with nitrogen in order to remove the oxygen. The electropolymerization of pyrrole upon the electrode surface was achieved by the cyclic voltammetric scans between 0.0 and + 0.9 V at a scan rate of 50 mV/s. The electrode was washed with acetonitrile and then buffer solution after the coating process.
100 µL xanthine oxidase enzyme (2.5 Unit /mL), 1 mg bovin serum albumin, 50 µL 0.025 M phosphate buffer at a pH of 7.4 and 25 µL 5% glutaraldehyde was dropped upon platinium/polypyrrole-ferrocenium (Pt/PPy-Fc) electrode. The electrode was dried at room temperature, washed with buffer solution (pH 7.4 0.025 M phosphate buffer) several times in order to remove the non-immobilized excess enzyme and glutaraldehyde. The electrode was kept in a refrigerator at +4°C in phosphate buffer when it was not in use.
Amperometric Measurements
In order to determine whether the enzyme electrode was sensitive to xanthine, an aqueous buffer solution containing 0.025 M phosphate and 0.1 M lithium perchlorate at a pH of 7.4 was added to the cell. The solution was purged with oxygen for ten minutes and a steady-state background current was allowed to decay at a constant value at +0.7 V. Then the aliquots xanthine stock solution was added to the cell using a micropipette, and stirred for 15 seconds. The response of the biosensor against xanthine was measured after 3-4 min following the application of a constant potential of +0.7 V (vs SCE). The cell was purged with oxygen for one minute prior to each addition of xanthine into the cell. The current values against xanthine concentration were plotted in order to determine the working range of the electrode.
RESULTS AND DISCUSSION
In this study a new xanthine sensitive amperometric enzyme electrode was prepared by the use of ferrocene as mediator. The parameters effecting to the performance of the biosensor were investigated.
The Effect of Oxygen
Since the reaction of xanthine in the presence of xanthine oxidase takes place in an oxygenated media, the solution was purged with oxygen for certain periods to keep the oxygen concentration at certain value. In order to investigate the effect of oxygen passage time the calibration plots prepared the solutions not purged with oxygen and the solution purged with oxygen for 5, 10 and 15 minutes were drawn and given in .
Figure 1 The effect of oxygen passage time upon the sensitivity of biosensor to 1.5 × 10−4 M xanthine in 0.025 M phosphate buffer (pH 7.4) at 25°C. (a): without the passage of oxygen (b): 5 minutes of oxygen passage, (c): 10 minutes of oxygen passage, (d): 20 minutes of oxygen passage.
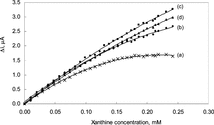
As seen from the figure, the linear working range is highly narrow when the solution was not purged with oxygen. This was attributed to the fact that since the amount of hydrogen peroxide, which forms as a result of enzymatic reaction, is dependent on the amount of oxygen present; the amount of oxygen in the test solution was not sufficient enough for the completion of the enzymatic reaction.
Although the working range increases with the time of passage of oxygen, it was observed that the sensitivity was decreased for the oxygen passage time of 20 minutes at xanthine concentrations above 1.5 × 10−4 M. Serge Cosnier explained this by the fact that the excess oxygen in the solution inhibits the electron transfer during the reduction of FAD into FADH2 [Citation[16]]. Therefore, it was decided that the best time for passage of oxygen was 10 minutes.
Response Time
The amperometric response time of the enzyme electrode to xanthine was determined at three different xanthine concentrations. The current differences for 1.0 × 10−5, 5.0 × 10−5 and 1.0 × 10−4 M xanthine against time was plotted and given in . The figure shows that the response time is shorter at lower concentrations than at higher concentrations. The response time can be taken as 3–4 minutes where the currents are approximately constant. Since the curves are parallel to each other, the measurements can be taken before 3–4 minutes provided that that they are made exactly at the same times (i.e., after 50 seconds). All the parameters were investigated basing upon the measurements taken after 4 minutes. These times are highly suitable for biosensor response [Citation[17]].
