Abstract
New ammonium-selective membrane electrode based on poly(vinyl chloride) (PVC) membrane containing palmitic acid (a long-chain fatty acid) and nonactin as an ammonium ionophore for the determination of ammonim ions in the 10−7 − 10−1 mol/L concentration range was prepared and compared to those of the electrode prepared by using carboxylated PVC. Sebacate was used as a plasticiser for both of the ammonium sensor membranes. The analytical characteristics of the ammonium electrodes was investigated. The effect of pH, buffer concentration, temperature and stirring rate on the response to ammonium electrode was investigated. The linear working range and sensitivity of the electrodes were also determined. Ammonium electrodes give Nernstian response (52–58 mV/p[NH4+]) throughout the ammonium ion concentration range of 10−1 to 10−6M with detection limits of 10−6M ammonium ions. The ammonium-selective electrodes prepared by using the PVC membranes containing palmitic acid showed more effective performance than those of the carboxylated PVC. The ammonium ion sensor has potential application in the analysis of ammonium ions for biosensor construction.
INTRODUCTION
Potentiometric ion-selective membrane electrodes for the direct determination of discrete ions in biological, enviromental, and industrial samples have grown rapidly in recent years. Ammonium ion sensor is a useful tool in environmental pollution control [Citation[1], Citation[2]], food and clinical analyses [Citation[3-5]], and other industry applications [Citation[6]].
Over the last few years, the measurement of ammonium (NH4+) for different purposes has become increasingly important. Various detection methods and techniques have been used for the determination of ammonium ions. In particular, the methods based on the Berthelot reaction [Citation[7]] have been widely used. The reaction is highly selective for ammonia and the method is rapid and sensitive, but some important drawbacks related to the high temperature required (60°C), making it unsuitable for in situ application, and instability of the reagents used invalidate it for automatic monitoring purposes [Citation[8]].
Measurement of ammonium ions in biosensors has found an increasing application in the past 10 years because ammonium ions are a metabolic product in many enzymatic reactions. For example, ammonium and bicarbonate ions can be produced by the metabolism of urea with enzymatic reaction of urease. Therefore, urea can be detected by sensing its metabolic product, i.e. ammonium ions [Citation[9-11]]. Ammonium ion-selective electrodes are also used most frequently as potentiometric biosensors where enzymes or microbial cells are immobilized on the surface of the sensor [Citation[9], Citation[12], Citation[13]]. The detection of ammonium ions has been widely reported in the literature, including the spectrophotometric [Citation[9], Citation[12], Citation[14]], enzymatic [Citation[15]], potentiometric [Citation[12], Citation[14]] and amperometric [Citation[10], Citation[11]] methods.
In the literature, it is reported that the ammonium sensor based on plasticised carboxylated poly(vinylchloride) (PVC-COOH), the membrane of which contains nonactin as an ionophore, has the best performance [Citation[16]] and is capable of the direct determination of ammonium ion without any prior pre-treatment. Although the ammonium sensor based on plasticised poly(vinylchloride) (PVC) show a good response against ammonium ions, this type of electrode is not suitable for biosensor construction [Citation[12], Citation[17], Citation[18]]. For biosensor construction, the enzymes are immobilized with adsorption on the surface of poly(vinylchloride) membrane. Therefore, the biosensor response is good, but the life-time of the biosensor is very short.
In this present study, we decided to add a big molecule containing active ends (palmitic acid) into the PVC membrane composition while construction of the ammonium sensor. For this reason, we have developed a new ammonium ion sensor by adding palmitic acid into poly(vinyl chloride) membrane containing nonactin as an ammonium ionophore. The ammonium electrodes containing carboxylated poly(vinyl chloride) membrane matrix material were also prepared for comparing the sensitivities of these electrodes.
EXPERIMENTAL
Reagents and Apparatus
Nonactin, palmitic acid, bis-(2ethyl)hexyl sebacate (DOS), poly(vinylchloride) (PVC), carboxylated poly(vinylchloride) (PVC-COOH), tris(hydroxymethyl) aminomethane (Tris), chlorides of ammonium, potassium, sodium, lithium, and calcium were obtained from Fluka. All other chemicals used were analytical reagent grade. Standard solutions and buffer solutions were prepared with bidistilled water. Potential and pH measurements were carried out with an ORION 720A model pH-ionmeter.
Preparation of Ammonium-Selective Polymer Membrane Electrodes
The membrane compositions of ammonium-ion selective electrodes prepared using PVC containing palmitic acid and carboxylated PVC are shown in .
