Abstract
The objective of this study was to assess the in vitro and in vivo degradation properties of macroporous sponges composed of oxidized acetyl-cellulose (AC; 45.000 Mw) and ethyl-cellulose (EC; 50.000 Mw). The sponges were constructed by solvent-casting and particulate-leaching technique using a polymer concentration of 2.5 and 5.0% (w:v), and periodate oxidation. The resulting sponges were: AC2.5, AC5.0, EC2.5 and EC5.0. While AC sponges exhibited a gradual degradation overtime, EC sponges had a very slow in vitro mass loss. In general, sponges made up of 2.5% (w:v) polymer content degraded faster than the ones with 5.0% (w:v). The sponges degraded faster at pH 5.0, compared to pH 6.0 and 7.4 conditions. About 60%, 44% and 31% of dry mass loss was determined for AC2.5 sponges after 60 weeks at pH 5.0, pH 6.0 and pH 7.4 conditions, respectively; thus, ca. 21%, 13% and 12% of dry mass loss from EC2.5 sponges was observed at the same pH conditions, in the same order. The in vivo degradation studies were performed on Wistar rats (n = 24) for a duration of 60 weeks. In general, all sponge implants were well-tolerated by the subjects. While granulation tissue or fibrotic capsule was not formed around the sponges, neovascularization was observed. AC and EC sponges demonstrated an in vivo degradation behavior quite similar to that observed for the in vitro study conducted at pH 5.0 conditions. Histomorphometric analysis revealed that the in vivo degradation of AC2.5 and EC2.5 after 60 weeks was about 47% and 18%, respectively. The results indicate that oxidized acetyl cellulose may be considered as a partially degradable scaffold material for tissue engineering applications.
INTRODUCTION
The concept of tissue engineering technology is the transplantation of three-dimensional constructs consisting of cells grown ex vivo on predesigned scaffolds for tissue repair and regeneration [Citation[1], Citation[2]]. Highly porous scaffolds are an integral part of tissue engineering since they act as a boundary to retain transplanted cells in the defect as well as provide a substrate for migration and attachment of anchorage-dependent cells [Citation[3-7]], and permit neovascularization of the developing tissue [Citation[8], Citation[9]]. Studies have shown that polymers that permit modulation of their mechanical properties, porosity, and degradation time are ideal; thus, resorption of polymeric scaffolds by time can eliminate problems associated with permanent implants [Citation[1], Citation[3]].
Cellulose and its derivatives have been widely used in several biomedical applications: cellulose acetate, an amorphous thermoplastic polymer, has been used in constructing hemodialysis membranes [Citation[10], Citation[11]], oxidized cellulose gauze as hemostatic agent [Citation[12]]; regenerated cellulose has been considered in medical domains for bone sites and has been investigated as a diaphyseal obturator in orthopedic surgery [Citation[13], Citation[14]]. The biocompatibility and osteoconductive properties of regenerated cellulose and its derivatives have previously been reported [Citation[13], Citation[15], Citation[16]]. Recently, a resorbable form of regenerated cellulose was reported where macroporous sponge material was oxidized using periodic acid [Citation[17]].
Cellulose acetate (acetyl cellulose) and ethyl cellulose have hardly been considered as scaffold materials for tissue engineering applications, since they are known to degrade very slowly, or are practically non-resorbable, in vivo. This is related to the lack of enzymes in the human circulation, targeting the bonds in their chemical structure [Citation[18]]. A hydrophilic backbone, hydrophilic endgroups, and more reactive hydrolytic groups in the backbone are the major factors that accelerate polymer degradation [Citation[19]]. Periodate has a regioselective action by way of oxidation of the C2 and C3 atoms in the glucopyranose units [Citation[18], Citation[20]], thereby forming a structure with two aldehyde groups that are less stable at physiological conditions. Besides the chemical structure, the level of crystallinity, the molecular weight, the porosity, shape and size of the device are other important variants that may modulate polymeric implant degradation [Citation[19], Citation[21]].