The Effect of pH
The effect of solution pH on the xanthine response at the proposed biosensor is shown in . The amperometric response is enhanced by increasing the solution pH in the range of pH 7.0 to 7.4 at a constant xanthine concentration of 5.0 × 10−5 M. The graph shows that the best pH for xanthine oxidase is 7.4. This value is similar to those of solution phase enzyme [Citation[18]] and also in good compliance with most of the data reported in literature [Citation[8]]. In xanthine determination with biosensors, pH values other than 7.4 were employed in literature (pH 8.0 and 9.0). This was attributed to the fact that the used polymer and the type of immobilization were different [Citation[16], Citation[19]].
Figure 3 The effect of solution pH on the response to 5.0 × 10−5 M xanthine in 0.025 M phosphate buffer solution at 25°C. The operating potential is +0.7 V vs SCE.
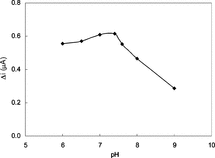
Therefore, pH 7.4 is selected by considering both the sensitivity and the stability of the biosensor and consequently further studies were performed at pH 7.4.
The Effect of Temperature
The amperometric response of the biosensor prepared was investigated at different temperatures using constant xanthine concentration of 5.0 × 10−5 M, .
Figure 4 The effect of temperature upon the sensitivity of biosensor against xanthine (0.025 M pH 7.4 phosphate buffer).
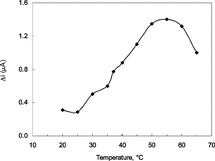
As seen from the figure, the current difference increases with temperature up to 55°C and decreases afterwards. The highest current difference was obtained at 55°C. The enzyme is thought to be denaturated after this temperature. The study was carried out at 25°C due to the difficulties involved in working at 55°C. In addition, the linearity of the calibration curves plotted at 55°C was not satisfactory (R2 = 0.745). Similar situations were encountered with other enzyme electrodes in literature [Citation[10]]. Using the data obtained between 20–55°C, 1/T–lnΔi graph was plotted and the slope of the curve gave the activation energy of the xanthine oxidase catalyzed reaction of xanthine into uric acid as 22.9 kJ/mol.
The Effect of Phosphate Concentration
The amperometric response of the biosensor was monitored changing the phosphate concentration of the phosphate buffer used in the study at a pH value of 7.4 pH with a constant xanthine concentration of 5.0 × 10−5 M. The response of the biosensor was plotted against the phosphate concentration (). The figure revealed that the best response was obtained in a buffer solution with phosphate concentration of 0.025 M. Above or below this concentration the response was found to show a significant decrease. It can be concluded that the buffering capacity of the solution was decreased at lower phosphate concentration. At higher phosphate concentration, on the other hand, the conductivity of the polypyrrole films was decreased as a result of the replacement of the negativity charged ions in the structure of the film with phosphate ions. These results were found to be in good compliance with the similar studies in literature [Citation[20]].
The Effect of Amount of Enzyme
The effect of enzyme concentration upon the response of the biosensor prepared by the immobilization of the enzyme upon the surface of Pt/PPy-Fc electrode was also investigated. The response of the biosensor after immobilization of seven different enzyme concentrations at pH 7.4 and xanthine concentration of 5.0 × 10−5 M was measured and the resulting data were plotted in . The highest current for xanthine concentration of 5.0 × 10−5 M was obtained with an enzyme concentration of 1.02 units.
Substrate Concentration and Calibration Curves
In order to determine the linear working range of the xanthine biosensor prepared, the current values obtained for solutions containing 1.0 × 10−5 − 5.0 × 10−3 M xanthine were recorded ().
Figure 7 The effect of xanthine concentration upon the amperometric response of the biosensor (0.025 M pH 7.4 phosphate buffer, 25°C).