Table 1 The membrane compositions of ammonium-ion selective electrodes
Each membrane, according to the compositions in , was prepared by dissolving about 400 mg membrane components in 5 ml of tetrahydrofurane (THF). The solutions were poured on a glass plate inside a glass ring (42 mm diameter). After 24 hours drying at room temperature, the membrane was formed. Disks of 5 mm diameter were cut out and attached to the glass electrode body. The inner electrode solution was 10−2 M ammonium chloride (pH 7.0). Before measurement, all fresh electrodes were conditioned in 10−2 M ammonium chloride solution.
Measurement of the Electrodes' Response
Potential measurements were carried out by varying ammonium chloride concentration in steady-state condition. 5 mM of TRIS buffer was used as a working buffer solution (pH 7.0). The following electrochemical cell was formed with the proposed ammonium sensors using a double junction Ag/AgCl reference electrode by connecting an Orion ion meter similar to those reported by Heng and Hall [Citation[14]].
Measurements were made with the proposed ammonium sensor and reference electrode immersed to a depth of 1.5cm in ammonium chloride solution and the solution was stirred by a magnetic stirrer.
The pH values were determined using an Orion combination glass-pH electrode. All the experimental works were carried out at 20±1°C. The calibration curves were obtained by plotting the potential values of a series of standard ammonium chloride solution against the logarithm of NH4Cl concentration. The selectivity coefficients were evaluated by the separated solution method according to the IUPAC recommendation [Citation[19]].
RESULTS AND DISCUSSION
The slopes of the electrodes obtained from the membranes containing nonactin of 3% were better than those of 4%. The comparison between ammonium-ion selective electrodes prepared by using PVC containing palmitic acid and carboxylated PVC was made.
The Response of Ammonium-Selective Electrodes to Ammonium Ions
In order to understand sensitivity of the ammonium sensors to ammonium ions, a series of NH4Cl solutions were prepared and the pH values of these solutions were adjusted to pH 7.0 with trizma-base. mV measurements were made with each biosensor in a series of ammonium chloride solutions. Then calibration graphs were plotted and linear working ranges for ammonium ion and slopes of electrodes were determined from these curves. The linear range of these sensors extends from 1.0 × 10−6 to 1.0 × 10−1 M, showing an apparent Nernstian response (58,0 mV/p[NH4+]for electrode I) within this range.
It can be seen from and and that the sensitivies of all ammonium-selective electrodes are very good and in the concentration range of 1.0 × 10−1–1.0 × 10−6 M. Therefore we have decided that these ammonium electrodes can be used for determination of several analytes (urea, creatine, etc.) in biological fluids [Citation[9], Citation[20]]. We were able to carry out ammonium-selective urea and creatine biosensors for determining these substrates in human serum samples [Citation[9]].
Figure 1 Calibration curve for ammonium ion. Electrode I: with 3% nonactin prepared by using PVC membrane containing palmitic acid. Electrode II: with 3% nonactin prepared by using carboxylated PVC membrane.
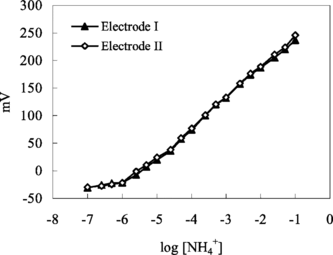
Figure 2 Calibration curve for ammonium sensor. Electrode IV: with 4% nonactin prepared by using PVC membrane containing palmitic acid. Electrode V: with 4% nonactin prepared by using carboxylated PVC membrane.
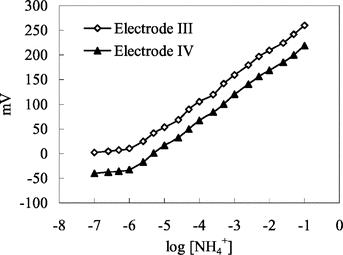
Table 2 Slopes and linear working ranges of the ammonium electrodes at 20 ± 1°C
It can be seen the calibration graphs of electrode I and electrode III from and that the sensitivity of electrode I prepared by using PVC containing palmitic acid is better than those of the others and the slope of this sensor was 58.0 ± 0.1 mV/p[NH4+]. All sensors were found to give a linear response against ammonium ions in the ammonium chloride concentration range of 1.0 × 10−1–1.0 × 10−6 M. The slope of electrode II prepared by using carboxylated PVC was 54.7 ± 0.1 mV/p[NH4+].
The slopes of the ammonium electrodes prepared with membranes containing 3% nonactin were bigger than those of the ammonium electrodes prepared with membranes containing 4% nonactin. Therefore we can conclude that using the membranes containing 3% nonactin was more suitable. Electrode I showed the best results among other ammonium sensors.
Effect of Buffer Concentration
The responses of the ammonium-selective sensors were measured at six different buffer concentrations varying from 5 mM to 30 mM. The best results for all ammonium sensors were obtained at 5 mM TRIS buffer (). Buffer concentrations lower than 5 mM were not investigated due to poor buffering capacity of the solution. Therefore, optimum buffer concentration was accepted to be 5 mM.
