Abstract
A new enzyme electrode for the determination of creatine was developed by immobilizing creatinase (CI) and sarcosine oxidase (SO). The enzymes were co-immobilized in a poly(vinylferrocenium) matrix onto the surface of a platinum working electrode. Crosslinking with glutaraldehyte (GA) and bovine serum albumin (BSA) was selected as the best immobilization method for the enzymatic system. Determination of creatine was performed by the oxidation of enzymatically generated H2O2 at + 0.7 V vs. Ag/AgCl. The linear working range of the electrode was 2.0 × 10−5 − 3.2 × 10−4 M and the response time was about 50 s. The effects of pH, temperature, enzyme ratio and buffer concentration were investigated and optimum parameters were found to be 7.5, 37°C, 2.5:1 (CI:SO) and 0.05 M, respectively. The stability and reproducibility of the enzyme electrode have been also studied.
INTRODUCTION
Creatine is an amino acid that is synthesized from L-arginine, glycine and S-adenosylmethionine. Creatine is converted to phosphocreatine in muscle tissue in a reversible reaction with adenosine triphosphate (ATP), and this reaction is catalyzed by the enzyme creatine kinase. Phosphocreatine is a major energy storage form in the body and plays an important role in muscle energy metabolism. Creatine is excreted from the body as creatinine, thus the amount of creatinine in the urine is proportional to the amount of creatine and is used to predict the total muscle mass [Citation[1]]. Creatine is also popular as ergonoic aid [Citation[2]]. The creatine level in blood serum and urine is clinically used as a parameter of muscle damage. The physiological normal concentration range for creatine is below 140 µmol/L in serum, but pathological values due to muscle disorder may rise to concentrations higher than 1000 µmol/L [Citation[3]].
For creatine determination, the most commonly used method is the spectrophotometric one based on the Jaffé reaction, in which the active methylene group reacts with alkaline sodium picrate to give a yellow-red complex [Citation[4]]. However, this reaction is not specific for creatine, because many substances could interfere in this assay [Citation[5]]. Therefore, other various instrumental methods such as HPLC [Citation[6], Citation[7]] capillary electrophoresis [Citation[8]], HPLC-mass spectrophotometry [Citation[9], Citation[10]], and IR spectrophotometry [Citation[11]] were also proposed for the determination of creatine.
An alternative method for creatine determination is the use of enzyme electrodes, which allow direct measurement of the creatine in the samples. Several potentiometric and amperometric enzyme electrodes for creatine and creatinine have been proposed [Citation[12-15]]. Amperometric creatine and creatinine enzyme electrodes are based on the enzyme sequence described by Tsuchida and Yoda [Citation[16]] where creatininase (CA), creatinase (CI) and sarcosine oxidase (SO) are coupled to convert creatinine to amperometically detectable products, H2O2 and O2.
In creatine enzyme electrodes, creatine is converted to electroactive H2O2 by two enzymatic reactions with creatinase and sarcosine oxidase:
The idea for the construction of an amperometric enzyme electrode stemmed from the reaction of sarcosine to give H2O2 as given above with the presence of sarcosine oxidase. It is a well-known fact that H2O2 is oxidized as follows on Pt the electrode [Citation[17]]:
If a sufficiently anodic potential to create the above reaction is applied to the electrode, the anodic current passing from the circuit is proportional to the amount of H2O2 formed and thus to the concentration of creatine [Citation[18]].
In this work, we developed a new creatine enzyme electrode by the co-immobilization of two enzymes in a poly(vinylferrocenium) matrix as a redox polymer including a mediator, and we investigated the parameters that influence the electrode performance. Although the working principles of the enzyme electrode constructed are based upon the same reactions, there is no study in the literature where PVF was used as a matrix for the creatine enzyme electrode.
