Abstract
For pilot-scale manufacturing of hemoglobin-based oxygen carrying drugs, we should get highly pure and viral inactivated hemoglobin (Hb) at high recovery. In our method, placenta hemoglobin (PHb) solutions were purified by heating in the presence of reducing agent and deoxygenating conditions so that heat-sensitive proteins were selectively precipitated and virus was inactivated. The optimum preparative condition resulted in highly purified PHb solution (>99% pure) with approximate 90% recovery and less than 2% of MetHb content, maintained oxygen carrying capacity, residual phospholipids less than 1 ppm, free of endotoxin, bacteria, type A&B antigens and virus. Finally, we compared the efficacy of blood exchange on rat with poly-PHb and poly-Hb from adult blood. The results showed no significant difference between two products. Therefore, the placenta Hb obtained from this method could be supplied as materials for oxygen carrying drugs.
INTRODUCTION
Hb-based oxygen carrying drugs have been developing since 1934 and a variety of products have been developed using human blood or animal blood [Citation[1-3]]. As the starting materials, Hb are mainly supplied from human outdated RBC component, animal sources (bovine blood, ovine blood, porcine blood, et al.) and transgenically produced Hb [Citation[16]]. Although the current blood sources could provide large amount starting materials, there are many disadvantages [Citation[12-15]]: (1) Because of the shortage of donors and increasing demand for fresh blood in clinical setting, less and less outdated blood could be supplied (2) Hb from animal sources is probably contaminated with proteins, such as antibodies, which could cause immune system response in the recipient, at the same time animal-borne infectious diseases are another problem; (3) Transgenically produced Hb is usually associated with low yield and poor purity. So we choose placenta blood as the alternative source, which has proved to have some advantages; for example, it utilizes the blood discarded in the past and it allows transporting more O2 to the hypoxia tissues for the different types of Hb in the placenta blood that have higher O2 affinity.
Donated blood has been used in clinical studies for a long time and many methods have been developed to detect and pathogenically remove agents from it. However, virus contamination of the donated blood is still a major concern [Citation[4]]. Today, donated blood is tested for the presence of well-known viruses, including hepatitis B and C, HIV1/2, and HTLV I/II as required by government health agencies. However, current blood screening does not test for a variety of other potential pathogenic agents, including herpes viruses such as cytomegalovirus or Epstein-Barr virus, parvoviruses, bacteria or parasites, such as Yersinia or Trypanosoma cruzi, respectively, or poorly characterized pathogens, such as those responsible for Creutzfeld-Jakob disease [Citation[5]]. To eliminate the risk of viral contamination, many methods have been developed for viral inactivation. Pasteurization was invented in 1856 [Citation[6]], and had been employed in many drugs' viral inactivation. The current methods, however, generally require sophisticated equipment and are associated with very high cost. In recent years, heat treatment has been successfully applied on the inactivation of a number of virus including HIV, Porcine Parvovirus (PPV) and Bovine Viral Diarrhea Virus (BVDV) [Citation[7-8]]. More viruses, such as Encephalomyocarditis Virus (EMC), Pseudorabies Virus (PSR), and Simian Virus (SV40), were inactivated in the studies of Harry et al. [Citation[9]]. In addition, Estep et al. pointed out that heat treatment showed significant inactive effect on Sindbis Virus, Polio Virus, and Pseudorabies Virus [Citation[10]]. Therefore, heat treatment was demonstrated to be a simple and promising technology for viral inactivation.
At present, most scientists adopt chromatograms to purify Hb, which need expensive instruments and chromatogram medium. In order to remove potential contaminated viruses, several viral inactivation steps have to be added to the purification process, which would further increase the complexity of the purification process and overall cost. In this study, we developed a simple and effective method to achieve a high quality of purified Hb at a low cost. The instruments for heat treatment were cheap and easy to sterilize and depyrogen. During this heating-based purification process, impurities were denatured to precipitation, and the virus was inactivated successfully.