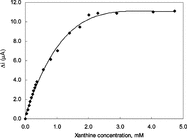
There are two linear parts in the region up to 2.2 × 10−3 M ranging between 1.0 × 10−5 − 4.0 × 10−4 M (R2 = 0.998) and 4.0 × 10−4 − 2.2 × 10−3 M (R2 = 0.986). The graph for the lower concentration range is given in . It has been shown that the linearity of this graph is highly satisfactory and it can be used for the quantitative determination of xanthine. The low detection limit of the biosensor prepared was found to be 1.0 × 10−6 M. The figure shows that the current remains constant at xanthine concentrations above 2.2 × 10−3 which shows that xanthine oxidase enzyme was saturated with xanthine. The KM constant, a specific parameter of enzymes, was computed by the use of 1/[xanthine])-1/Δi graph (Lineweaver–Burke plot). KM and Vmax values were found as 1.33 mM and 2.75 µA/minute, respectively. The KM value that shows the affinity of the biosensor was 1.33 mM and the values cited in literature were 7 mM, 1.11 mM and 0.04 mM [Citation[8], Citation[19], Citation[20]]. Since the enzymatic reactions take place through a carrier medium the affinity of the enzyme towards the subrate is not the same in every carrier media. Therefore it is normal that KM values differ.
The Reproducibility of the Enzyme Electrode
In order to test the reproducibility of the enzyme electrode prepared the current changes obtained after subsequent usage were plotted against the number of measurements. The relative standard deviation obtained after 15 measurements at a constant xanthine concentration of 5.0 × 10−5 M was found to be 6%. In another reproducibility test three calibration curves were plotted by the use of the same electrode. The relative standard deviation was found to be 1.4% from the slopes of these three curves. This shows that the reproducibility of the xanthine electrode was highly satisfactory.
The Storage Stabilization of the Enzyme Electrode
The response of the enzyme electrode prepared under optimum conditions was measured for a period of 45 days at constant xanthine concentration (5.0 × 10−5 M). The result of 15 measurements during this period is plotted in .
There was no noticeable decrease in the response during the first ten days. There was a rapid decrease in current values between 10th and 20th days and there was not a significant change after the 30th day. The electrode showed 35% of the initial amperometric response at the end of the 45th day. This indicates that the biosensor prepared can be used for quite a long time. The properties and the optimum working conditions of the biosensor prepared are summarized in .
Table 1. The properties and the optimum working conditions of the biosensor
The properties and the preparation conditions of the biosensors made for the determination of xanthine in literature are summarized in in order to compare the data obtained in our study in literature.
Table 2. The properties of xanthine biosensors literature
In conclusion, the xanthine biosensor prepared in this study:
Is useable in a large concentration range (1.0 × 10−5 − 4.0 × 10−4 M).
Has a very low detection limit (1.0 × 10−6 M).
Has an acceptable response time for a biosensor (t95 = 3–4 min).
Gives highly reproducible results (the relative standard deviation is 6% after 15 measurements, the standard deviation obtained from the slopes of the calibration curves is 1.4%).
Has a satisfactory storage stabilization (gives the 95% after 10 days and the 35% of the initial amperometric response at the end of the 45th day).
The KM and Vmax values of xanthine oxidase enzyme immobilized in polypyrrole–ferrocene film were 1.33 mM and 2.75 µA/minute, respectively.
It is easy to prepare and highly cost effective.
It can conveniently be concluded that the biosensor prepared in this study can easily be employed for xanthine determination in biological samples.
REFERENCES
- Richter, T., Shultz-Lockyear, L.L., Oleschuk, R.D., Bitewski, U., Harrison, D.J. (2002). Bi-enzymatic and capillary electrophoretic analysis of non-flurescent compounds in microfluidic devices determination of xanthine. Sensor. Actuat. B. 81: 369–376. [CSA]