MATERIALS AND METHODS
Equipment and Reagents
The electrochemical studies were carried out using BAS 100 B/W electrochemical analyzer using a three-electrode cell. The working electrode was a Pt plate (0.5 cm2). The counter and the reference electrodes were a Pt wire (MW 1034) and Ag/AgCl (MF 2052) electrode, respectively. The pH values of the buffer solutions were measured with ORION Model 720A pH/ionmeter. Temperature control was achieved with Grant LTD GG thermostat.
Creatinase (E.C.3.5.3.3.; from Flavobacterium sp.) and sarcosin oxidase (E.C.1.5.3.1.; from Arthrobacter sp.) were purchased from Sigma. Dichloromethane, sodium monohydrogenphosphate and sodium dihydrogenphosphate were supplied from Riedel-de Haën. Hydrogen peroxide and sodium hydroxide were obtained from Merck. Vinylferrocen was from Aldrich. All other chemicals were obtained from Fluka. The standard solutions of creatine were prepared every 2–3 days and stored at 4°C.
Preparation of Creatine Enzyme Electrodes
The surface of the Pt electrode was cleaned according to Gros et al. [Citation[19]]. PVF was prepared by the chemical polymerization of vinylferrocene [Citation[20]]. PVF is an electroactive redox polymer and the oxidized form of the polymer PVF+ClO4− can be electroprecipatated on Pt electrode [Citation[21]]. Before the electroprecipitation, the solution was purged with nitrogen for 10 minutes in order to remove the oxygen. PVF+ClO4− modified electrode was prepared by electro-oxidizing the polymer (2mg/mL PVF) at+0.8V vs. Ag/AgNO3 in a 5 mL solution of 0.1 M tetrabutylammonium perchlorate (TBAP) in dichloromethane. The electrode was washed with phosphate buffer solution (0.05 M pH 7.5) after the coating process.
The enzymes were immobilized using the following procedures:
Adsorption: 50 µL sarcosine oxidase enzyme (26 U/mL), 150 µL creatinase enzyme (41.6 U/mL) were mixed with 50 µL 0.05 M phosphate buffer at a pH of 7.5. The resulting mixture was dropped onto the PVF+ClO4−modified Pt electrode surface. The electrode was dried at room temperature.
Crosslinking by immersing into GA: 50µL sarcosine oxidase enzyme (26U/mL), 150µL creatinase enzyme (41.6U/mL) were mixed with 50µL phosphate buffer. The resulting mixture was dropped onto the PVF+ClO4− modified Pt electrode surface. The electrode was dried at room temperature. This electrode was then dipped into a 2.5% glutaraldehyte solution in water for 10s for crosslinking.
Crosslinking with GA-BSA system: The mixture of 100µL sarcosine oxidase enzyme (26U/mL), 300µL creatinase enzyme (41.6U/mL), 1mg bovin serum albumin, 100µL 0.05M phosphate buffer at a pH of 7.5 and 30µL 5% glutaraldehyde was dropped onto PVF+ClO4− modified electrode. The electrode was dried at room temperature.
All these electrodes were washed with buffer solution several times in order to remove the non-immobilized excess enzyme and glutaraldehyde and stored in refrigerator at+4°C in phosphate buffer when not in use.
Amperometric Measurements
All amperometric measurements were performed in phosphate buffer solutions (0.05M pH 7.5) with constant stirring. We first investigated the electrochemical oxidation of H2O2 at Pt, PVF+ClO4− modified and creatine enzyme electrodes. 5mL phosphate buffer solution was added to the cell. After application of a+0.7V potential, the background current was allowed to decay to constant value. Then an aliquot of H2O2 stock solution was added to the cell and stirred for 3 minutes. The response of the electrode against H2O2 was measured after 50 s. The cell was purged with nitrogen for one minute prior to each addition of H2O2. The current values against H2O2 concentration were plotted in order to determine whether the electrode was sensitive to H2O2 or not. The same experiment was performed for Pt and creatine enzyme electrode.