MATERIALS AND METHODS
Preparation of Purified and Viral Inactivated Hb
Preparation of Hemolysate. Placenta blood was collected from abandoned placentas after babies were born. The procedure was composed of washing collected blood with 0.9% (W/V) NaCl solution and centrifugation to separate the plasma and white cells from red blood cells (RBCs). After suspending the isolated RBCs in hypotonic phosphate buffer solutions, the mixture was stirred for hemolyzing. Then substantially cell-free hemolysate was obtained.
Purification and Viral Inactivation. Before heating, the hemolysate was adjusted to pH6.00–6.50, then the Hb kept was in deoxy form by exposure to vacuum and bubbling with inert gas (2L/min); additionally, reducing agent was added. After added protective agent, the mixture was heated to 60 ± 1°C over 30 minutes, held at 60 ± 1°C for 10 ∼ 12 hours, and cooled to 4°C. Then 0.5% (W/V) activated charcoal was added, and the mixture was centrifugated to separate the top layer and filtered with 0.45 µm micron membrane. The filtered material was ultrafiltrated against physically salt solution by LabscaleTM TFFsystem (Millpore) containing membrane cut off molecular weight below 10 kD. The procedure was carried out at 4°C with inert gas to prevent MetHb formation. After that, a 0.22 µm micron filtration might be employed, and the pH of filtrate was adjusted to 7.4 to yield the final purified and viral inactivated PHb solution.
Preparation of Poly-PHb
Above purified Hb solution was firstly adjusted to 10 gm/dl by ultrafiltration and modified with pyridoxal phosphate (PLP) [Citation[17]]. Cross-linked Hb was prepared by the reaction between glutaraldehyde (GDA) and Hb at 4°C for 90 minutes in phosphate buffer solution (PBS), excess amount of lysine was added to inactivate the unreacted GDA, the reacted mixture was ultrafiltrated with PBS by system containing membrane cut off molecular weight below 100 kD to remove uncross-linked hemoglobin and redundant GDA and lysine. Finally, the filtrated solution was performed through GPC column, and Poly-PHb was collected according to the molecular weight. Details will be discussed in other articles.
Analytical Methods
Spectroscopic Assay. The concentration of Hb was determined by conversion of Hb into cyanmethemoglobin and detecting the absorption at 540 nm [Citation[18]]. The percentage of MetHb was determined by the absorption of 630 nm according to the method of Evelyn 3(b)[Citation[19]]. The percentage of recovery was based on grams of PHb recovered, from hemolysate to final product.
Electrophoresis Assay. The purity of PHb was determined by native polyacrylamide gel electrophoresis (PAGE) and SDS-PAGE. After electrophoresis, proteins were stained with Coomassie Brilliant Blue R-250 and analyzed by ChemiImagerTM 5500 systems (Alpha Innotech).
HPLC Assay. The HPLC assay was also carried out for determining the purity of PHb. The analysis was conducted with an instrument equipped with TSK3000 SW column (30 cm × 7.8 mm, TOSOH) and HPLC system (VARIAN). The mobile phase was 0.1 M PBS + 0.1 M NaCl, flow rate was 0.5 ml/min and the detection wave-length was 280 nm. The data was analyzed by Star Chromatography Workstation (VARIAN).
Residual Phospholipids Detection. Samples for analysis of phospholipids were prepared from 5gm/dL purified PHb solution, and performed with Strong and Koch's method [Citation[22]].
Endotoxin Determination and Type A&B Antigens Test. Tests were conducted by using standard methods described in the China Pharmacopoeia 2005.
Cell Debris Detection. The samples were 5gm/dl hemolysate and purified PHb solution, which were detected with CK40-32PH inverted microscope (Olympus).
Assay of Biological Activity. Biological activity assays included all-wave scan and oxygen carrying capacity determination. All-wave scan was conducted using CARY50 UV-Visible spectrophotometer (VARIAN). The absorption spectra of samples (hemolysate and purified PHb) and standard (human adult Hb, Sigma) were recorded at 200 ∼ 1100 nm. Another method to determine the biological activity was performed to investigate the P50 as compared to whole blood, which was determined by ABL555 blood gas analyzer (RADIOMETER).
