Abstract
Poly(acrylic acid) (PAA)-grafted cellulose copolymer beads were synthesized and tested in vitro as an adsorbent for selective removal of low-density lipoprotein (LDL) from human plasma. The copolymers were prepared by graft copolymerization of acrylic acid (AA) onto porous cellulose beads using cerium ammonium nitrate (CAN) as an initiator. The effect of initiator concentration, monomer amount and reaction time on the grafting was examined, and it revealed that the extent of grafting could be controlled by setting the appropriate reaction conditions. In vitro batch-wise adsorption tests were conducted to evaluate the lipoprotein sorption properties of the resulted copolymer beads, and the effect of grafting conditions on the adsorption performance was investigated. It was shown that the binding capacities of the best adsorbent derived from the appropriate reaction conditions could reach 4.96 mg/g total cholesterol (TC) and 4.46 mg/g LDL cholesterol (LDL-C) from human plasma, respectively, without significantly affecting the contents of beneficial constitutes such as high-density lipoprotein (HDL) and total proteins (TP). The influences of plasma amount and adsorption period on the adsorption properties were also determined and analyzed. It appears that this kind of copolymer is worthy of being developed as an alternative LDL adsorbent.
INTRODUCTION
LDL particles are the main carriers of cholesterol in the human circulation. Elevated level of LDL has been widely recognized as a major risk factor for developing atherosclerosis and coronary heart disease [Citation[1]]. Since the 1970s, LDL apheresis has gradually become the last resort treatment for patients with homozygous and severe heterozygous familial hypercholesterolemia (FH) who do not sufficiently respond to diets and hyperlipidemic drugs [Citation[2]]. In the past thirty years, the primitive and nonspecific plasma exchange has increasingly been replaced by several more effective and selective techniques in clinical use, such as cascade or double filtration, heparin-induced extracorporeal LDL precipitation (HELP), and specific or selective adsorption of LDL [Citation[3]]. Among those, selective adsorption of LDL from plasma, which includes immuoadsorption using anti-LDL antibodies immobilized agarose beads [Citation[4]] and chemoadsorption onto cellulose beads bearing dextran sulfate [Citation[5]], has been proved to be a better option with higher selectivity and fewer side-effects. Especially in the most recent years, LDL hemoperfusion known as direct adsorption of lipoprotein has been invented and offers the advantages of selective adsorption more obvious for its simple procedure [Citation[6], Citation[7]]. However, the wide application of those adsorbents mentioned above has been greatly limited by their drawbacks, such as expensive costs, complicated preparation or instability of bio-ligands. Therefore, recently extensive research attention has focused on the development of other LDL adsorptive materials in order to overcome the above limitations [Citation[8-17]]. PAA immobilized polyacrylamide beads have been successfully used in LDL hemoperfusion, which were fabricated by covalently coupling PAA with a proper molecular weight onto porous polyacrylamide supports [Citation[6]]. It suggested that PAA could be used as effective ligands for LDL binding, and other LDL adsorbents might be obtained by utilizing some readily obtained matrix and introducing PAA via some simple procedures. According to this idea, an LDL adsorbent was synthesized by graft copolymerizing of AA onto cross-linked porous chitosan beads, and the resulting copolymer beads showed good LDL specificity [Citation[18]]. The LDL binding capacity of this adsorbent might be further elevated by moderately decreasing the amount of grafted PAA, for redundant PAA might block the pores across the matrix, hence preventing LDL particles from diffusing into the inner surface. However, since chitosan exhibited high graft activity, relatively lower amounts of grafted PAA could not be readily controlled.
In this paper, porous cellulose beads were chosen as the matrix instead of chitosan beads, since cellulose should be less active than chitosan while being copolymerized with AA. Furthermore, cellulose beads, featuring good biocompatibility and mechanical strength even without being cross-linked, have already been effectively used as the supports of LDL adsorbents [Citation[5]]. PAA-grafted cellulose beads were synthesized simply through graft copolymerizing of AA onto cellulose beads with CAN as a redox initiator. A few basic aspects for the grafting were examined, and the effect of grafting conditions on the lipoprotein sorption performance was investigated. The adsorbent derived from the appropriate grafting conditions gave satisfactory LDL binding capacity.