In order to determine whether the enzyme electrode was sensitive to creatine, 5 mL buffer solution was added to the cell. The solution was purged with oxygen for 10 minutes and after stabilization of the background current at + 0.7 V, the aliquots of creatine stock solution were added to the cell. The response of the enzyme electrode against creatine was measured at + 0.7 V vs. Ag/AgCl after 5 minutes constant stirring and calibration curve was plotted. The cell was purged with oxygen for one minute prior to each addition of creatine to the cell.
RESULTS AND DISCUSSION
In this study, we prepared a new creatine enzyme electrode by the use of redox polymer poly(vinylferrocen). The parameters affecting the performance of the enzyme electrode were investigated.
It has been shown that PVF+ClO4− matrix catalyzes the electrooxidation of H2O2 in aqueous solution and anion exchange properties of this matrix can be used in the immobilization of enzymes [Citation[22]]. The enzymes CI and SO are in the anionic form at pH values above their isoelectric points.
In the phosphate buffer solution (pH 7.5; 0.05 M) these enzymes are negatively charged so they also are held in the polymer matrix.
H2O2 Responses of Pt, PVF+ClO4− Modified and Creatine Enzyme Electrodes
shows the current values against H2O2 concentration obtained with Pt, PVF+ClO4− modified and creatine enzyme electrodes. It is clear from the figure that the sensitivity of the PVF+ClO4− modified electrode is higher than that of Pt electrode.
Figure 1 Current difference-concentration curves obtained for the electrooxidation of H2O2 at Pt, PVF+ClO4− modified and enzyme electrodes: ▪: Pt, ▴: PVF+ClO4− modified, ♦: creatine enzyme electrodes (0.05M pH 7.5 phosphate buffer, 25°C).
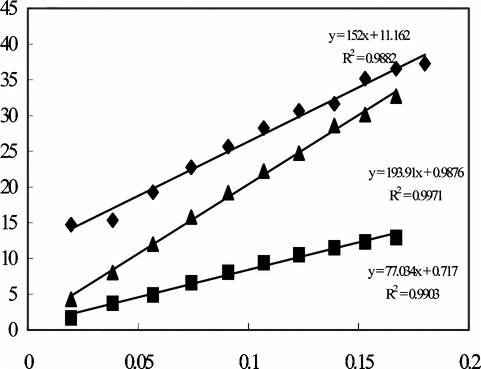
H2O2 is electrooxidized at + 0.7 V vs. Ag/AgCl on Pt electrode and PVF+ matrix catalyzes the electrooxidation of H2O2. PVF is also electrooxidized at + 0.7 V potential and the oxidized polymer, PVF+, is re-formed [Citation[23]]. This catalytic process provides an increase in the sensitivity of the PVF+ClO4− modified electrodes:
Furthermore, shows that the creatine enzyme electrode is sensitive to H2O2 with higher sensitivity than Pt electrode.
Creatine Response of the Enzyme Electrode
In order to determine the linear working ranges and sensitivities of the creatine enzyme electrodes prepared by three different immobilization methods, the current values obtained for solutions containing creatine were recorded (). As can be seen from the figure, the sensitivity of the enzyme electrode prepared by adsorption is better than the other electrodes. The linear working range of the creatine enzyme electrode prepared by crosslinking with glutaraldehyte and bovin serum albumin was 2 × 10–5 − 3.2 × 10−4 M and larger than the other two enzyme electrodes. The current remained constant at creatine concentrations above 3.2 × 10−4 M, which showed that CI and SO enzymes were saturated with creatine. The low detection limit of the creatine enzyme electrode prepared by adsorption was found to be 2.0 × 10−5M, and the linear working range was 3.8 × 10−5–2.0 × 10−4 M.
Figure 2 Creatine response of the enzyme electrodes, İmmobilization method; ♦: Adsorption ▪: Immersing into GA ▴: Crosslinking with GA-BSA (0.05 M pH 7.5 phosphate buffer, 25°C).