Here, the degradation of macroporous polymer sponges composed of oxidized acetyl cellulose and ethyl cellulose were evaluated for a duration of 60 weeks, both under in vitro and in vivo conditions. The in vitro degradation was investigated under three different conditions at pH 5.0, 6.0, and 7.4, by calculating the dry mass loss of the constructs. The in vivo degradation was investigated at the rat subcutaneous epigastric groin fascia model by using histology, SEM, and histomorphometry.
MATERIALS AND METHODS
Preparation of the Sponges
Macroporous sponges were fabricated by a modified salt-leaching process [Citation[3]]: briefly, either acetyl cellulose (AC; 45,000 Mw; 40% acetyl content; Sigma) or ethyl cellulose (EC; 50,000 Mw; 48–49.5% ethoxy content; Sigma) dissolved in acetone (2.5 and 5.0%, w:v) were loaded into Teflon molds packed with NaCl crystalline particles sieved to a size of 250–400 µm. The solvent was allowed to evaporate and the salt was removed by immersing the sponges in lukewarm running distilled water. After drying in a vacuum oven, they were cut into cylindrical shape (Φ = 4 mm, h = 2 mm), and then oxidized with a 0.5 M periodate (HIO4) solution at 40°C in the dark for 90 minutes [Citation[17], Citation[20]]. The sponges were washed in distilled water, sterilized overnight in 70% (v/v) ethanol, and washed three times in PBS prior to implantation.
In Vitro Degradation Experiments
In vitro degradation of polymer sponges was studied in buffers of pH 7.4 (0.1 M PBS), pH 6.0 and pH 5.0 (0.1 M acetate-buffered saline) at 37°C up to 60 weeks, in 15 mL-sterile dissolution containers with screw caps, under static conditions. Samples were removed at set time points, and the mass loss (ML) was determined by using the following formula, ML = [(W0 − Wt)/W0] × 100%, where W0 = initial dry mass, and Wt = dry mass at a given time point. The degradation medium was replaced with fresh buffer every week. Medium changes were performed to obtain an infinite sink condition during the experiments.
In Vivo Studies
A total of 24 male adult Wistar strain rats weighing 175–225 g at the time of implantation were used in the experiments designed to investigate the in vivo degradation and tissue response of the sponges. The rats were housed in duplicates in cages with free access to food pellets and drinking water. All experimental protocols involving animals were conducted according to the standards of international regulations.
Sponges were implanted into the groin fascia of the rats after Avertine anesthetia. Briefly, 3-cm-long incisions were made bilaterally through the skin and the sponge implants moistened with 0.85% NaCl solution were inserted into the right and left groin fascia at the furthest possible distance from the incision. Care was taken to prevent contact between the implant and the incision site. After the addition of 0.1 mL sodium cefazolin antibiotic (0.4 g/mL, Kefzol, Lilly and Co, Indianapolis, IN, USA), the skin was sutured using 6.0 EthilonTM (Ethicon, NJ, USA) sutures and closed using 9 mm wound clips (Becton Dickinson, Sparks, MD). Animals were numbered and held for 10 to 60 weeks, and the implants were removed with some of the surrounding tissue at time points and transferred into PBS for washing, and then fixed either for histology or SEM.
Histology and In Vivo Implant Evaluation
Light microscopy was performed to evaluate the level of implant degradation. The samples were fixed in formalin (2.5% in PBS at pH 7.4), embedded in paraffin, sectioned and then stained with hematoxyline and eosin. The wound healing response and neovascularization at the implantation interfaces were also evaluated.
Scanning Electron Microscopy
Sponges were prepared for SEM using routine methods. Samples were fixed in 2.5% glutaraldehyde in 0.1 M cacodylate buffer (pH 7.4) at 4°C, dehydrated in a graded ethanol series, mounted on aluminum supports and were sputter-coated with gold using a Sputter Coater (Desk II, Denton Vacuum, Cherry Hill, NJ, USA). A JEOL JSM 5600 (Tokyo, Japan) electron microscope was utilized to image samples at a voltage of 20 kV.
Statistical Analysis
The in vitro quantitative results were obtained from at least triplicate samples. Data were expressed as the mean ± SD. Histomorphometric measurements were performed on technically best sections to determine the in vivo degradation of sponges at 10, 30 and 60 weeks after implantation. The images were processed and analyzed by using the Leica QWin Plus (Germany) software. For each experimental group, 16 counts from 8 different histology sections were performed. Statistical analysis was carried out using the unpaired Student's t test for the histomorphometric data. A value of P < 0.05 was considered to be statistically significant.