Animal Experiments
All animals used in the experiments were handled according to national standards. Anesthetized Sprague-Dawley rats weighing 240 ∼ 260 g were used to evaluate the effect of poly-PHb. About 70% of total blood volume was involved in blood replacement. Blood was slowly withdrawn at the rate of 0.5ml/min, and immediately thereafter the same volume of poly-PHb was infused at the same rate. The results were compared with that of poly-AHb. After the completion of infusion, rats were returned to separate cages and monitored for 24 hours. Those rats that lived for 24 hours were considered as having “survived.” The experiments were operated with the assistance of the national standard anti-shock laboratory (Institute of Surgery Research of Third Military Medical University).
RESULTS AND DISCUSSIONS
Purification Procedure
Influence of Hemolytic Time on Hemolysate
The first step in preparation was to produce a crude hemolysate. Hemolytic data from four separate experiments were shown in . It suggested that most PHb (90%) was tended to release within 30 minutes. Regarding the denaturation caused by mechanical stir and consistent MetHb formation when time went by, 30 minutes were chosen as the most suitable hemolytic time.
Influence of Deoxy Methods
As we know, deoxy-Hb was more heat-stable than oxy-Hb and heating at 60°C will induce denaturation of oxy-Hb and has no effects to the deoxy form of Hb. To further stabilize PHb during the heating process, Hb in the hemolysate obtained from the previous steps was first converted to the deoxy form by exposing the hemolysate to vacuum and bubbling with inert gas. The comparisons of three deoxy methods were shown in . We could see that the highest yield and least MetHb formation were obtained by the method mentioned above, so it was more efficient to induce PHb in deoxy form and make the loss minimum. Also, conversion of oxy-Hb to HbCO was usually one characteristic point for stabilizing Hb in previous studies [Citation[11]]. However, toxic gas carbon monoxide (CO) would be a potential danger for workers and it might be difficult to manipulate in pilot-scale Hb purification. Furthermore, conversion of HbCO to oxy-Hb after heat treatment would increase the running cost, therefore it was not suitable in our method.
Influence of Reducing Agents
Apart from the physical method, the reducing agent was also adopted to convert Hb into deoxy form. Two agents that were widely used were investigated: ascorbic acid and reduced glutathione. Different recovery resulted from different agent were exhibited in (a). Data indicated that introducing ascorbic acid into the system yields a higher recovery; this could be explained by its potency in both reducing oxyHb and removing O2. However, reduced glutathione gave an intermediate level of protection for it induced Hb deoxygenation during, but not prior to heating, so the residual oxyHb would have denatured before it worked. Another reason might be that reduced glutathione was heat unstable, most of which was denatured at 60°C, where ascorbic acid was stable. From (b), it is noted that less MetHb formation when introduced ascorbic acid, that might because ascorbic acid could reduce MetHb directly, but reduced glutathione induce MetHb formation in purified Hb. In this study, pH of ascorbic acid solution should be adjusted to 6.00 ∼ 6.50 in advance, for ascorbic acid solution would greatly change the PH of solution and cause adverse influence to PHb.
Influence of Stabilizer
Hb was a sensitive protein; any adverse situation might result in biological inactivation. Therefore, several stabilizers (glucose, sucrose, HES20, HES40, sodium caprylate) were introduced (3%,W/V) and the influence of each agent was investigated. The results are shown in . It indicated that these agents provided nearly the same protective effect except for sodium caprylate. This phenomenon was proved by the protective mechanism that increasing viscosity of solution and making PHb more stable when heating. Therefore, one of them could be chosen. Regarding the cost and availability, glucose was chosen in our method.