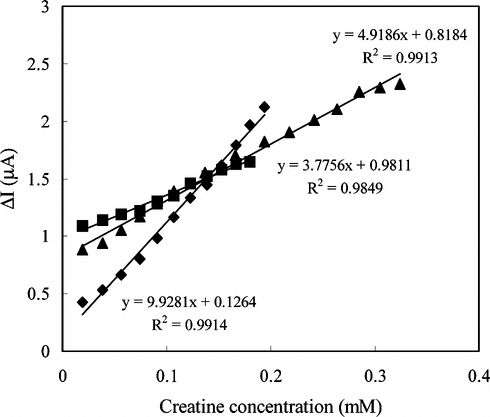
In order to test the reproducibility of the enzyme electrode prepared by adsorption, three calibration curves were plotted by the use of the same electrode (). It is clear from the figure that the reproducibility of the enzyme electrode prepared by adsorption method is not satisfactory. This can be caused by desorption of the enzymes that are poorly held in the polymeric matrix.
Figure 3 The reproducibility of the enzyme electrode (Immobilization method: adsorption; 0.05 M pH 7.5 phosphate buffer, 25°C).
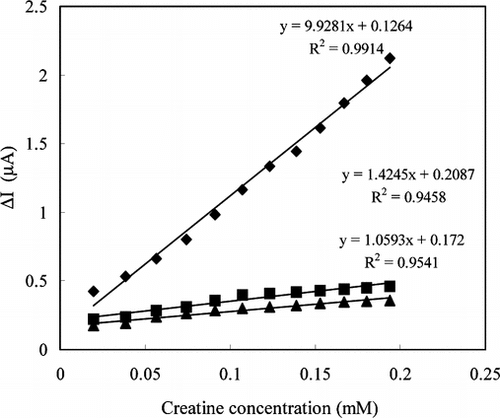
We also investigated the creatine response of the free enzyme system (data not shown). The creatine sensitivity of the free enzymes was similar to the enzyme electrode prepared by adsorption. This suggests that only a little amount of the enzymes was held in the polymeric matrix by the adsorption method; most of the immobilize enzymes were moved from the polymeric matrix to the solution immediately and behaved as free enzymes.
Because the reproducibility of the enzyme electrode prepared by adsorption was not satisfactory, we investigated different immobilization methods. The enzyme electrode prepared by adsorption was dipped into glutaraldehyte solution for the crosslinking of the enzymes. The creatine response of this electrode was given in . The reproducibility of the electrode was also tested (). The reproducibility of this electrode was better than the electrode prepared by adsorption, but it had lower sensitivity than that observed with one of the enzyme electrodes immobilized by the adsorption method. This result suggests that the enzymes lose activity when crosslinked with glutaraldehyte.
Figure 4 The reproducibility of the enzyme electrode prepared by immersing into GA (0.05 M pH 7.5 phosphate buffer, 25°C).
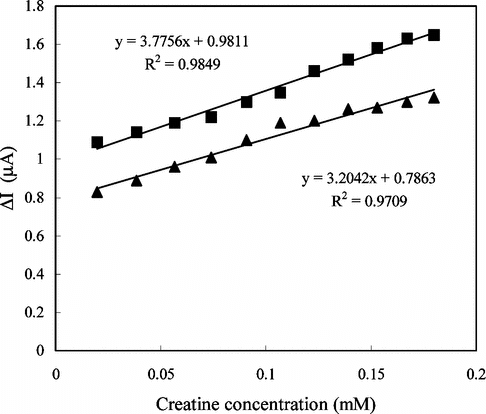
We also studied the parameters that affect the electrode response for the enzyme electrodes prepared by adsorption and immersing into GA (data not shown) and we performed all the measurements under optimum conditions.
In order to improve the sensitivity, linear working range and reproducibility of the enzyme electrodes, enzymes were crosslinked with GA and BSA system and the parameters affecting the performance of the electrode were investigated. showed that enzyme electrode prepared by this method has a larger linear working range than the others.