MATERIALS AND METHODS
Materials
Porous cellulose beads were synthesized by a reported method [Citation[19]]. AA with analytical grade purchased from Tianjin No. 2 Chemical Reagent Plant (Tianjin, China) was distilled to remove inhibitor before use. CAN was of analytical grade obtained from Tianjin Damao Chemical Reagent Plant (Tianjin, China). All other chemical reagents were of analytical grade and used as received. Plasma used in adsorption tests was obtained from Tianjin No. 2 Adjunctive Hospital. Biochemical reagent kits for TC, LDL-C, HDL-cholesterol (HDL-C) and total protein (TP) assays were purchased from Beijing Zhongsheng High Tech Bioengineering Company (Beijing, China).
Preparation of PAA-grafted Cellulose Beads
PAA-grafted cellulose beads were prepared according to the studies on graft copolymerizing of AA onto cellulose power or fiber [Citation[20], Citation[21]]. 1 g of suction-dried cellulose beads were put into a 10 mL glass bottle with tight lid containing a known amount of CAN dissolved in 0.14 M nitric acid solution. The mixture was shaken under nitrogen atmosphere at room temperature for 2 h. The beads were then filtered out, and washed with distilled water to remove remnant cerium ions. To the Ce-initiated beads in the bottle, a preset volume of 0.28 M nitric acid solution and 30 mg of copper power acted as homopolymer inhibitor were added. The mixture was then heated to 35°C under nitrogen atmosphere and shaken for 10 min before a definite volume of AA was injected, with the total volume of reaction medium in the bottle kept to 8 mL. The graft copolymerization reaction was allowed to proceed for a certain time. At the end of the reaction, the final beads were filtered out and washed with methanol and distilled water successively, followed by being extracted with distilled water in a Wheaton Soxhlet extractor for 24 h to remove homopolymers. The resultant PAA-grafted beads were stored at 4°C for further use.
The copolymer beads with different PAA grafting degree were obtained by varying the concentrations of CAN and AA, or the reaction time.
IR Spectroscopy Analysis
The IR spectra of dried copolymer beads were recorded by a FT-IR spectrometer (FTS 6000, Bio-Rad). For the purpose of comparison, the IR spectra of xerogel from cellulose beads without grafting modification were also determined at the same time.
Determination of Grafting Percentage
PAA grafting percentage (GP) was calculated according to the following equation:where W1 and W2 are the weights of dried cellulose beads and dried PAA-grafted beads, respectively.
In Vitro Adsorption Properties
Batch-wise adsorption tests were employed to measure in vitro sorption properties of the copolymers for binding plasma components. Typically, 0.5 g of suction-dried beads, which were previously equilibrated with sterilized 0.9% NaCl solution, were incubated with 2.0 mL of human plasma (TC content was in the range of about 150–200 mg/dL) at 37°C for 3 h. The concentrations of TC, LDL-C, HDL-C and TP in plasma samples before and after adsorption were determined, respectively, by enzymatic colorimetric methods using the corresponding test kits and a UV-Vis spectrophotometer (UV762, Shanghai Precision & Scientific Instrument Co., Ltd, China). Adsorption percentage (AP) and adsorption capacity (AC) were calculated by the following equations:where C0, C are the concentrations of the related components (TC, LDL-C, HDL-C and TP) in plasma samples before and after adsorption, respectively; Vp and W are the volume of the plasma and the weight of the beads used during adsorption, respectively. All the AC and AP were presented with a mean and a standard deviation based on three test runs.
RESULTS AND DISCUSSION
Preparation and Characterization of Copolymer Beads
Graft copolymerization of AA and other vinyl monomers onto different cellulose materials, such as cellulose-based powder, fibers and membranes, has been extensively studied [Citation[22-25]]. CAN was often chosen as a redox initiator, and the grafting mechanism was given by Yoshida et al. [Citation[25]]. However, grafting onto porous cellulose beads has been rarely reported. Liu et al. [Citation[26]] and Du et al. [Citation[27]] studied the graft copolymerization of acrylonitrile onto cellulose beads, respectively, and the resulted copolymers were further converted to ion exchangers. In this paper, we studied the graft copolymerization of AA onto cellulose beads using CAN as an initiator. The obtained copolymers were analyzed by IR spectroscopy and the grafting conditions were examined.
Infrared Spectroscopy Analysis
The IR spectra of dried samples of cellulose and PAA-grafted cellulose beads are shown in . Compared with Fig. , it can be found from Fig. that the two adsorption bands at 1022 and 1072 cm−1 (skeletal vibrations), which were assigned to the characteristics of saccharide structure, were still remaining. This suggested that the grafting reaction did not destroy the main structure of cellulose. In Fig. , a new strong absorption that appeared at around 1716 cm−1 was attributed to the C=O stretching of PAA. The presence of this absorption confirmed that PAA had been successfully grafted onto the cellulose backbone.