Influence of pH
pH was another important factor we investigated. Many researchers preferred to have pH at 7 ∼ 8, for that slightly alkaline condition helped prevent MetHb formation. In this study, pH ranged from 5.00 ∼ 7.00 were investigated and the results are shown in . It was observed that when pH was below 6.00, more PHb denatured and the recovery was much lower than that of pH ≥ 6.00, especially when pH at 5.00, nearly all PHb denatured. High yields of Hb recovery were obtained from the conditions of pH 6 ∼ 7 with similar levels of MetHb formation. According to the literature, pI values of many non-Hb proteins present in the hemolysate were close to 6.00, and pH range from 6.00 ∼ 6.50 would promote their precipitation from Hb. Therefore, pH at 6.00 ∼ 6.50 was the best choice in our method.
The Characteristics of Purified PHb
PHb Concentration and MetHb Content. PHb concentration was usually adjusted to 10 ∼ 15 g/dl, which was suitable for further applications, and the average MetHb content was less than 2% (n = 5). In the first two hours, MetHb content increased steadily, but during the remaining heating time, MetHb tended to decrease (see ). The reason might be that heat induced residual oxy-Hb convert to MetHb at first, but later, MetHb was promoted to precipitate for its heat-unstableness. Also, heat forced O2 to release that reduced the amount of oxy-Hb, so MetHb content decreased at last.
Purity. The purity of purified PHb was determined by PAGE (), SDS-PAGE () and HPLC (). The results were illustrated in . As shown in and , the samples before purification came forth many impurity bands, while the purified PHb solution was appear to a single band indicating that nearly all impurities such as stroma and plasma protein had been removed. In , the HPLC profiles of hemolysate and purified PHb were similar in sharps and locations, and the % of peak areas were about 93% and 99%, respectively. This suggested that the purity of PHb greatly increased after purification. As shown in , the purified PHb exhibit purity above 99%.
Figure 7 SDS-PAGE pattern of purified PHb and hemolysate Band 2, 4, purified PHb Band 1, 3, hemolysate.
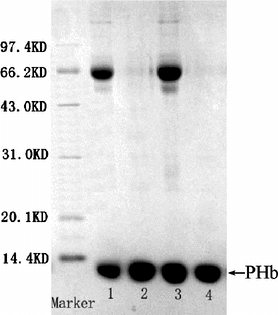
Figure 8 Native-PAGE pattern of purified PHb and hemolysate Band 1, 3, 5, 7, purified PHb. Band 2, 4, 6, 8, hemolysate.
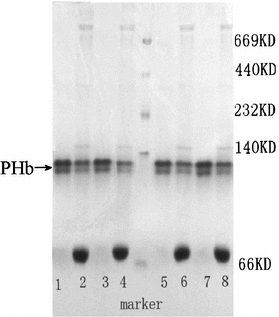
Table 1 Purity of purified PHb
Toxic Materials. The analysis of toxic materials was composed of cell debris detection, type A&B antigens detection, residual phospholipids detection and endotoxin determination. Cell membrane debris was not observed in purified PHb solution, which indicates that heat treatment, microporously filtration and ultrafiltration all were efficient technologies for removal of membrane debris. The result of type A&B antigens test was negative, thus we could infer the amount of type A&B antigens was greatly decreased, most of which were removed along with membrane debris. It is critical to remove nearly all phospholipids, largely due to fact that the phospholipids contaminant in parenteral Hb solution displayed various toxic effects even when presented at very low levels. The detection results showed that residual phospholipids content of purified PHb was less than 1 ppm. Endotoxin determination noted that endotoxin levels in purified PHb solution were less than 0.25 EU/ml.
Biological Activity Assays. The maximum absorption of samples (hemolysate and purified PHb) was at 415 nm, which was slightly different from the reference standard (), the reason might be that the standard Hb (Sigma) was adult Hb and in forms of MetHb while the placenta blood is high rich in embryonic Hb form. Also the absorption spectrums of PHb were virtually unchanged before and after heat treatment. So we could infer that purification procedure did not have adverse influence to the structure of PHb that was the premise of biological activity. Additionally, the purified PHb showed absorptions in 540 nm and 577 nm, which were the symbols of carrying O2 [Citation[23]], therefore the O2 carrying capacity of purified PHb might be reserved.
