Abstract
Yeast cells were immobilized by absorption onto porous ceramic support and evaluated for continuous beer primary fermentation using a bioreactor in comparison to yeast cells immobilized by entrapment in calcium alginate gel. The effects of temperature and flow rate as a function of reaction/fermentation time on fermentation rate were investigated. The fermentation reaction (in terms of loss of total soluble solids in the beer wort as a function of time) was first-order with half-lifes in the range of ∼ 9–11 hours at ∼ 10–12°C at beer wort linear flow rates of ∼ 0.8–1.6 cm/minute for ceramic support, compared with ∼ 16 hours for Ca-alginate gel, the former support matrix being more efficient and demonstrating greater potential for future commercial application.
INTRODUCTION
Beer production is often cited as a typical example of traditional biotechnology because of its extremely long history, but it is also true that brewing has been and still is at the forefront of biotechnological development, since the modern malting and brewing industry develops and implements a wide range of new technical, biochemical, microbiological and genetic processes and inventions [Citation[1], Citation[2]]. In the competitive world of beer production, efficient utilization of raw materials and equipment together with smooth operation is advantageous [Citation[3]]. In the brewing industry, fermentation and maturation technology in most breweries takes the form of time-consuming low productivity batch processes. Continuous immobilized yeast cell technology has been examined and evaluated to intensify these traditional fermentation processes. Immobilized cell systems are heterogeneous systems in which considerable mass transfer limitations can occur, resulting in a changed yeast metabolism. Therefore, proper selection and design of the carrier material should go hand in hand with the design of the accessory reactor [Citation[4]].
There is growing interest in using immobilized cell systems for different fermentation processes [Citation[5-7]]. This is due to the advantages of immobilized cell technology over traditional batch free-cell systems, since they permit higher bioreactor cell densities, make reutilization and continuous operation possible, and result in easier purification. There are several industrial applications of such systems for the secondary fermentation of beer and for the production of alcohol-free and low-alcohol beer [Citation[4], Citation[8], Citation[9]], but immobilized cell systems have not yet been successfully applied for primary beer fermentation [Citation[10]].
In immobilization technology, the nature of supports/matrix used plays a very important role in the success and commercialization of the developed technology. The substantial concern with use of immobilized yeast cells for beer production is reduction of internal mass transfer resistance during continuous fermentation [Citation[7]]. External mass transfer, which includes the exchange of nutrients and products between the cell carrier surface and the surrounding medium, greatly depends on bioreactor design and flow pattern. Internal mass transfer relates to transfer of substrates and products within the carrier, i.e. through the polymeric carrier matrix and dense aggregates of immobilized cells inside the carrier. It is particularly significant for systems with cells entrapped within the carriers as opposed to systems with the cells adsorbed on the carrier surface. Internal mass transfer resistances can be minimized by adjusting the size, texture and porosity of immobilized polymer particles [Citation[6]].
Calcium-alginate gel is the primary method used in cell immobilization studies in a variety of different processes including beer production. However, as ceramics have more excellent mechanical and chemical properties than Ca-alginate gel, are not subject to microbial attack, and can be steam sterilized, reprocessed and reutilized [Citation[11], Citation[12]], it is of practical significance to evaluate yeast cells immobilized using such ceramic support. Therefore, the present investigation was carried out in order to compare the process kinetics of beer primary fermentation using yeast cells immobilized using Ca-alginate gel and ceramic supports.
MATERIALS AND METHODS
Ceramic Supports
Ceramic raw material (consisting of SiO2, Al2O3, CaO, K2O, etc.), was mixed with 10% w/v sawdust and water to allow molding into ring-shaped supports, which were calcinated at 1200°C to yield ceramic supports. The physical parameters of the product were: external diameter 5 mm, internal diameter 8 mm, porosity 53% and density 0.8 g/cm3. More detailed information on production/analysis of ceramic supports was reported previously [Citation[13]].