Figure 3 The effect of buffer concentration on ammonium-selective sensors. I and II: electrodes with 3% and 4% nonactin prepared by using PVC containing palmitic acid; III and IV: electrodes with 3% and 4% nonactin prepared by using carboxylated PVC, respectively.
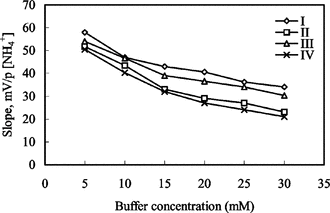
It can be seen that the high slope was obtained from 5 mM buffer concentration. The slopes of ammonium electrodes I and III prepared with membranes containing 3% nonactin were bigger than those of ammonium electrodes II and IV containing 4% nonactin. Electrode I showed best results among other ammonium sensors. Therefore we can conclude that using the membranes containing 3% nonactin was more suitable. However, because the slopes obtained from four ammonium electrode are high enough to use analytical purphases and are near Nernstian response (59 mV), it can be said that four ammonium electrodes can be used for determination of ammonium ions at 5 mM buffer concentration.
Effect of Buffer pH
Effect of buffer pH on the analytical signal of the ammonium sensors was investigated by measurements in 5 mM TRIS buffer at six different pH in the range of 6.0–8.0. It is shown that the slopes and sensitivities of ammonium sensors gradually increase until pH 7.0 and decrease after pH 7.0 (). Above pH 7.0, urease is denaturated due to deprotonation at active site. At pH less than 7.0, urease can also be denaturated and hence reduce enzyme activity [Citation[21]]. The response was also diminished with increase in buffer pH, which is a common property of the potentiometric systems [Citation[22]]. pH 7.0 was therefore used for all other measurements.
Figure 4 The effect of pH on ammonium sensors. I and II: electrodes with 3% and 4% nonactin prepared by using PVC containing palmitic acid; III and IV: electrodes with 3% and 4% nonactin prepared by using carboxylated PVC, respectively.
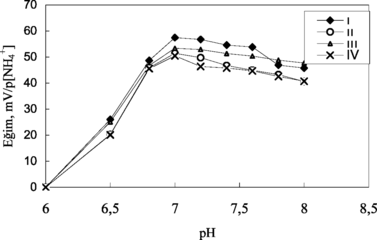
It is seen from that the potential responses of ammonium electrodes prepared by using four different procedures were linear and that these biosensors can be used in biosensor preparation for the determination of some analytes.
Effect of Temperature
Effect of temperature on analytical signal of all ammonium sensors was investigated by measurements for six different temperatures changing from 20°C to 45°C. As is seen from , the best suitable temperature for the ammonium sensors is 20°C–40°C and the slope of the ammonium electrodes decrease after 45°C because the membranes of the ammonium electrode were deformed with the increase in temperature. Therefore it can be said that the membranes prepared according to this procedure are not suitable to use after 45°C and it is thought that ammonium determination can be carried out at room temperature by using these sensors.
Selectivity Coefficients of Ammonium Electrodes
The prepared ammonium electrodes were tested in view of their sensitivity towards sodium, potassium, lithium and calcium ions (as chlorides, pH 7.0), and their selectivity coefficients were evaluated by the separated solution method [Citation[19]].
It can be seen from that these results are good correlation with literature data. Interference effects of Na+, K+, Li+ and Ca+2 ionson the response of theammonium electrodes, using poly(vinyl chloride) (PVC) membrane containing palmitic acid (a long-chain fatty acid) and nonactin as an ammonium ionophore, were low, but K+ ions showed the most interference among these cations. It can be said that the ammonium electrodes using poly(vinyl chloride) (PVC) membrane containing palmitic acid and nonactin can also be succesfully used in biological fluids. These ions also showed low interference effects against the electrodes prepared by using carbaxylated PVC (Electrodes III and IV).
Table 3 Selectivity coefficients of ammonium electrodes
Effect of Stirring Rate
To study the effect of stirring rate on the responses of ammonium sensors, the measurements were done in 5 mM TRIS buffer (pH 7.0) at three different stirring rates (100, 250, and 750 rpm) after reaching stable potential. The effect of stirring rate is not important on the response of ammonium electrodes.
Response Time
The response time of the ammonium sensors was determined by recording the time needed to reach a stable potential value after the sensor and the reference electrode were immersed in calibration solutions. This response time changed between 30–60 sec. At lower ammonium concentrations the responses of ammonium electrodes were bigger (40–60 sec.) than those of higher ammonium concentrations (30–40 sec.). In conclusion, the response time of the sensors that we prepared is better than most similar sensors reported in the literature.