Creatine Enzyme Electrodes Prepared by Crosslinking with GA-BSA System
Response Time
The amperometric response time of the enzyme electrode to creatine was determined at two different creatine concentrations. The current differences for 1.2 × 10−5 and 1.8 × 10−4 M creatine against time were plotted. The results showed that the response time was shorter at lower concentrations than at higher concentrations. The response time can be taken as 2 minutes where the currents are approximately constant. Since the response curves are parallel to each other, the measurements can be taken before 2 minutes, provided that they are made exactly at the same times. The response time was expected as 50 s and all the parameters were investigated based upon the measurements taken after 50 s. This response time is quite fast and highly suitable for biosensor response [Citation[24]]. There are longer [Citation[25]] and shorter [Citation[18]] response times for creatine enzyme electrodes in literature.
The Effect of pH
The buffers at various pH values were tested to investigate the effect of pH. The pH of the buffers was varied between 5.0 and 9.0. The measurements were performed at a constant creatine concentration of 1.4 × 10−4 M. shows that the maximum response was obtained at pH 7.5. Therefore pH 7.5 was selected as the optimum pH and all following measurements were performed at this pH. This value is in good agreement with most of the data reported in the literature [Citation[16], Citation[18], Citation[26], Citation[27]]. In creatine enzyme electrodes pH values different than 7.5 were also reported in the literature [Citation[12], Citation[25], Citation[28], Citation[29]]. This was attributed to the fact that the used polymer and the immobilization methods were different. The decrease in the responses of the enzyme electrode at pH values below and above 7.5 can be attributed to the change of the enzyme conformations and thus decrease of the enzyme activities.
The Effect of Temperature
Temperature has a great effect on enzyme activity and it is important to investigate the temperature dependence of the response of the enzyme electrode. The temperature influence on the response of creatine enzyme electrode was tested between 20°C and 45°C at pH 7.5 using constant creatine concentration of 1.4 × 10−4 M (). As seen from the figure, the electrode response increases with temperature up to 37°C and decreases afterwards. The highest electrode response was obtained at 37°C. The sudden decrease in the responses after 37°C is thought to be caused by the denaturation of the enzymes. Although the electrode response is higher at 37°C, we performed the creatine measurements at room temperature (25°C–27°C) without thermal control because of the fact that the linearity of the calibration curves plotted at 37°C was not satisfactory.
The Effect of Phosphate Concentration
The amperometric response of the enzyme electrode was determined at five different phosphate concentrations of the phosphate buffer used in the study at a pH value of 7.5 with a constant creatine concentration of 1.4 × 10−4 M. The response of the enzyme electrode was plotted against the phosphate concentration (). The figure shows that the best response was obtained in a buffer solution with phosphate concentration of 0.05 M. This result was found to be in good compliance with similar studies in the literature [Citation[18], Citation[30], Citation[31]]. Above or below this concentration the response was found to show a significant decrease. It can be concluded that the buffering capacity of the solution was decreased at lower phosphate concentration. At higher phosphate concentration, on the other hand, the conductivity of the PVF+ films was decreased as a result of the replacement of the negativity charged perchlorate ions in the structure of the film with phosphate ions [Citation[21]], resulting in decreased responses.
The Effect of Enzyme Ratio
The effect of the enzyme ratio used in the immobilization on the electrode response was also determined. By keeping the concentration of sarcosine oxidase constant at 4 mg/mL and varying the creatinase concentration, the response of the enzyme electrode was measured at four different ratios at a pH of 7.5 in the creatine solution of 1.4 × 10−4 M. The results are shown in . The maximum response was obtained with ratio of creatinase to sarcosine oxidase to be 3.3:1(m/m). We used the enzyme ratio of 2.5:1(m/m) in our studies because of the fact that the linearity of the calibration curves plotted with this ratio was better than the linearity obtained with an enzyme ratio of 3.3:1(m/m).