- Gunnett, C.A., Heistad, D.D., Berg, D.J., Faraci, F.M. (2000). IL-10 deficiency increases superoxide and endothelial dysfunction during inflammation. Am. J. Physiol. 279: H1555–H1562. [CSA]
- Hu, S., Liu, C.-C. (1997). Development of a hypoxanthine biosensor based on immobilized xanthine oxidase chemically modified electrode. Electroanal. 9: 372–377. [CSA], [CROSSREF]
- Cayyuela, G., Pena, N., Reviejo, A.J., Pingarron, J.M. (1998). Development of a bienzymatic graphite-teflon composite electrode for the determination of hypoxanthine in fish. Analyst 123: 371–377. [CSA], [CROSSREF]
- Luong, J.H.T., Male, K.B., Masson, C., Nguyen, A.L. (1992). Hypoxanthine ratio determination in fish extract using capillary electrophoresis and immobilized enzymes. J. Food Sc. 57: 77–81. [CSA]
- Mulchandani, A., Male, K.B., Luong, J.H.T. (1990). Development of abiosensor for assaying postmortem nucleotide degradation in fish tissues. Biotechno. Bioeng. 35: 739–745. [CSA], [CROSSREF]
- Hlavoy, J., Haemmerli, S.D., Guilbault, G.G. (1994). Fibre-optic biosensor for hypoxanthine and xanthine based on a chemiluminescence reaction. Biosens. Bioelectron. 9: 189–195. [CSA], [CROSSREF]
- Pei, J., Li, X.-Y. (2000). Xanthine and hypoxanthine sensors based on xanthine oxidase immobilized on a CuPtCl6 chemically modified electrode and liquid chromatography electrochemical detection. Anal. Chim. Acta. 414: 205–213. [CSA], [CROSSREF]
- Umana, M., Waller, J. (1986). Protein-modified electrodes. The glucose oxidase/polypyrrole system. Anal. Chem. 58: 2979–2983. [CSA], [CROSSREF]
- Gülce, H., Özyörük, H., Çelebi, S., Yıldız, A. (1995). Amperometric enzyme electrode for aerobic glucose monitoring prepared by glucose oxidase immobilized in poly(vinyl ferrocenium). J. Electroana. Chem. 394: 63–70. [CSA], [CROSSREF]
- Gerard, M., Chavbev, A., Malhotra, B.D. (2002). Application of conducting polymers to biosensors. Biosens. Bioelectron. 17: 345–359. [PUBMED], [INFOTRIEVE], [CSA], [CROSSREF]
- Reiter, S., Habermüller, K., Shuhman, W. (2001). Areagentless glucosebiosensor based on glucose entrapped into osmium-complex modified polypyrrole films. Sensos. Actuat. B. 79: 150–156. [CSA], [CROSSREF]
- Chavbey, A., Malhotra, B.D. (2002). Mediated biosensors. Biosens. Bioelectron. 17: 441–456. [CSA], [CROSSREF]
- Gros, P., Durliat, H., Comtat, M. (2000). Use of polypyrrole film containing Fe(CN)63− as pseudo-reference electrode: application for amperometric biosensors. Electrochim. Acta. 46: 643–650. [CSA], [CROSSREF]
- Chaubey, A., Gerard, M., Singhal, R., Singh, V.S., Malhotra, B.D. (2000). Immobilization of lactate dehydrogenase on electrochemically prepared polypyrrole-polyvinylsulphonatecomposite films for application to loctate biosensors. Electrochim. Acta. 46: 723–729. [CSA], [CROSSREF]
- McKenna, K., Brajter-Toth, A. (1987). Tetrahiafulvane tetra-cyanoquinodimethane xanthine oxidase amperometric electrode for the determination of biological purines. Anal. Chem. 59: 954–962. [PUBMED], [INFOTRIEVE], [CSA], [CROSSREF]
- Telefoncu, A., Biyosensörler, Biyokimya, Lisansüstü, Yaz, Okulu, Haziran. (1999). 20–26 Ege Üniversitesi, Kuşadasι, 136–137. [PUBMED], [CSA]
- Arai, G., Takahashi, S., Yasumori, I. (1996). Xanthine and hypoxanthine sensors based on xanthine oxidase immobilized in poly(mercapto-p-benzoquinone) film. J. Electroanal Chem. 410: 173–179. [CSA], [CROSSREF]
- Kilinc, E., Erdem, A., Gokgunec, L., Dalbasti, T., Karaoglan, M., Ozsoz, M. (1998). Butternilk based cobalt phthalocyanine dispersed ferricyanide mediated amperometric biosensor for the determination of xanthine. Electroanal. 10: 273–275. [CSA], [CROSSREF]
- Adeloju, S.B., Shaw, J.S., Wallace, G.G. (1996). Polypyrrole-based amperometric flow injection biosensor for urea. Anal. Chim. Acta. 323: 107–113. [CSA], [CROSSREF]
- Llaudet, E., Botting, N.P., Crayston, J.A., Dale, N. (2003). A tree-enzyme microelectrode sensor for detecting purine release from central nervous system. Biosensors and Bioelectronics 18: 43–52. [PUBMED], [INFOTRIEVE], [CSA], [CROSSREF]