Yeast Cell Immobilisation
Saccharomyces cerevisiae yeast was obtained from Nanchang Can and Beer Factory, Nanchang City, Jiangxi Province, People's Republic of China. The yeast was inoculated into a medium containing glucose (2.0%, w/v), yeast extract (1.0% w/v), and peptone (2.0%, w/v), and was incubated (24 hours at 28°C). Yeast cells were concentrated by centrifugation, and finally washed with sterile NaCl solution (0.85% w/v).
Ceramic support was washed with deionized water, sterilized (by autoclaving), and placed in yeast cell suspension (yeast cells suspended in Solution A: 142 mM NaCl, 2.5 mM CaCl2, ∼ 5.8 × 109 cells/mL), permitting absorption of yeast cells into the micropores of the ceramic support (yeast cell loading ∼ 108 cells/g support).
For comparison, yeast cell suspension was mixed into Na-alginate solution (3% w/v), and the mixture injected into CaCl2 solution (1.0% w/v). This yielded a soft Ca-alginate gel containing immobilized yeast cells (∼ 108 cells/g gel), which was washed with deionized water.
Beer Primary Fermentation
The bioreactor system () included a glass bioreactor (i.d. 17 cm, 10 L total volume, and 6 L effective volume when containing ceramic support). Bioreactor temperature was maintained using a thermostatically controlled water jacket (10–12°C). Beer wort linear flow rate was also regulated (0.2–1.6 cm/min).
Ceramic support (or Ca-alginate gel) containing immobilized cells was then placed into the pre-sterilized bioreactor as a fixed bed. Fresh beer wort (10°Brix) was fed through the system as quickly as possible at 12.0°C to fill the bioreactor and the immobi1ized yeast cells were proliferated for 12 hours at 12.0°C. The beer wort used for cell proliferation was replaced with fresh beer wort (10°Brix) as required and the desired fermentation variables (fermentation temperature, linear beer wort flow rate, and immobilized yeast cell support matrix) were altered accordingly, in order to determine their effects on beer wort total soluble solids (°Brix) as a function of fermentation time (hours). Beer wort samples were removed from the reservoir for analysis at hourly intervals and their total soluble solids contents (°Brix) determined by refractometry [Citation[14]].
When the total soluble solids content of the beer wort reached ∼ 4°Brix (using the yeast cells immobilized on ceramic support and fermentation performed at C), the primary fermentation was stopped and a sample of “green” beer was removed and its ethanol and diacetyl (2,3-butanedione) concentrations determined by pycnometry [Citation[15]] and distillation/colorimetry [Citation[16]], respectively.
RESULTS AND DISCUSSION
Effect of Temperature on Beer Primary Fermentation Rate
Fresh beer wort (10°Brix, 8 L) was continuously cycled (at a linear flow rate of 0.77 cm/min) through the bioreactor (containing yeast cells immobilized on ceramic support) at 10.6°C, and a separate batch at 12.0°C. Samples were taken at hourly intervals and analyzed by refractometry, and the fermentation process was terminated when the beer wort total soluble solids contents reached ∼ 4°Brix. As expected, the total soluble solids content of beer wort (initially 10°Brix) decreased as a function of fermentation time at both 10.6°C and 12.0°C (), and the fermentation rate was visibly faster at 12.0°C (taking ∼ 11 hours to reach ∼ 4°Brix), compared with at 10.6°C (taking ∼ 13 hours to reach ∼ 4°Brix).
Figure 2 Effect of temperature on beer primary fermentation rate using yeast cells immobilized by adsorption onto ceramic support (beer wort linear flow rate of 0.77 cm/min).