The Reproducibility of the Enzyme Electrode
The reproducibility of the enzyme electrode prepared by crosslinking with GA-BSA system was also investigated. Five calibration curves were plotted by the use of the same electrode (). The relative standard deviation of the sensitivities (the slopes of the curves) was found to be 14.5%. This result indicates that the reproducibility of the enzyme electrode prepared by crosslinking with GA-BSA system was highly satisfactory when compared with the other two enzyme electrodes prepared with adsorption and immersing into GA.
Storage Stability
shows the stability of the creatine enzyme electrode at 4°C. There was a rapid decrease in current values during the first four days. There was no significant change in the responses between 5th and 14th days, but the current values decreased sharply after 15th day. This shows that the enzyme electrode can be used for two weeks.
Interferences
Ascorbic acid and uric acid are two of the common interferences that can be found in serum and they are also oxidized at a potential of + 0.7 V applied to the platinum working electrode similar to the enzymatically formed H2O2 [Citation[32]].
The sensitivity of the enzyme electrode towards H2O2 was compared to those of ascorbic acid and uric acid in physiological concentrations and the enzyme electrode produced higher current values than creatine towards ascorbic acid and uric acid. The results showed that both compounds provide a potential source of interference and that might cause a positive error in creatine determination.
Creatine Determination in Human Serum
The proposed creatine enzyme electrodes were used to determine the creatine in hemodialysis patients in serum. For the evaluation of the new creatine enzyme electrode, the measurement results were compared to those obtained by the Jaffé method.
The creatine enzyme electrode was placed in the cell containing 5 mL phosphate buffer and after the stabilization of background current, 0.5 mL of the serum sample was added. After the current again reached a steady-state value, standard addition procedure was used to determine the creatine. The standard addition procedure was performed by subsequent addition of standard creatine solution. The results obtained by creatine enzyme electrodes were higher than those by obtained from the Jaffé method. The higher values determined with the creatine enzyme electrode can be caused by the interferences in serum.
Our future study will be focused on the modification of the electrodes by using anti-interference layers to avoid interfering from electroactive compounds such as ascorbic acid and uric acid.
CONCLUSION
In this study we investigated three different enzyme immobilization techniques on the surface of the PVF+ClO4− modified electrode. Creatinase and sarcosine oxidase enzymes were immobilized by adsorption, immersing into GA and crosslinking with the GA-BSA system. Crosslinking with the GA-BSA system was found to be the optimum immobilization procedure. This immobilization technique provides a good sensitivity, a wider linear working range and higher reproducibility for the creatine enzyme electrode.
The properties and the optimum working conditions of the enzyme electrode prepared by crosslinking with GA-BSA system are summarized in .
Table 1 The properties and the optimum working conditions of the enzyme electrode
It can be concluded that the presented redox polymer, PVF+, is a suitable immobilization matrix for creatinase and sarcosine oxidase. This matrix catalyzes the oxidation of hydrogene peroxide and provides the development of sensitive amperometric creatine enzyme electrodes.
We gratefully acknowledge the financial support of T.R. Prime Ministry State Planning Organization (Project No: 98-K-120830) and a scholarship by Scientific and Technical Research Council of Turkey for P. E. Erden.
REFERENCES
- Champe, P., Harvey, R. (1997). Biyokimya, Nobel Tιp Kitap Evleri: İstanbul, pp. 264–265.
