Abstract
Surface decoration of hemoglobin (Hb) with six copies of PEG-5K employing thiolation mediated PEGylation platform neutralizes the vasoaconstricive activity of acellular Hb. The molecular size homogeneity of hexaPEGylated Hb, in spite of the fact that the PEGylation is distributed at multiple sites and PEGylation at each of the sites is not quantitative, is an unusual aspect of this PEGylation reaction. We have introduced three cys residues—Cys-13 (α), Cys-111 (α), and Cys-13 (β)—onto Hb by molecular modeling. This new mutant Hb with four reactive Cys residues has been used to build molecular models of PEGylated Hbs with two, four, six, and eight PEG-chains of different masses. The calculated loss of surface area was used to design and gain insight into the structure and the surface shielding of PEGylated Hbs. The modeling shows the adequate surface coverage of the protein hemoglobin with six copies of PEG-5K chains and also exhibits more surface coverage of the hemoglobin as compared to that afforded by two copies of PEG-20K chains.
INTRODUCTION
The development of artificial blood or blood substitutes has been pursued in different directions to fulfill the growing needs of blood in the world. The modified hemoglobin is one of the approaches pursued by different research groups. Increasing the molecular size of acellular hemoglobin (Hb) has been proposed as an approach to reduce its undesirable vasoactive properties. Poly(ethylene glycol) (PEG) is a water soluble polymer that when covalently linked to proteins, alters their properties in ways that extend their potential uses. PEG-modified conjugates are being exploited in many different fields. The improved pharmacological performance of PEG-proteins when compared with their unmodified counterparts prompted the development of this type of conjugate as a therapeutic agent [Citation[1-3]]. The decoration of hemoglobin with PEGylation to increase the molecular size in order to use it as blood substitute is our goal. The PEG-protein conjugates exhibit: 1) enhanced solubility, 2) decreased antigenicity, 3) decreased proteolysis, 4) reduced rate of kidney clearance, 5) non toxic. This decoration not only fulfills the oxygen carrying capacity of hemoglobin and also satisfies the colligative requirements: colloid osmotic pressure and viscosity. The objective of most PEG conjugation techniques is to increase the circulation half-life without altering activity. PEG molecular weight has a direct impact on the activity; higher molecular weight PEG conjugates tend to have lower in vitro activity but have higher in vivo activity due to the improved pharmacokinetic profile [Citation[4]]. Polyethylene glycols have been used extensively for the precipitation and crystallization of proteins. They also lower the surface tension of water and display strong nonpolar character. Our earlier [Citation[5]] studies suggest that the modulation of the vasoactivity of Hb by PEG could be a function of surface shielding.
It was also shown that polyethylene glycol is not a fully flexible random coil in aqueous solution, but there are some restrictions in the rotations about the bonds along the chain [Citation[6]]. The surface decoration involves three main factors: the mass of the PEGylated molecule, linkage positions on the molecular surface, and type of linkage between PEG molecule and hemoglobin.
Molecular modeling provides the amount of shielding, effect of mass and the number of molecules. We have considered eight sites of linkage in the hemoglobin molecule, three types of PEG chains in terms of mass (5k, 10k, 20k) and with maleidophenyl linkage. Static surface shielding has been calculated by accessibility studies.
MATERIALS & METHODS
The hemoglobin molecule was taken from the protein Data bank (4HHB). These coordinates were refined by steepest descent method to remove any van der Waals overlap and steric clash. The original structure was intact from the studies. The chosen sites were mutated to Cys residues, using biopolymer module in Insight II. The new cys residue position was verified from the rotatmer library in WHATIF [Citation[7]]. The maleiophenyl PEG was built using the builder module in INSIGHTII. The chains were built linearly. The final models were derived by simulated annealing, by carrying out MD simulations from 300 K to 600 K and back to 300 K. The covalent bonding between the PEG molecule and CYS residue was carried out by inking its SH group with PEG molecule by a succinimidophenyl linkage [Citation[8]]. The whole complex was energy minimized by steepest energy minimization (10,000 steps). A 10 Å cutoff was used for truncating the van der Waals and electrostatic interactions. The Consistent Valence Force Field (CVFF) was used for our calculations. The discover program In INSIGHT module was used for our calculations. The increase in the volume and accessible area was also calculated from the static structures. These calculations were carried out using the algorithm of Lee & Richards [Citation[9]], and using the program developed at Scripps by Sanders & Olson [Citation[10]]. The Insight Graphics was mainly used for computer modeling, followed by Rasmol.
RESULTS & DISCUSSION
The crystal structure of Hemoglobin (Protein Data Bank entry 4HHB [Citation[11], Citation[12]]) was the starting model for this study. The coordinates were obtained from Protein Data Bank. The structure was minimized using the CVFF force field and using partial charges for all the atoms. The partial double amine bond was used to connect the PEG chains to the linker molecule.