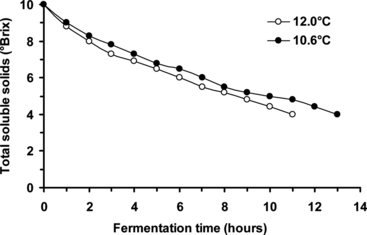
The reaction (disappearance of total soluble solids as a function of time) demonstrated first-order reaction kinetics (i.e. an exponential decrease of total soluble solids concentration as a function of time), the relationship between total soluble solids concentration (C) and time (t) being:where Ct is the total soluble solids concentration at time t, C0 is the initial the total soluble solids concentration (i.e. at time t = 0), and k is the rate constant. Equation (1) can be re-expressed as:
Therefore, for a first-order reaction, a plot of ln (Ct/C0) versus time (t) gives a straight line with a gradient of –k (). Linear regression analysis of data gives k values of 0.067 hour−1 (at 10.6°C) and 0.078 hour−1 (at 12.0°C).
Figure 3 Determination of beer primary fermentation first-order rate constants (using a plot of ln (Ct/C0) versus t) at different temperatures (10.6 and 12.0°C) using yeast cells immobilized by adsorption onto ceramic support (beer wort linear flow rate of 0.77 cm/min).
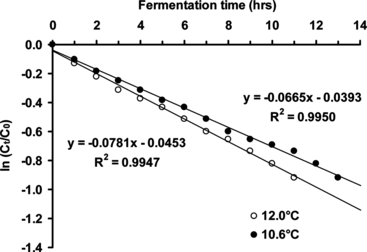
and show that for this first-order irreversible fermentation bioreaction the reaction rate increases with increasing temperature, since an increase of ∼ 17% was observed for an increase in temperature of only 1.4°C. Although the beer primary fermentation rate can thus be significantly increased by raising the temperature, care must be taken in the design of a commercial-scale bioreactor and control of the fermentation temperature/rate within proper limits so as to ensure that the produced beer has the required organoleptic properties, especially its unique flavor.
Another useful indication of the fermentation reaction rate is the time it takes for the total soluble solids concentration to fall to half its initial value (half-life, t1/2). In the case of a first-order reaction, the time for the concentration to decrease from C0 to 1/2 C0 is given by:and Equation (3) can be re-expressed as:
Therefore the half-lifes for the beer primary fermentation using a beer wort flow rate of 0.77 cm/min were ∼ 10.4 hours (at 10.6°C) and ∼ 8.9 hours (at 12.0°C). The ethanol and 2,3-butanedione concentrations of the extracted “green” beer were 2.5% w/v and 0.3 mg/L, respectively.
Effect of Beer Wort Flow Rate on Primary Fermentation Rate
Fresh beer wort batches (10°Brix, 8 L) were separately continuously cycled through the bioreactor (containing yeast cells immobilized on ceramic support) for one hour at 10.6°C, at beer wort linear flow rates in the range 0.2–1.6 cm/minute. After 1 hour wort samples were collected and their total soluble solids contents (°Brix) determined, and used to calculate initial fermentation rates (°Brix kg−1 hour−1), as a function of beer wort linear flow rate ().
Figure 4 Effect of beer wort linear flow rate on initial rate of fermentation using yeast cells immobilized by adsorption onto ceramic support (at 10.6°C).
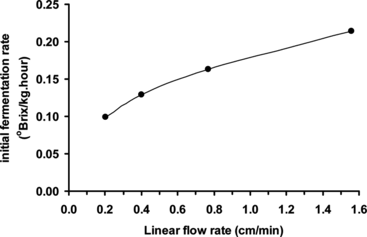
Beer wort total soluble solids content decreased during this initial hour for all beer wort linear flow rates investigated, and initial beer primary fermentation rate increased with increasing beer wort linear flow rate. This indicates that the bioreaction is influenced by mass transfer of substrate through the immobilization matrix [Citation[17]]. Increasing the flow rate assists in overcoming limiting mass transfer effects, since the substrate (beer wort sugars) can better penetrate the immobilization matrix and interact with the immobilized yeast cells. Effect of linear flow rate on rate of primary fermentation must be investigated with respect to design and operation of a commercial bioreactor.