- Stefan, R.-I., Bokretsion, R.G., Van Standen, J.F., Aboul-Enein, H.Y. (2003). Simultaneous determination of creatine and creatinine using amperometric biosensors. Talanta. 60: 1223–1228, [CSA]
- Sena, S.F., Syed, D., McComb, R.B. (1988). Effect of high creatine content on the Kodak single-slide method for creatinine. Clin. Chem. 34: 594–595, [PUBMED], [INFOTRIEVE], [CSA]
- Jaffé, M.Z. (1886). Über den Niederschlang welchen Pikrinesäure in normalen Harn erzeugt und über eine neue Reaktion des Kreatinins. Hoppe-Seyler's Z. Physiol Chem. 10: 391–400, [CSA]
- Weber, J.A., Van Zanten, A.P. (1991). Interferences in current methods for measurement of creatinine. Clin. Chem. 37: 695–700, [PUBMED], [INFOTRIEVE], [CSA]
- Persky, A.M., Hochhaus, G., Brazeau, G.A. (2003). Validation of a simple liquid chromatography assay for creatine suitable for pharmacokinetic applications, determination of plasma protein binding and verification of percent labeled claim of various creatine products. J. Chromatography B 794: 157–165, [CROSSREF], [CSA]
- Karatzaferi, C., De Haan, A., Sargeant, A.J. (1999). Improved high-performance liquid chromatographic assay for the determination of high-energy phosphates in mammalian skeletal muscle. Application to a single-fibre study in man. J. Chromatography B 730: 183, [CSA]
- Smith-Palmer, T. (2002). Seperation methods applicable to urinary creatine and creatinine. J. Chromatography B. Review 781: 93–106, [CROSSREF], [CSA]
- Yasuda, M., Sugahara, K., Zhang, J., Ageta, T., Nakayama, K., Schuin, T. (1997). Simultaneous determination of creatinine, creatine and guanidoaceticacid in human serum and urine using liquid chromatography-atmospheric pressure chemical ionization mass spectrometry. Anal. Biochem. 253: 231–235, [PUBMED], [INFOTRIEVE], [CROSSREF], [CSA]
- Schwedhelm, I., Tsikas, D., Durand, T., Gutzki, F.M., Guy, A., Rossi, J.C., Froelich, J.C. (2000). Tandem mass spectrometric quantification of 8-iso-prostoglandin F2alpha and its metabolite 2,3-dinor-5,6-dihydro-8-iso-prostoglandin. J. Chromatography B: Biomed. Sci. Appl. 744: 99–112, [CROSSREF], [CSA]
- Pezzaniti, J.L., Jeng, T.W., Mcdowell, L., Oosta, G.M. (2001). Preliminary investigation of near-infrared spectroscopic measurements of urea, creatinine, glucose, protein, and ketone in urine. Clin.Biomed. 34: 239–246, [CSA]
- Koncki, R., Walcerz, I., Ruckruh, F., Glab, S. (1996). Bienzymatic potentiometric electrodes for creatine and L-arginine determination. Anal. Chim. Acta. 333: 215–222, [CROSSREF], [CSA]
- Yomato, H., Ohwa, M., Wernet, W. (1995). A polypyrrole/three-enzyme electrode for creatinine detection. Anal. Chem. 67: 2776–2780, [CROSSREF], [CSA]
- Madaras, M.B., Buck, R.P. (1996). Miniaturized biosensors employing electropolymerized permselective films and their use for creatinine assays in human serum. Anal. Chem. 68(21): 3832–3839, [PUBMED], [INFOTRIEVE], [CROSSREF], [CSA]
- Shin, J.H., Choi, Y.S., Lee, H.J., Choi, S.H., Ha, J., Yoon, I.J., Nam, H., Cha, G.S. (2001). A planar amperometric creatinine biosensor employing an insoluable oxidizing agent for removing redox-active interferances. Anal. Chem. 73: 5965–5971, [PUBMED], [INFOTRIEVE], [CROSSREF], [CSA]