RESULTS
In Vitro Studies
The in vitro degradation of the constructs by time are presented in . Results showed that the in vitro degradation rate of AC and EC sponges after 60 weeks was in the range of about 10–60%; and the mass loss at pH 5.0 was found to be higher than that of the pH 6.0 and pH 7.4 buffer conditions.
Figure 1 Relative mass loss of sponges over time at pH 7.4 (in 0.1 M phosphate buffered saline) under infinite sink conditions at 37°C.
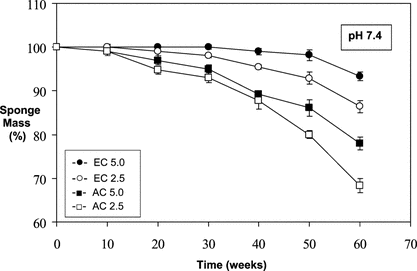
Figure 2 Relative mass loss of sponges over time at pH 6.0 (in 0.1 M acetate buffered saline) under infinite sink conditions at 37°C.
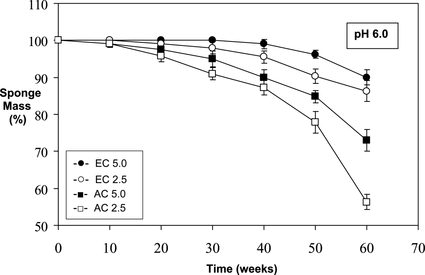
Figure 3 Relative mass loss of sponges over time at pH 5.0 (in 0.1 M acetate buffered saline) under infinite sink conditions at 37°C.
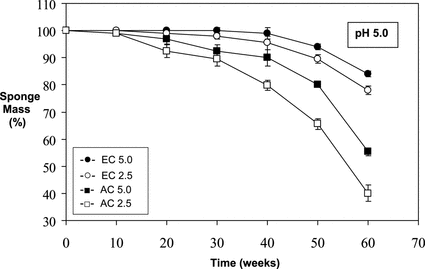
The results of the in vitro degradation test carried out in PBS (pH 7.4, 37°C) are given in . As can be seen from the figure, EC5.0 sponge did not demonstrate an appreciable dry mass loss for the duration of the experiments; thus the situation for EC2.5 sponges was quite similar up to 30 weeks, and then a ∼ 12–14% of mass loss was observed after 60 weeks. The AC5.0 and AC2.5 sponges had mass losses of ∼ 20–22% and ∼ 30–33% respectively, after same period of time ().
shows the results of the in vitro degradation test performed at pH 6.0 acetate buffer conditions (37°C). It is clear that while EC sponges lost < 15% of their dry mass, AC sponges had mass losses of ∼ 24–30% and ∼ 42–46% (AC5.0 and AC2.5, respectively), after 60 weeks ().
The highest level of in vitro degradation was observed in the experiment performed at pH 5.0 conditions (acetate buffered saline; 37°C) (). As can be seen from the figure, after 60 weeks, mass losses of ∼ 56–62% and ∼ 44–45% were calculated for AC2.5 and AC5.0 sponges, respectively. Macroscopical deformation and degradation was also evident in the AC sponges retrieved at 60 weeks, both under pH 6.0 and pH 5.0 buffer conditions (not shown).
In Vivo Studies
Two animals (out of 24) died due to complications of anaesthesia. None of the implants were infected, and none of the subjects in the groups showed any sign of distress or reaction for the duration of experiments.
Histology results from the in vivo experiments are presented in . Histology confirmed that none of the implants showed significant level of tissue reaction at or around the transplantation sites (). Implant-host tissue interfaces lacked capsule formation. In general, AC sponges showed a much higher rate of in vivo degradation compared to that of EC sponges ( and ). Cells invaded the pores of all sponges and neovascularization process occurred in time.