Comparison of Ceramic and Ca-alginate Gel Supports
Fresh beer wort batches (11°Brix, 8 L) were separately continuously cycled (at a linear flow rate of 1.56 cm/min) through the bioreactor (containing yeast cells immobilized on ceramic support and Ca-alginate gel, respectively) at 10.0°C. Samples were taken at hourly intervals and analyzed by refractometry, and the fermentation process was terminated when the beer wort total soluble solids contents reached ∼ 4°Brix. As expected, the total soluble solids content of beer wort (initially 11°Brix) decreased as a function of fermentation time using both immobilized yeast cell systems (), and the fermentation rate was visibly faster for the yeast cells immobilized on ceramic support (taking ∼ 16 hours to reach ∼ 4°Brix), compared with Ca-alginate gel (taking > 21 hours to reach ∼ 4°Brix).
Figure 5 Effect of immobilization matrix (ceramic support adsorption and Ca-alginate gel entrapment) on beer primary fermentation rate using immobilized yeast cells (at 10.0°C, beer wort linear flow rate of 1.56 cm/min).
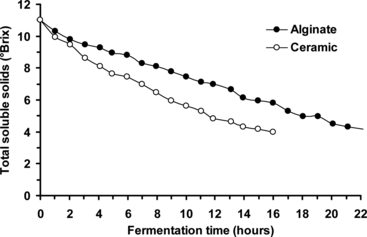
First-order reaction kinetics were again observed by plotting ln (Ct/C0) versus time (t) giving a straight line with a gradient of –k (). Linear regression analysis of data gave k values of 0.064 hour−1 (ceramic support) and 0.043 hour−1 (Ca-alginate gel). Yeast cells immobilized by adsorption onto ceramic support had a significantly higher rate of beer primary fermentation than those immobilized by entrapment in Ca-alginate gel (∼ 1.5 times faster under the same conditions).
Figure 6 Determination of beer primary fermentation first-order rate constants (using a plot of ln (Ct/C0) versus t) using yeast cells immobilized by adsorption onto ceramic support and entrapped in Ca-alginate gel (at 10.0°C, beer wort linear flow rate of 1.56 cm/min).
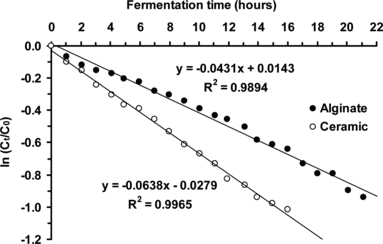
Calculated half-life (t1/2) values for the beer primary fermentation using a beer wort flow rate of 1.56 cm/min were ∼ 10.8 hours (ceramic support, 10°C) and ∼ 16.1 hours (Ca-alginate gel, 10°C), indicating that choice, design and thus support characteristics are very important.
CONCLUSIONS
Many industries have profited from application of immobilized cells, but there remain many problems to be solved. For example, some supports and methods of cell immobilization are not necessarily economic. Organic materials have mainly been used as the support in early studies and applications, such as Ca-alginate gel, but they are not optimal and fall short of the required physical and chemical properties that many industrial applications technology demands. Ceramics, on the other hand, exhibit unique physical and chemical properties that are at most only partially shown by organic supports. For example, they can be used at higher temperatures, have better structural stability without the problems of swelling, and can withstand more harsh chemical environments.
The ceramic adsorbed yeast cells, investigated with respect to their kinetics of beer primary fermentation rate in the present study, are significantly more efficient (in terms of reaction rate and half-life) than those entrapped in Ca-alginate gel as an immobilization matrix for rapid beer primary fermentation. The developed technology has wide potential application in the brewing and associated industries, and requires further development/investigation on a larger sale.