P50 was measured to further determine the biological activity of purified PHb and the result was 21 mmHg (n = 5). The slightly lower P50 value compared to that of fresh hemolysate suggested that the purified Hb by our method has an increased O2 affinity. The reason might be that heat destroyed the bonds between a-a or β-β unit of Hb and nearly all Hb dissociated into aβ subunits [Citation[20]], so O2 carrying sites of PHb exposed and O2 carrying capacity increased. Therefore, P50 of purified PHb was somewhat lower than that of hemolysate. If tetramer structure was needed, cross-linking of a-a or β-β with appropriate chemicals, such as DBBF, was necessary.
Animal experiment. In order to investigate the effect of poly-PHb on rats, we adopted the model with about 70% removal of total blood volume, and compared the effect of poly-PHb with poly-AHb on PO2 of arterial blood after blood exchange and survival; the results were summarized in the . In the group that received poly-PHb, the HCT decreased from 45.5 ± 3.2% to 11.7 ± 3.3%, so the rate of blood exchange was about 75%, and after exchange, the PO2 of rat arterial blood in this group increased dramatically, from 93.1 ± 13.9 mmHg to 131.4 ± 14.3 mmHg, all rats survived for 24 hours. For the group that received poly-AHb, the average rate of blood exchange was about 70%, and the PO2 increased from 87.3 ± 10.0 mmHg to 110.7 ± 32.1 mmHg, which was slightly lower than that of rat received poly-PHb. One reason for this phenomenon was that the O2 affinity of PHb was higher than adult Hb [Citation[21]], therefore the poly-PHb could carry more O2 in the blood circulation and bring about higher PO2 of arterial blood, which would be benefits for acute hemorrhagic shock, ischemia cerebral and heart diseases in the clinical setting. Based on our results, poly-PHb have similar 24 hours survival rate (100%) with poly-AHb, and showed better O2 carrying than that of poly-AHb. Thus, the poly-PHb could be supplied as another candidate for O2 carrying drugs in emergency.
Table 2 Efficacy of exchange blood with Poly-PHb and Poly-AHb
CONCLUSION
In this study, heat treatment was demonstrated to be a credible method to purify PHb and the process condition could be effective to inactive contaminating virus in the blood. Excessive amount of reducing agent was efficient to maintain PHb in deoxy form and resulted in higher recovery than that which introduced none or little amount of reducing agent. For its low total investment and running cost, this method was suitable for pilot-scale purification and viral inactivation of PHb.
There were no significant differences of efficacy of poly-PHb and poly-AHb in animal experiments. Therefore, PHb obtained from this method could be supplied as materials for O2 carrying drugs.
REFERENCES
- Chang, T.M.S. (2003). Artificial cells for replacement of metabolic organ functions. Artif Cells Blood Substit Immobil. Biotechnol. 31(2): 151–161, [PUBMED], [INFOTRIEVE], [CSA]
- Chang, T.M.S. (2002). Red blood cell substitutes. Artif Cells Blood Substit Immobil Biotechnol. 30(5&6): 421–437, [PUBMED], [INFOTRIEVE], [CROSSREF], [CSA]
- Yang, C.M., Li, J.Z., Ji, Y. (2001). Scientific Basis of Transfusion Medicine. University of Science and Technology of China Press: Beijing, pp. 547–568.
- Schreiber, G.B., Busch, M.P., Kleinman, S.H., Korelitz, J.J. (1996). The risk of transfusion-transmitted viral infections. New Engl. J. Med. 334(26): 1685–1690, [PUBMED], [INFOTRIEVE], [CROSSREF], [CSA]
- Nelson, Deanna J. (2002). Blood substitutes: hemoglobin-based oxygen carriers. Encyclopedia of Pharmaceutical Technology 3: 236–262, [CSA]
- Gilmore, Thomas M. (2003). “Pasteurization systems,” in Encyclopedia of Agricultural, Food, and Biological Engineering, Dennis R. Heldman, Ed., Marcel Dekker Inc., pp. 734–739.