Table 1. Histomorphometric analysis on the in vivo degradation of AC and EC spongesFootnote[1]
Figure 4 Histological appearance of sponge implants retrieved: (A) after 10 weeks [AC5.0]; (B) after 10 weeks [AC2.5]; (C) after 30 weeks [AC2.5]; (D) higher magnification of C; (E) adjacent tissue at 60 weeks post-implantation [AC2.5]; (F) higher magnification of E; P: polymer, arrows: capillaries; FP: fat pad; H&E-stainings; scale bars = 100 µm.
![Figure 4 Histological appearance of sponge implants retrieved: (A) after 10 weeks [AC5.0]; (B) after 10 weeks [AC2.5]; (C) after 30 weeks [AC2.5]; (D) higher magnification of C; (E) adjacent tissue at 60 weeks post-implantation [AC2.5]; (F) higher magnification of E; P: polymer, arrows: capillaries; FP: fat pad; H&E-stainings; scale bars = 100 µm.](/cms/asset/3c61ad7d-b99f-4f9e-96f4-58aa8511d335/ianb19_a_176927_f0004_b.gif)
Ten weeks after implantation, all the sponges (AC and EC) were filled with fibroblasts and connective tissue. Some cracks and fissures started to appear in the thinnest parts of the pore walls of AC2.5 and AC5.0 implants ( and ). Some infammatory cells could be observed at the vicinity of these sponges.
Thirty weeks after implantation, the border between the fronts of the AC2.5 sponge walls and host tissue was not clearly seen; the contours of the pore walls had already softened ( and ). The cellularity of the host tissue demonstrated an ongoing degradation; some new-formed capillaries were observed near the AC2.5 sponge implants. Sixty weeks after implantation, the neovascularization had progressed and the remnants of the AC2.5 sponges had decreased.
Data from the histomorphometrical measurements are given in . Histomorphometrical analysis demonstrated comparable results with the in vitro degradation experiments, in terms of polymer degradation (AC versus EC). Statistically significant differences (P < 0.05) in mass loss were found between the sponge specimens retrieved at 10 and 60 weeks, in groups AC2.5, AC5.0 and EC2.5. The rate of mass loss of EC sponges was significantly lower, reaching only 11.6 ± 2.9% (EC2.5) and 8.8 ± 3.6% (EC5.0) in 60 weeks. The percentage of mass loss of the AC sponges implanted in the rat groin fascia in 60 weeks, as shown in , was calculated to be approximately 41.1 ± 3.3% (AC2.5) and 25.2 ± 2.8% (AC5.0).
and show representative scanning electron micrographs of AC5.0 and AC2.5 sponges prior to implantation, respectively. The structures consisted of pores with diameters ranging from approximately 250 to 400 µm. The pores were interconnected, thereby forming a three-dimensional porous network (, ). The appearance of the contours and fissures in the thinner parts of the pore walls of AC5.0 sponge (5A) seemed to be tougher than the ones in the AC2.5 sponge (5B).
Figure 5 SEM images of sponge implants: (A) new prepared [AC5.0]; (B) new prepared [AC2.5]; (C) 10 weeks post-implantation [AC2.5]; (D) 30 weeks post-implantation [AC2.5]; (E) 60 weeks post-implantation [AC2.5].
![Figure 5 SEM images of sponge implants: (A) new prepared [AC5.0]; (B) new prepared [AC2.5]; (C) 10 weeks post-implantation [AC2.5]; (D) 30 weeks post-implantation [AC2.5]; (E) 60 weeks post-implantation [AC2.5].](/cms/asset/077eb4c8-e9c4-4ac8-89b0-cdbd486c0d23/ianb19_a_176927_f0005_b.gif)
Following implantation, the macroporous sponges were infiltrated by the host connective tissue (–). Supporting the histological analysis (), SEM also demonstrated a gradual in vivo degradation of the AC sponges by time; , and corresponding to micrographs taken at weeks 10, 30, and 60, respectively.