Effect of Reaction Conditions on the PAA PG
Several factors, including initiator concentration, AA concentration and reaction time on the grafting, were investigated and the results were plotted in , , and . It was obvious that the amount of PAA grafted onto cellulose beads could be controlled within a quite wide range via varying grafting conditions. The grafting could even give PAA GP as low as 2.5%, in contrast with graft copolymerization of AA onto chitosan beads, where PAA GP lower than 50% was difficult to achieve [Citation[18]].
Figure 2 Effect of CAN concentration on PAA grafting percentage. AA: 1.06 mol/L; reaction time: 3.5 h; reaction temperature: 35°C.
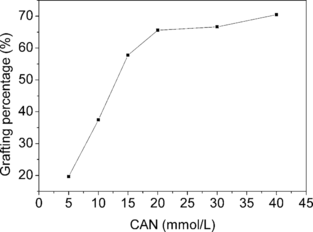
In Vitro Adsorption Tests
Effect of PAA GP on Adsorption of Lipoproteins
Through varying AA concentration in grafting reactions from 0.09 to 0.46 mol/L, the copolymers with different PAA GP were obtained in order to investigate how the amount of grafted PAA influenced their lipoprotein binding behaviors. lists the change of the lipoprotein sorption with PAA GP. The adsorption of LDL-C and TC onto PAA-grafted beads increased gradually with the increase of PAA GP, then decreased after reaching a peak. This can be explained as follows. Different from the bare cellulose beads that adsorbed LDL via rather weak hydrogen-bond interaction, the grafted PAA on the cellulose beads could act with LDL through electrostatic interaction between polyanions and the residues of basic amino acids rich on some regions of LDL particle surface, thus increasing the adsorption amount of LDL-C and TC. At the same time, the grafted PAA also acted as spacer arms, which favored LDL binding via reducing steric hindrance. However, excessive grafted PAA would badly block the internal pores of beads, thus hindering LDL particles from diffusing into pores and approaching the internal adsorptive sites [Citation[18]]. In this study, we found that PAA GP greater than 15% led to a rapid decreasing of LDL adsorption (data not presented). Therefore, there was an optimal amount of grafted PAA on cellulose beads for enhancement of LDL binding. On the other hand, the introduction of PAA seemed to have little influence on the adsorption of HDL-C.
Table 1 Dependence of lipoprotein sorption on PAA grafting percentage
Effect of Plasma Amount on Adsorption of Lipoproteins
shows the dependence of lipoprotein uptake on the amount ratio of adsorbent mass to plasma volume employed in the adsorption tests. With the amount of the added plasma increasing, the AC of TC and LDL-C markedly increased (especially from 1:2 to 1:3), while that of HDL-C decreased gradually, thus giving better LDL binding selectivity. This might be due to the fact that more plasma gave higher lipoprotein equilibrium concentration in the plasma, which was advantageous to competitive adsorption of LDL.
Table 2 Effect of plasma amount on the lipoprotein adsorption
Effect of Incubation Time on Adsorption of Lipoproteins
illustrates the dynamic sorption of the lipoproteins onto the copolymer beads. All the adsorption of TC, LDL-C and HDL-C reached equilibrium within 2.5 h. It is noticeable that, before approaching the constant values, the AP of TC and LDL-C increased gradually, while that of HDL-C reached a maximum. This result coincided with our previous observations on Try-modified chitosan beads [Citation[12]], and can be explained as follows. HDL particles, which are much smaller than LDL (3.5–9 nm vs. 20–25 nm), rapidly diffused into the inside of adsorbent and occupied the adsorptive sites prior to LDL at the initial stage of adsorption. However, with the adsorption proceeding, they were desorbed gradually due to the stronger competitive adsorption of LDL until the equilibrium was reached.
Adsorption of TP
TP, including albumin and globulin, which are important beneficial components in human plasma, should be saved as far as possible when any extracorporeal therapy procedure is performed. The resulted adsorbent exhibited little effect on the TP level in plasma, and the adsorption of TP was less than 10%, which met the clinical requirements.
Comparison with Related Adsorbents
As listed in , we here compare the in vitro lipoprotein sorption performance of the PAA-grafted cellulose beads with that of some related LDL adsorbents. Although there were some differences in the adsorption conditions, such as initial lipoprotein concentrations in the plasma and the amount ratio of plasma to adsorbent, it can be seen that the modified cellulose beads in this work represented quite good lipoprotein binding performance, except that the proportion of adsorbed HDL was slightly higher.