- Tsuchida, T., Yoda, K. (1983). Multienzyme membrane electrodes for determination of creatinine and creatine in serum. Clin. Chem. 29: 51–55, [PUBMED], [INFOTRIEVE], [CSA]
- Umana, M., Waller, J. (1986). Protein—modified electrodes. The glucose oxidase/polypyrrole system. Anal. Chem. 58: 2979–2983, [CROSSREF], [CSA]
- Schneider, J., Gründig, B., Renneberg, R., Cammann, K., Madaras, M.B., Buck, R.P., Vorlop, K.-D. (1996). Hydrogel matrix for three enzyme entrapment in creatine/creatinine amperometric biosensing. Anal. Chim. Acta. 325: 161–167, [CROSSREF], [CSA]
- Gros, P., Durliat, H., Comtat, M. (2000). Use of polypyrrole film containing Fe(CN)63− as pseudo reference electrode: Application for amperometric biosensors. Electrochim. Acta. 46: 643–650, [CROSSREF], [CSA]
- Smith, W.T., Kuder, J., Wychick, E. (1976). Voltommetric behaviour of poly(vinylferrocene). J. Polym. Sci. 14: 2433–2448, [CSA]
- Gülce, H., Çelebi, S.S., Özyörük, H., Yιldιz, A. (1995). Amperometric enzyme electrode for sucrose determination prepared from glucose oxidase and invertase co-immobilized in poly(vinilferrocenium). J. Electroanal. Chem. 397: 217–223, [CROSSREF], [CSA]
- Gülce, H., Özyörük, H., Çelebi, S.S., Yιldιz, A. (1995). Amperometric enzyme electrode for aerobic glucose monitoring prepared by glucose oxidase immobilized in poly(vinilferrocenium). J. Electroanal. Chem. 394: 63–70, [CROSSREF], [CSA]
- Gülce, H., Gülce, A., Yιldιz, A. (2002). A novel two-enzyme amperometric electrode for lactose determination. Anal. Sci. 18: 147–150, [CROSSREF], [CSA]
- Telefoncu, A. (1999). Biyosensörler, Biyokimya Lisansüstü Yaz Okulu, Haziran 20-26, Ege Üniversitesi Yayιnlarι: Kuşadasι, pp. 136–137.
- Madaras, B.M., Popescu, I.C., Ufer, S., Buck, R.P. (1996). Microfabricated amperometric creatine and creatinine biosensors. Anal. Chim. Acta. 319: 335–345, [CROSSREF], [CSA]
- Kim, E.J., Harayuma, T., Yanagida, Y., Kobatake, E., Aizawa, M. (1999). Disposable creatinine biosensor based on thick-film hydrogen peroxide electrode system. Anal. Chim. Acta. 394: 225–231, [CROSSREF], [CSA]
- Yao, T., Kotegawa, K. (2002). Simultaneous flow-injection assay of creatine and creatinine in serum by the combined use of a 16-way switching valve, some specific enzyme reactors and a highly selective hydrogen peroxide electrode. Anal. Chim. Acta. 462: 283–291, [CROSSREF], [CSA]
- Motonaka, J., Takabayashι, H., İkeda, S., Tanaka, N. (1988). The preparation and properties of an enzyme electrode for creatine. The Chemical Society of Japan 61: 3341–3343, [CSA]
- Khan, G.F., Wernet, W. (1997). A highly sensitive amperometric creatinine sensor. Anal. Chim. Acta. 351: 151–158, [CROSSREF], [CSA]
- Tombach, B., Schneider, J., Schaefer, R.M., Chemnitius, G.C. (2001). Amperometric creatinine biosensor for hemodialysis patients. Clin. Chim. Acta. 312: 121–134, [CROSSREF], [CSA]
- Nguyen, V.K., Wolff, M., Series, J.L., Schwing, J.-P. (1991). İmmobilized enzyme electrode for creatinine determination in serum. Anal. Chem. 63: 611–614, [PUBMED], [INFOTRIEVE], [CROSSREF], [CSA]
- Wu, J., Suls, J., Sansen, W. (2000). Amperometric determination of ascorbic acid on screen printing ruthenium dioxide electrode. Electrochemistry Communications 2: 90–93, [CROSSREF], [CSA]