DISCUSSION
The aim of this particular study was to evaluate both the in vitro and in vivo degradation properties of macroporous sponges made up of oxidized acetyl- and ethyl-cellulose. Degradation is referred to as the process whereby implanted polymeric material experiences a decrease in its mechanical properties and/or mass [Citation[22]]. The decrease in the cross-sectional area and the changes in the structure of pore walls indicated that EC sponges degraded much slower in tissues compared to AC sponges. On the other hand, the time needed for the total disappearance of the AC sponge (AC2.5) from the subcutaneous tissue was longer than the 60-weeks observation period. In vivo degradation of the sponges resembled the in vitro degradation profile obtained from the experiment conducted in acetate buffer at pH 5.0, which might have partially simulated the acidic microenvironment caused by the activated macrophages.
Degradation is usually a combination of chemical, biological and mechanical processes. It is possible that weakening by the chemical and biological factors continued with mechanical stress, which shattered the pore walls, giving better accessibility to the chemical and biological factors. EC sponge, implanted for a short period (e.g. < 10 weeks), may be regarded as a stable material without significant degradation. On the other hand, the AC sponge used as a permanent implantable scaffold for tissue engineering can be recognized as partially degradable.
In theory, an implantable biomaterial should not induce a harmful foreign body reaction, must be free of potential infection risks, should have the needed mechanical properties, such as flexibility and strength, and be storable and readily available when needed. The readily available sponge implants used in this study were neither infected, nor induced a harmful foreign body reaction. A certain level of inflammation should have progressed, although fibrinous network formation and leukocyte accumulation, which are the early phase signs of inflammation, were not detected in the neighborhood of the sponge walls in the histology specimens; thus, the earliest specimens were collected at the tenth week of experiments.
The speed of the connective tissue ingrowth not only depends on the size, shape and internal structure of the implant, but on the age, sex and species of the experimental animal as well [Citation[23]]. In our case, the implants had a macroporous structure, which permitted the easy access of the host tissue infiltration at the subcutaneous epigastric groin fascia of young male Wistar rats.
In conclusion, this study demonstrates that a partially degradable polymer sponge can be constructed from periodate-oxidized acetyl cellulose and by using the salt-leaching technique. AC sponges may have potential in tissue engineering applications where a longer term and partial degradation is acceptable; thus ongoing cell-interaction studies at our laboratory with AC sponges should give some of the answers. Related to its very low degradability, ethyl cellulose-derived sponge seems not suitable to tissue engineering; however, it may be considered as a support material in bioartificial organ devices.
The support of the Gazi University BAP is acknowledged. Special thanks to Professor Y. M. Elçin for the technical help in fabricating the sponges.
REFERENCES
- Vacanti, J.P., Langer, R. (1999). Tissue engineering: The design and fabrication of living replacement devices for surgical reconstruction and transplantation. Lancet 354(sI): 32–34. [CSA]
- Elçin, Y.M. (2003). Tissue Engineering, Stem Cells and Gene Therapies. AEMB Series, 534 Kluwer Academic-Plenum Publishers: , NY, 1st Ed.
- Mikos, A.G., Thorsen, A.J., Czerwonka, L.A., Bao, Y., Langer, R. (1994). Preparation and characterization of poly (L-lactic acid) foams. Polymer 35: 1068–1077. [CSA], [CROSSREF]
- Elçin, A.E., Elçin, Y.M., Pappas, G.D. (1998). Neural tissue engineering: Adrenal chromaffin cell attachment and viability on chitosan scaffolds. Neurol. Res. 20: 648–654. [CSA]