After minimization the overall change in the shape is only 0.2 Å, showing the conservation of the overall structure. All hydrogen atoms were generated for the whole molecule. The hemoglobin contains four chains (two identical α and β chains).
PEGylation of free cysteine residues in proteins is the main approach for site-specific modification because reagents that specifically react with cysteins are available and the number of free cysteins on the surface of the proteins is much less than lysine residues. In the absence of free cysteine in a native protein one or more free cysteines can be added by genetic engineering. The advantage of this approach is that it makes possible site-specific PEGylation at areas on the proteins that will minimize a loss in biological activity but decrease immunogenicity. The wild type contains two cys residues (β 93). We have mutated the following residues to cys for further linkage: α 111, α 13, and β 13. These sites for placing PEG-chains have been selected based on the reactivity of these Cys residues in animal Hbs [Citation[13]]. shows the four chains of hemoglobin and the attachment sites. Our goal is to shield the hemoglobin molecule by PEGylation. shows the distances between the various sites. This figure shows that in terms of geometry, adequate coverage points were chosen. The shortest distance is 18 A between the residues and 53 A is the longest distance. If we consider the hemoglobin of a sphere of diameter of ∼ 60 A, the starting points are adequate. The chemical formula for the PEG chain is shown in . The linkage with cys molecules is shown in . The double bond in the ring structure was broken to form a linkage with cys residues; the two ring structures followed by a partial double bond keeps the PEG cluster away from the protein chain. We have not treated the PEG cluster as a fully random coil. The simulated annealing of the PEG molecules of 5 K and 10 K ended up with a structure with overall elliptical shape. The starting models of 5 K, 10 K & 20 K after buildup and simulated annealing was shown in . Even after many attempts of simulated annealing procedure, much globularity is not obtained with 20k PEG molecule. We have also started with different starting conformations to overcome any local minima; we arrived at similar cylindrical conformation from our simulation. Even after 1 ns simulation with hemoglobin, the structure remains more or less intact. This convinces the reliability of our starting PEG model. We then covalently link 5 K PEG molecules at β 93, followed by α 111, α 13, β 13. At each stage, the steric overlap is avoided and high surface coverage is attempted. At each stage the surface coverage is shown in . The accessible area after each addition is calculated and shown in . The surface coverage steadily increases (surface area decrease) with the addition of PEG chains up to 6 molecules. Then the increase is minimal. After 8 molecules, we have to perturb the hemoglobin molecules to introduce new PEG molecules. After 6 5k PEG molecules, the surface coverage has not increased in proportion to the mass added. This modeling clearly shows the adequacy of 6 PEG chains to decorate the hemoglobin and the increase in the number of chains does not provide enough surface coverage on par with the added mass. We also attempted to cover the hemoglobin with 4 10k PEG molecules (Fig. 10a) and two 20k PEG molecules (Fig. 10b). But the loss of area was not in comparison with 5k surface coverage. The loss of area for 4 10k molecules is 4453 Å2, whereas for 2 20k molecules the loss is only 2810 Å2. Therefore the surface coverage is not on par with mass added. The addition increases the total surface exposure as well as increasing volume. shows the total exposure area and volume for the various complexes. The number of water molecules displaced due to PEGylation is also given. The table shows increase in the size of the molecule due to PEGylation and the number of water molecules displaced due to PEGylation. The average increase in volume and number of displaced water molecules per 5 K appears to be uniform; the total area of exposure is different due to linkage and shape of the molecule. The surface area is a relative measure of first hydration shell. For the same mass addition, 8 5k shows the highest increase in volume, but lowest increase in surface area in comparison with 4 10k PEGylation and 2 20k PEGylation. Both size of the molecule, as well as surface coverage, plays an important role in the vascoocular properties of these oxygen carriers. Modeling studies play an important role in the design of PEGylation in terms of size, attachment site and nature of attachment. These reflect changes in molecular specific volume (the reciprocal of the density).
Figure 1 Four chains of hemoglobin are shown in the form of ribbon. Light gray chains correspond to α type and dark gray chains correspond to β. All the eight sites are shown with cys residues in CPK model. The cys atoms color coded with white correspond to the sulfur atoms.
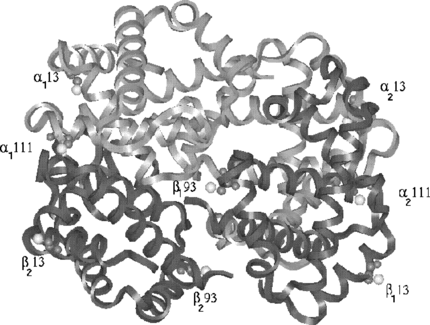
Figure 2 The distances between the sites are shown to show the adequate coverage of the protein surface. The shortest distance is 13 Å and widest distance is 56.8 Å.