Table 3 Comparison of lipoprotein sorption performance with some related LDL adsorbents
Compared with the PAA-grafted chitosan beads reported previously [Citation[18]], the modified cellulose beads in this study gave much higher LDL and TC binding capacities, accounting for the fact that a half amount of plasma was used in those adsorption tests while the resulted reduction extent of lipoprotein levels was comparative. Different from the case with the chitosan beads, suitably low PAA grafting percentage onto cellulose beads could be readily controlled, and this might contribute to the reason.
The PAA-grafted cellulose beads at least gave comparative adsorption performance while in comparison with the other two cellulose-based adsorbents, i.e. dextran sulfate immobilized cellulose beads (commercial Liposorber LA-40) [Citation[28]] and a new amphiphilic cellulose adsorbent bearing sulfate and cholesterol [Citation[10]]. The Liposorber LA-40 is very expensive since dextran sulfate is not readily obtained. The preparation of the amphiphilic adsorbent consisting of multi-step rigorously nonaqueous reactions was very complicated, hence leading to high production costs. As described above, however, it is quite easy to perform single-step graft copolymerization of inexpensive AA onto cellulose beads.
CONCLUSION
In the present study, a new adsorbent for selective LDL removal was prepared simply by graft copolymerization of AA onto cellulose beads. Through adjusting the reaction conditions, the resulted copolymer beads bearing a properly low amount of PAA exhibited relatively good LDL binding performance. The uptake of HDL is still expected to be further reduced by optimizing the grafting variables. It appears that this kind of copolymer is worthy of being developed as an alternative LDL adsorbent.
The project was supported by the National Key Project of Fundamental Research and Advance of P. R. China (Grant No. G1999064707).
REFERENCES
- Pekkanen, J., Linn, S., Heiss, G., Suchindran, C., Leon, A., Rifkind, B., Tyroler, H. (1990). Ten year mortality from cardiovascular disease in relation to cholesterol level among men with and without preexisting cardiovascular disease. N. Engl. J. Med. 322: 1700–1707. [INFOTRIEVE], [CSA]
- Thompson, G.R., Lowenthal, R., Myant, R. (1975). Plasma exchange in the management of homozygous familial hypercholesterolemia. Lancet 1: 1208–1211. [INFOTRIEVE], [CSA], [CROSSREF]
- Thompson, G.R. (2003). LDL apheresis. Atherosclerosis 67: 1–13. [CSA], [CROSSREF]
- Stoffel, W., Borberg, H., Grewe, V. (1981). Application of specific extracorporeal removal of low density lipoprotein in familial hypercholesterolemia. Lancet 2: 1005–1007. [INFOTRIEVE], [CSA], [CROSSREF]
- Yokoyama, S., Hayashi, R., Satani, M., Yamamoto, A. (1985). Selective removal of low density lipoprotein by plamapheresis in familial hypercholesterolemia. Atherosclerosis 5: 13–22. [CSA]
- Bosch, T., Schmidt, B., Blumenstein, M., Gurland, H.J. (1993). Lipid apheresis by hemoperfusion: In vitro efficacy and ex vivo biocompatibility of a new low-density lipoprotein adsorber compatible with human whole blood. Artif. Organs 17: 640–642. [INFOTRIEVE], [CSA]
- Kobayashi, A., Nakatani, M., Furuyoshi, S., Tani, N. (2002). In vitro evaluation of dextran sulfate cellulose beads for whole blood infusion low-density lipoprotein-homoperfusion. Ther. Apher. 6(5): 365–371. [INFOTRIEVE], [CSA], [CROSSREF]
- Murabayashi, S., Nishide, T., Mitamura, Y. (2002). In vitro evaluation of newly developed adsorbent for selective removal of glycosylated low-density lipoprotein. Ther. Apher. 6(6): 425–430. [INFOTRIEVE], [CSA], [CROSSREF]
- Xu, J.K., He, B.L. (2002). Preparation of a new adsorbent for binding low density lipoprotein. Chem. J. Chin. Univ. 23(7): 1421–1424. [CSA]