- Elçin, Y.M., Dixit, V., Lewin, K., Gitnick, G. (1999). Xenotransplantation of fetal porcine hepatocytes in rats using a tissue engineering approach. Artif. Organs 23: 146–152. [CSA], [CROSSREF]
- Elçin, Y.M., Elçin, A.E., Pappas, G.D. (2003). Functional and morphological characteristics of bovine adrenal chromaffin cells on macroporous poly (DL-lactide-co-glycolide) scaffolds. Tissue Eng. 9(5): 1047–1056. [CSA], [CROSSREF]
- Inanç, B., Elçin, A.E., Elçin, Y.M. (2006). Osteogenic induction of human periodontal ligament fibroblasts under two and three-dimensional culture conditions. Tissue Eng. 12(2): 257–266. [CSA], [CROSSREF]
- Elçin, Y.M., Dixit, V., Gitnick, G. (2001). Extensive in vivo angiogenesis following controlled release of human vascular endothelial cell growth factor: Implications for tissue engineering and wound healing. Artif. Organs 25(7): 558–565. [CSA], [CROSSREF]
- Elçin, A.E., Elçin, Y.M. (2006). Localized angiogenesis induced by human vascular endothelial growth factor-activated PLGA sponge. Tissue Eng. 12(4): 959–968. [CSA], [CROSSREF]
- Stamatialis, D.F., Dias, C.R., de Pinho, M.N. (2001). Structure and permeation properties of cellulose esters asymmetric membranes. Biomacromolecules 1(4): 564–570. [CSA], [CROSSREF]
- Riekerink, M.B., Engbers, G.H., Wessling, M., Feijen, J. (2002). Tailoring the properties of asymmetric cellulose acetate membranes by gas plasma etching. J. Colloid Interface Sci. 245: 338–348. [CSA], [CROSSREF]
- Raccuia, J.S., Simonian, G., Dardik, M., Hallac, D., Raccuia, S.V., Stahl, R., Dardik, H. (1992). Comparative efficacy of topical hemostatic agents in a rat kidney model. Am. J. Surg. 163: 234–238. [INFOTRIEVE], [CSA], [CROSSREF]
- Fricain, J.C., Granja, P.L., Barbosa, M.A., de Jeso, B., Barthe, N., Baquey, C. (2002). Cellulose phosphates as biomaterials. In vivo biocompatibility studies. Biomaterials 23: 971–980. [INFOTRIEVE], [CSA], [CROSSREF]
- Märtson, M., Viljanto, J., Hurme, T., Laippala, P., Saukko, P. (1999). Is cellulose sponge degradable or stable as implantation material? An in vivo subcutaneous study in the rat. Biomaterials 20: 1989–1995. [CSA], [CROSSREF]
- Miyamoto, T., Takahashi, S., Hiraku, I., Hiroshi, I., Noishiki, Y. (1989). Tissue biocompatibility of cellulose and its derivatives. J. Biomed. Mater. Res. 23: 125–133. [INFOTRIEVE], [CSA], [CROSSREF]
- Märtson, M., Viljanto, J., Hurme, T., Laippala, P., Saukko, P. (1998). Biocompatibility of cellulose sponge with bone. Eur. Surg. Res. 30: 426–432. [CSA], [CROSSREF]
- Laurence, S., Bareille, R., Baquey, C., Fricain, J.C. (2005). Development of a resorbable macroporous cellulosic material used as hemostatic in an osseous environment. J. Biomed. Mater. Res. 73A: 422–429. [CSA], [CROSSREF]
- Nevell, T.P. (1985). Oxidation of cellulose, in Cellulose Chemistry and its Applications, T.P. Nevell, S. Haig-Zeronian, Eds., John Wiley & Sons: New York, pp. 243–263.
- Daniels, A.U., Chang, M.K.O., Andriano, K.P. (1990). Mechanical properties of biodegradable polymers and composites proposed for internal fixation of bone. J. Appl. Biomat. 1: 57–78. [CSA], [CROSSREF]
- Dimitrijevich, S.D., Tatarko, M., Gracy, R.W., Linsky, C.B., Olsen, C. (1990). Biodegradation of oxidized regenerated cellulose. Carbohydr. Res. 195: 247–256. [INFOTRIEVE], [CSA], [CROSSREF]
- Durkut, S., Elçin, A.E., Elçin, Y.M. (2006). Biodegradation of chitosan-tripolyphosphate beads: In vitro and in vivo studies. Artif. Cells Blood Substit. Immobil. Biotechnol. 34(2): 263–276. [INFOTRIEVE], [CSA], [CROSSREF]
- Göpferich, A. (1996). Mechanism of polymer degradation and erosion. Biomaterials 17: 103–114. [CSA], [CROSSREF]
- Pajulo, O., Viljanto, J., Lönnberg, B., Hurme, T., Lönnqvist, K., Saukko, P. (1996). Viscose cellulose sponge as an implantable matrix: Changes in the structure increase the production of granulation tissue. J. Biomed. Mater. Res. 32: 439–446. [INFOTRIEVE], [CSA], [CROSSREF]