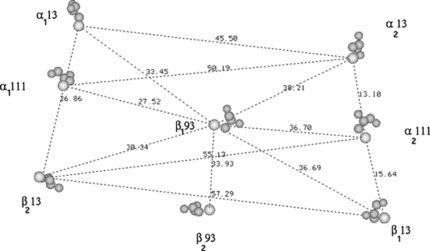
Figure 3 a) Structure of the poly(ethylene glycol)-based mono functional maleimide reagents. The number n gives the number of PEG molecules in the various chains in our study; b) The maleidophenyl linkage between cys residue and the PEG chains.
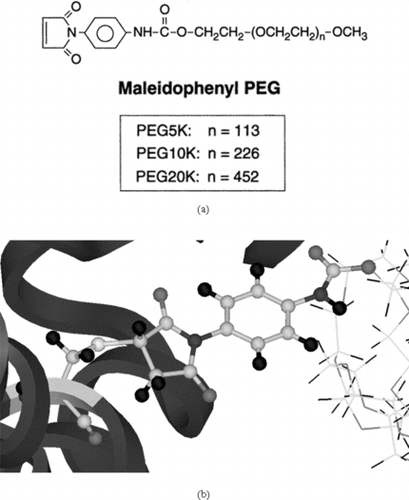
Figure 4 The three-dimensional models of the 5K, 10K and 20K PEG chains involved in our analysis. The connecting maleimide segment between the PEG and protein is shown in CPK type.
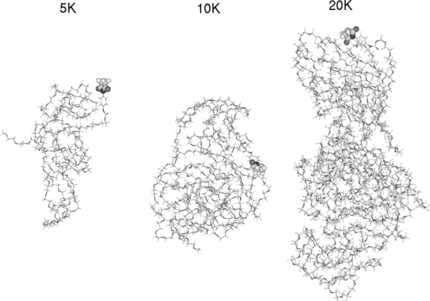
Figure 5 a)Symmetric linkage of 2 5k PEG chains with Hemoglobin at β93; b) Symmetric linkage of 4 5k PEG chains with Hemoglobin at β93 & α111; c) Symmetric linkage of 6 5k PEG chains with Hemoglobin at β93, α111 & α13; d) Symmetric linkage of 8 5k PEG chains with Hemoglobin at β93, α111 α13 & β13 (hydrogen are omitted in c & d for clarity).
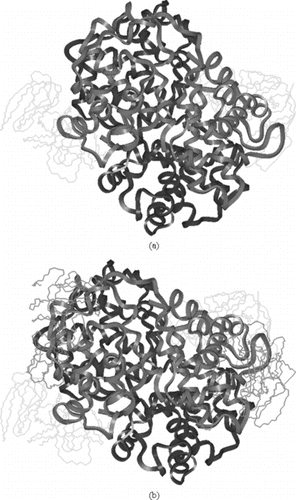
Figure 6 The accessible surface area for the water molecule of 1.4 Å radius at various stages of PEGylation of 5 K mass (2, 4, 6, & 8) are shown. The zero corresponds to the crystal structure.
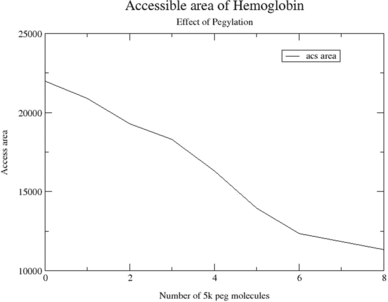
Figure 7 a) Symmetric linkage of 4 10k PEG chains with hemoglobin at β93 & α111; b) Symmetric linkage of 2 20k PEG chains with hemoglobin at β93 (hydrogen are omitted). In , and have the attachment of same mass.
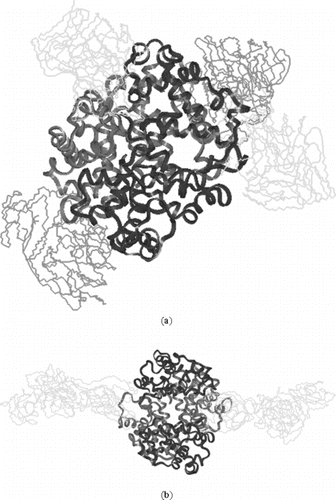
Table 1. Area & volume of modified hemoglobins
Our modeling clearly shows the usefulness of the selection of size and mass of the PEGylation. This also shows that compactness of the surface coverage plays an important role in the stability of structure and thereby function. A comparison of 6 5k PEGylation and 8 5k PEGylation shows that increase in the mass also plays an important role in increasing the perturbation of the protein as measured from root mean square deviation of the PEGylated protein model in comparison with the starting model.
The main theme for this work is the nature of surface coverage due to PEGylation. The static results clearly shows the adequacy of 6 5k molecules in comparison with 8 5k, 4 10k, 2 20k PEGylation.