Lifetime of the Ammonium Sensors
The lifetimes of all ammonium sensors were determined by reading the potential values of the calibration solutions and by plotting the calibration curves for a period of two months. The linear working ranges and slopes of ammonium sensors didn't show any important changing during a period of two months, but the slopes of the ammonium sensors were decreased after two months (). It can be concluded that the lifetime of the ammonium electrodes containing palmitic acid is longer than those of carboxylated PVC.
Reproducibility of the Ammonium Sensors
The ammonium sensors were tested for reproducibility by plotting the calibration curves for five times within one day with the same sensor and the slopes of the sensors were determined. The relative standard deviation of the slopes was found to be less than 0.5–1.0% for all ammonium electrodes.
CONCLUSION
A new type of ammonium electrode based on PVC membrane containing palmitic acid showed very good analytical parameters: high sensitivity, dinamic stability over 2 months with less decrease of sensitivity, response time 1–2 minutes. We proposed this ammonium electrode for several ammonium ion-selective biosensor constructions.
We gratefully acknowledge the financial support of T.R. Prime Ministry State Planning Organization (Project No: 98-K-120830).
REFERENCES
- Heng, L.Y., Alva, S., Ahmad, M. (2004). Sensors and Actuators B. 98: 160–165. [CSA]
- Alexander, P.W., Dikitrakopoloulos, T., Hibbert, D.B. (1997). Electroanalysis 9: 1331–1336. [CSA], [CROSSREF]
- Magalhaes, J.M.C.S., Machado, A.A.S.C. (2002). Analyst 127: 1069–1075. [INFOTRIEVE], [CSA], [CROSSREF]
- Palleschi, G., Mascini, M., Martinez-Fabregas, E., Alegret, S. (1988). Anal. Lett. 21: 1115–1129. [CSA]
- Lima, J.F.C.C., Delerue-Matos, C., Cristina, C.V.M. (1999). Anal. Chim. Acta. 385: 437–441. [CSA], [CROSSREF]
- Suzuki, T., Yasuda, T., Yamane, T., Shimizu, S. (1986). J. Ferment. Technol. 64: 63–70. [CSA], [CROSSREF]
- Searle, P.L. (1984). Analyst. 109. [CSA]
- Walcarius, A., Vromman, V., Bessiere, J. (1999). Sensors and Actuators B. 56: 136–143. [CSA], [CROSSREF]
- Karakuş, E., Pekyardιmcι, Ş., Kιlιç, E. (2005). Artif. Cells Blood Subst. Biochem. 33: 329–341. [CSA]
- Luo, Y.C., Do, J.S. (2004). Biosens. Bioelectron. 20: 15–23. [INFOTRIEVE], [CSA], [CROSSREF]
- Cho, W.J., Huang, H.J. (1998). Anal. Chem. 70: 3946–3951. [CSA], [CROSSREF]
- Ikeda, M., Hichaya, H., Ito, S., Asanao, Y., Imato, T. (1998). Biosens. Bioelectron. 13: 531–537. [CSA], [CROSSREF]
- Okada, T., Karube, I., Suzuki, S. (1982). Anal. Chim. Acta. 135: 159–163. [CSA], [CROSSREF]
- Heng, L.Y., Hall, E.A.H. (2000). Anal. Chem. 72: 42–51. [INFOTRIEVE], [CSA], [CROSSREF]
- Bertocchi, P., Compagnone, D., Palleschi, G. (1996). Biosen. Bioelectron. 11: 1–10. [CSA], [CROSSREF]
- Koncki, R., Walcerz, I., Ruckruh, F., Glab, S. (1996). Anal. Chim. Acta. 333: 215–222. [CSA], [CROSSREF]
- Fraticelli, Y.M., Meyerhoff, M.E. (1981). 53: 992–997. [CSA]
- Eggenstein, C., Borchardt, M., Diekmann, C., Gründig, B., Dumschat, C., Cammann, K., Knoll, M., Spener, F. (1999). Biosens. Bioelectron. 14: 33–41. [INFOTRIEVE], [CSA], [CROSSREF]
- Umezawa, Y., Bühlmann, P., Umezawa, K., Tohda, K., Amemiya, S. (2000). Pure Appl. Chem. 72(10): 1851–2082. [CSA]
- Koncki, R., Kopczewska, E., Glab, S. (1994). Anal. Lett. 27(3): 475–486. [CSA]
- Adeloju, S.B., Shaw, S.J., Wallace, G.G. (1996). Anal. Chim. Acta. 323: 107–113. [CSA], [CROSSREF]
- Ru-Qin, Y., Zong-Rang, Z., Guo-Li, S. (2000). Sensors Actuator. 65: 150–153. [CSA], [CROSSREF]