- Azari, M., Ebeling, A., Baker, R., Burhop, K., Camacho, T., Estep, T., Guzder, S., Marshall, T., Rohn, K., Sarajari, R. (1998). Validation of the heat treatment step used in the production of diaspirin crosslinked hemoglobin (DCLHbTM) for viral inactivation. Artif Cells Blood Substit Immobil. Biotechnol. 25: 577–582, [CSA]
- Azari, M., Catarello, J., Burhop, K., Camacho, T., Ebeling, A., Estep, T., Guzder, S., Krause, K., Marshall, T., Rohn, K., Sarajari, R. (1996). Validation of the heat treatment step used in the production of diaspirin crosslinked hemoglobin (DCLHbTM) for viral inactivation-effect of crosslinking. Artif Cells Blood Substit Immobil. Biotechnol. 24(4): 303, [CSA]
- Hens, Harry J.H., Grinwis, Marc, Fokke, G., Terpstra, Bakker, Joa C. (1996). Validation of virus inactivation by pasteurization of hemoglobin solutions. Artif Cells Blood Substit Immobil. Biotechnol. 24(4): 349, [CSA]
- Estep, T.N., Bechtel, M.K., Miller, T.J., Bagdasarian, A. (1988). Virus inactivation in hemoglobin solutions by heat. Blood Substit. 16(1–3): 129–134, [CSA]
- Sakai, H., Takeoka, S., Nishide, H., Tsuchida, E. (1994). Convenient method to purify hemoglobin. Artif Cells Blood Substit Immobil Biotechnol. 22(3): 651–656, [PUBMED], [INFOTRIEVE], [CSA]
- Chang, T.M.S. (1964). Semi-permeable microcapsules. Science 146: 524–525, [PUBMED], [INFOTRIEVE], [CSA]
- Chang, T.M.S. (1997). Blood Substitutes: Principle, Methods, Products and Clinical Trials, Karger Publisher: Basel, pp. 1–141.
- Chang, T.M.S. (2000). Red blood cell substitutes. Best Pract. Res. Clin. Haematol. 13: 651–668, [CROSSREF], [CSA]
- Winslow, R.M. (2000). Blood substitutes. Advanced Drug Delivery Reviews 40: 131–142, [PUBMED], [INFOTRIEVE], [CROSSREF], [CSA]
- Sharma, A., Martin, M.J., Okabe, J.F., Truglio, R.A., Dhanjal, N.K., Logan, J.S., Khumar, R. (1994). An isologous porcine promoter permits high level expression of human hemoglobin in transgenic swine. Bio-Technology 12: 55–59, [PUBMED], [INFOTRIEVE], [CSA]
- Benesch, R., Benesch, R.E., Yung, S., Edalji, R. (1975). Hemoglobin covalently bridged across the polyphosphate binding site. Phys. Res. Commum. 63: 1123, [CSA]
- Drabkin, D. (1949). The standardization of hemoglobin measurement. Am. J. Med. Sci. 217: 710–711, [CSA]
- Evelyn, K.A., Malloy, H.T. (1938). Microdetermination of oxyhemoglobin, methemoglobin, and sulfhemoglobin in a single sample of blood. J. Biol. Chem. 126: 655–662, [CSA]
- Agnillo, F., Alayash, A.I. (2000). Site-specific modification and toxicity of blood substitutes, the case of diaspirin cross-linked hemoglobin. Advanced Drug Delivery Reviews 40: 199–212, [PUBMED], [INFOTRIEVE], [CROSSREF], [CSA]
- Bunn, H.F., Forget, B.G. (1986). Hemoglobin: Molecular, Genetic and Clinical Aspects, W.B. Saunders Co.: Philadelphia.
- Strong, F.M., Koch, G.H. (1974). Biochemisty Laboratory Manual, WM.C. Borwn Co. Publishers: Lowa, USA, pp. 150–156.
- Wang, H.Y., Zheng, W.J., Bu, F.R., Zhu, X.X., Ma, L.R. (2001). Researches on the manufacture of blood substitute's stroma polymerized bovine hemoglobin. Chin. Pharm. J. 36(6): 416–419, [CSA]