- Wang, S.Q., Yu, Y.T., Cui, T., Cheng, Y. (2002). Cellulose amphiphilic adsorbent for the removal of low density lipoprotein. Artif. Cells Blood Substit. Immob. Biotechnol. 30(4): 285–292. [CSA], [CROSSREF]
- Wang, S.Q., Yu, Y.T., Cui, T., Cheng, Y. (2003). A novel amphiphilic adsorbent for the removal of low-density lipoprotein. Biomaterials 24: 2799–2802. [INFOTRIEVE], [CSA], [CROSSREF]
- Cao, N.N., Yu, Y.T., Wang, M.Y., Chen, C.Z. (2002). In vitro study of a novel low-density lipoprotein adsorbent. Art. Cells Blood Substit. Immob. Biotechnol. 30(1): 53–61. [CSA], [CROSSREF]
- Fu, G.Q., Shi, K.Y., Yuan, Z., He, B.L., Liu, B., Shen, B., Wang, Q.S. (2003). Preparation of tryptophan modified chitosan beads and their adsorption of low density lipoprotein. Chin. Sci. Bull. 48(21): 2303–2307. [CSA], [CROSSREF]
- Fu, G.Q., Yu, H.F., Yuan, Z., Liu, B., Shen, B., He, B.L. (2004). Chitosan adsorbents carrying amino acids for selective removal of low density lipoprotein. Artif. Cells Blood Substit. Biotechnol. 32(2): 303–313. [CSA], [CROSSREF]
- Yavuz, H., Denizli, A. (2005). Immunoadsorption of cholesterol on Protein A oriented beads. Macromol. Biosci. 5: 39–48. [INFOTRIEVE], [CSA], [CROSSREF]
- Li, H.T., Zhang, Y.M., Chen, X.F., Shi, K.Y., Yuan, Z., Liu, B., Shen, B., He, B.L. (2004). Synthesis and adsorption aspect of cross-linked PVA-based blood compatible adsorbents for LDL apheresis. React. Funct. Polym. 58: 53–63. [CSA], [CROSSREF]
- Yavuz, H., Denizli, A. (2003). Immunoaffinity beads for selective removal of cholesterol from human plasma. J. Biomater. Sci. Polym. E 14(5): 395–409. [CSA], [CROSSREF]
- Fu, G.Q., Li, H.Y., Yu, H.F., Liu, L., Yuan, Z., He, B.L. (2006). Synthesis and lipoprotein sorption properties of porous chitosan beads grafted with poly(acrylic acid). React. Funct. Polym. 66: 239–246. [CSA], [CROSSREF]
- Fang, H., Wei, J., Yu, Y.T. (2004). In vivo studies of endotoxin removal by lysine–cellulose adsorbents. Biomaterials 25: 5433–5440. [INFOTRIEVE], [CSA], [CROSSREF]
- Gurdag, G., Yasar, M., Gurkaynak, M.A. (1997). Graft copolymerization of acrylic acid on cellulose: reaction kinetics of copolymerization. J. Appl. Polym. Sci. 66: 929–934. [CSA], [CROSSREF]
- Chen, H., Hsieh, Y.L. (2005). Enzyme immobilization on ultrafine cellulose fibers via poly(acrylic acid) electrolyte grafts. Biotech. Bioeng. 90: 405–413. [CSA], [CROSSREF]
- Gurdag, G., Guclu, U.G., Ozgumaus, S. (2001). Graft copolymerization of acrylic acid onto cellulose: Effects of pretreatments and cross-linking agent. J. Appl. Polym. Sci. 80: 2267–2272. [CSA], [CROSSREF]
- Lori'A-Bastarrachea, M.I., Carrillo-Escalante, H.J., Aguilar-Vega, M.J. (2002). Grafting of poly(acrylic acid) onto cellulosic microfibers and continuous cellulose filaments and characterization. J. Appl. Polym. Sci. 83: 386–393. [CSA], [CROSSREF]
- Choi, Y.J., Ahn, Y., Kang, M.S., Jun, H.K., Kim, I.S., Moon, S.H. (2004). Preparation and characterization of acrylic acid-treated bacterial cellulose cation-exchange membrane. J. Chem. Tech. Biotech. 79: 79–84. [CSA], [CROSSREF]
- Yoshida, T., Hattori, K., Sawada, Y., Choi, Y., Uryu, T. (1996). Graft copolymerization of methyl methacrylate onto curdlan. J. Polym. Chem. 34: 3053–3060. [CSA], [CROSSREF]
- Liu, M.H., Li, X., Liu, W.G., Zhang, X.S. (2001). Preparation of a new kind of spherical cellulose adsorbent. Fine Chem. 18(7): 401–404. [CSA]
- Du, J., Zhang, X.S., Chen, J. (1999). Study on a novel amphoteric bead cellulose ion-exchanger. Ion Exch. Adsor. 15(6): 561–566. [CSA]
- Lewinska, D., Rosinski, S., Piatkiewicz, D. (1994). A new pectin-based material for selective LDL-cholesterol removal. Artif. Organs 18: 217–222. [INFOTRIEVE], [CSA]