REFERENCES
- Linko, M., Haikara, A., Ritala, A., Penttilä, M. (1998). Recent advances in the malting and brewing industry. J. Biotechnol. 65: 85–98. [CSA]
- Vanderhaegen, B., Neven, H., Verachtert, H., Derdelinckx, G. (2006). The chemistry of beer aging. Food Chem. 95: 357–381. [CSA], [CROSSREF]
- Virkajarvi, I., Vainikka, M., Virtanen, H., Home, S. (2002). Productivity of immobilized yeast reactors with very-high-gravity worts. J. American Soc. Brewing Chemists 60: 188–197. [CSA]
- Willaert, R. (2000). Beer production using immobilized cell technology. Minerva Biotecnologica 12: 319–330. [CSA]
- Divies, C., Cachon, R., Cavin, J.-F., Prevost, H. (1994). Immobilized cell technology in wine production. CRC Crit. Rev. Biotechnol. 14: 135–153. [CSA]
- Pilkington, P.H., Margaritis, A., Mensour, N.A., Russell, I. (1998). Fundamentals of immobilised yeast cells for continuous beer fermentation: A review. J. Inst. Brewing 104: 19–31. [CSA]
- Nedović, V.A., Durieux, A., Van Nedervelde, L., Rosseels, P., Vandegans, J., Plaisant, A.-M., Simon, J.-P. (2000). Continuous cider fermentation with co-immobilized yeast and Leuconostoc oenos cells. Enzyme Microb. Technol. 26: 834–839. [CSA], [CROSSREF]
- van Iersel, M.F.M., Meersman, E., Arntz, M., Rombouts, F.M., Abee, T. (1998). Effect of environmental conditions on flocculation and immobilization of Brewer's yeast during production of alcohol-free beer. J. Inst. Brewing 104: 131–136. [CSA]
- van Iersel, M.F.M., van Dieren, B., Rombouts, F.M., Abee, T. (1999). Flavor formation and cell physiology during the production of alcohol-free beer with immobilized Saccharomyces cerevisiae. Enzyme Microb. Technol. 24: 407–411. [CSA], [CROSSREF]
- Nedović, V.A., Obradović, B., Leskošek-Čukalović, I., Trifunović, O., Pešić, R., Bugarski, B. (2001). Electrostatic generation of alginate microbeads loaded with brewing yeast. Process Biochem. 37: 17–22. [CSA], [CROSSREF]
- Weethall, H.H., Detar, C.C. (1974). Studies on aminoacylase immobilised on porous ceramic carriers. Biotechnol. Bioeng. 16: 1537–1544. [CSA], [CROSSREF]
- Cabral, J.M.S., Novais, J.M., Kennedy, J.F. (1986). Immobilisation studies of whole metal activated inorganic supports. Appl. Microbiol. Tech. 23: 157–262. [CSA]
- Zhang, Y., Kennedy, J.F., Knill, C.J. (1996). Kinetic analysis of ethanol production from glucose fermentation by yeast cells immobilised onto ceramic supports. J. Biomater. Sci.–Polymer Edn. 7: 1119–1126. [INFOTRIEVE], [CSA]
- Clarke, M.A. (ed.) (1990). 932.14 Solids in syrups C. By means of refractometer, in Official Methods of Analysis of the Association of Official Analytical Chemists (AOAC), 15th Edition, Volume 2: Food Composition; Additives; Natural Contaminants, K. Helrich, Ed., AOAC: Arlington, Chapter 44, pp. 1011–1012.
- Gales, P.W. (Ed.) (1990a). 935.22 Alcohol (by weight) in beer–specific gravity method, in Official Methods of Analysis of the Association of Official Analytical Chemists (AOAC), 15th Edition, Volume 2: Food Composition; Additives; Natural Contaminants, K. Helrich, Ed., AOAC: Arlington, Chapter 27, p. 710.
- Gales, P.W. (Ed.) (1990b). 978.11 Diacetyl in beer–colorimetric method, in Official Methods of Analysis of the Association of Official Analytical Chemists (AOAC), 15th Edition, Volume 2: Food Composition; Additives; Natural Contaminants, K. Helrich, Ed., AOAC: Arlington, Chapter 27, p. 710.
- Bennet, C.O., Myers, J.E. (1982). Momentum, Heat and Mass Transfer, McGraw Hill: New York, pp. 550–559.