CONCLUSIONS
Computer modeling is carried out to design PEGylation with hemoglobin. The linkage sites and the number of PEG molecules and their mass are evaluated in terms of surface coverage and stability of the complex. Surface decoration of Hb with six copies of PEG-5K employing Maleidophenyl mediated PEGylation shows higher and adequate coverage in comparison with 4 10k PEG chains or two 20k chains. The reduction in the surface exposure of hemoglobin with the increase of 5k chains is linear up to 6 chains and becomes relatively flat afterwards. Our modeling efforts also exhibit the increase in the surface coverage of the protein by each PEGylation, thereby restricting the surface availability of the protein for further PEGylation. Both the two or four chains of PEG-5k chains decrease the surface exposure but do not saturate the surface coverage. The six PEG-5K chains reduces nearly 50 percent of the exposure area as shown in static calculations.
REFERENCES
- Veronese, F.M. (2001). Peptide and protein PEGylation: A review of problems and solutions. Biomaterials 22: 405–417. [INFOTRIEVE], [CSA]
- Kozlowski, C.A., Hariis, J.M. (2001). Improvements in protein PEGylation: PEGylated interferons for treatment of hepatitis C. J. of Controlled Disease 72: 217–224. [CSA], [CROSSREF]
- Pedro Nacharaju, Cabrales, Manjula, Parimala, Tsai, Belur N., Acharya, Amy G., Seetharama, A., Intaglietta, Marcos. (2005). Early difference in tissue pH and microvascular hemodynamics in hemorrhagic shock resuscitation using polyethylene glycol-albumin- and hydroxyethyl starch-based plasma expanders. Shock. 24: 2466–73. [CSA], [CROSSREF]
- Bailon, P., Palleroni, A., Schaffer, C.A., Spence, C.L., Fung, W.J., Porter, J.E., Erlich, G.K., Pen, W., Xu, Z.X., Modi, M.W., Farid, A., Berthold, W., Graves, M. (2001). Rational design of a potent, log lasting form of interferon: A 40 kDa branched polyethylene glycol-conjugated interferon alpha-2a for the treatment of hepatitis C. Bioconjug. Chem. 12: 195–202. [INFOTRIEVE], [CSA], [CROSSREF]
- Manjula, B.N., Tsai, A., Upadhya, R., Perumalsamy, K., Smith, P.K., Malavalli, A., Vandegriff, K., Winslow, R.M., Intaglietta, M., Prabhakaran, M., Friedman, J.M., Acharya, A.S. (2003). Site specific PEGylation of hemoglobin at cys-93(beta): Correlation between the colligative properties of the PEGylated protein and the length of the conjugated PEG chain. Bioconjucate Chemistry 14: 464–72. [CSA], [CROSSREF]
- Bhat, R., Timasheff, S.N. (1992). Steric exclusion is the principle source of the preferential hydration of proteins in the presence of polyethylene glycols. Protein Science 1: 1133–43. [INFOTRIEVE], [CSA]
- Vriend, G. (1990). WHAT IF: A molecular modeling and drug design program. J. Mol. Graph. 8: 52–56. [INFOTRIEVE], [CSA], [CROSSREF]
- Roberts, M., Bentley, J., Harris, M.D.J.M. (2002). Chemistry for peptide and protein PEGylation. Adv. Drug Del. Rev. 54: 459–76. [CSA], [CROSSREF]
- Lee, B., Richards, F.M. (1971). The interpretation of protein structures: Estimation of static accessibility. J. Molec. Biol. 55: 379–400. [INFOTRIEVE], [CSA], [CROSSREF]
- Sanner, Michel, F., Arthur J. Olson, Jean-Claude, Spehner. (1995). Fast and robust computation of molecular surfaces scanner. Proc. 11th ACM Symp. Comp. Geom. C6–C7.
- Fermi, M., Perutz, F., Shannan, B., Fourme, R. (1984). The crystal structure of human deoxyhaemoglobin at 1.74 A resolution. J. Mol. Biol. 175: 159–165. [INFOTRIEVE], [CSA], [CROSSREF]
- Forunme, R., Ascone, I., Kahn, R., Mezouar, M., Bouvier, P. (2000). Opening the high-pressure domain beyond 2 kbar to protein and virus crystallography – technical advance. Structure 10: 1409–1412. [CSA]
- Manjula, B.N., Tsai, A.G., Intaglietta, M., Tsai, C.H., Ho, C., Smith, P.K., Perumalsamy, K., Kanika, N.D., Friedman, J.M., Acharya, S.A. (2005). Conjugation of multiple copies of polyethylene glycol to hemoglobin facilitated through thiolation: Influence on hemoglobin structure and function. Protein J. 24: 133–46. [INFOTRIEVE], [CSA], [CROSSREF]