Abstract
To investigate the apoptosis effect of SARS coronavirus nucleocapsid protein on cultured cell lines and to explore the possible pathway of apoptosis. pCDNA3.1(-)/his-myc vector containing the SARS coronavirus nucleocapsid gene (N), matric gene (M), spike gene (S) were transfected into COS-1, Huh-7 and HepG2 cells. Apoptosis induced by SARS coronavirus N protein under starvation of serum of COS-1 cells was monitored by Annexin V and electron microscopy assays. Intracellular reactive oxygen species (ROS) and mitochondrial membrane potential (ΔΨm) were determined by flow cytometric assay. Cytochrome C, cleaved caspase (cysteine aspartic acid protease)-3, 9, and poly (ADP-ribose) polymerase (PARP) were detected by Western blot. After removal of serum in COS-1 cells, we observed the loss of ΔΨm, the increase of ROS and cytochrome C release into cytosol and subsequent activation of caspase-3 and PARP cleavage. The pan-caspase inhibitor z-VAD-fmk can block the activation of caspase 3, 9 and PARP cleavage. In conclusion, SARS coronavirus N protein can induce apoptosis of COS-1 cells by activating mitochondrial pathway. SARS coronavirus M, S protein can not induce apoptosis in COS-1, HepG2 and Huh-7 and SARS coronavirus N protein can not induce apoptosis in HepG2 and Huh-7 by methods used in this study.
INTRODUCTION
A great global threat was brought about by the outbreak of Severe Acute Respiratory Syndrome (SARS), which was caused by a novel coronavirus designated as SARS coronavirus. SARS is a system disease that injures many organs, such as the lungs, liver, and immune organs. Respiratory distress and decreased immune function are the main death causes of SARS patients [Citation[1-3]]. Apoptosis was found in many organs of SARS patients. The pathological changes in these organs may be caused directly by the cytopathic effect by the SARS-CoV or indirectly as a result of system responses to respiratory failure or as the harmful immune response induced by viral infection. In cell lines, we can study the cytopathic effect caused by SARS-CoV. It was reported that SARS-CoV induces apoptosis on Vero E6 cells [Citation[4]], but the relevant mechanisms have not yet been fully elucidated. SARS-CoV is an enveloped virus containing a single stranded, positive-sense RNA genome of about 29 kb nucleotides in length that encodes replicase, four viral structural proteins including the spike (S) glycoprotein, the matrix (M) protein, the small envelope (E) protein and the nucleocapsid (N) protein, and several non-structure proteins [Citation[5]]. It has been known that some virus proteins play important roles in virulence and pathogenesis. Many molecular aspects of SARS-CoV N protein have been reported, including self-dimerization [Citation[6]], RNA-binding capabilities [Citation[7]] and its abilities to activate signal transduction pathways [Citation[8]]. It found SARS-CoV N protein can induce actin apoptosis in COS-1 cells in the absence of growth factors detected by TUNEL (Terminal deoxynucleotidyl transferase-mediated dUTP nick-end labeling) assay and actived caspase 3 and 7 [Citation[9]]. We confirmed that N protein can induce apoptosis in COS-1 in several other assays such as Anneixn V and electron microscopy. We further studied which upstream pathway contributes to active caspase-3 and 7. As we all know, caspases are a family of cysteine-dependent aspartate-directed proteases that exist in cells as inactive zymogens and are triggered to cleave a set of proteins at specific sites containing aspartic acid to cause cell death. Initiator caspases (e.g., caspase 8 and caspase 9) are activated to trigger the full caspase cascade, and caspase 3 is activated as an effector to induce apoptosis [Citation[10-14]]. Caspase-9 activation was observed in SARS coronavirus N transfected COS-1 cells after serum withdrawal. Elevated level of intracellular reactive oxygen species (ROS), the disruption of mitochondrial membrane potential (MMP), and release of cytochrome C from mitochondria into the cytosol were also observed. Our results demonstrated that SARS N protein induces apoptosis by mitochondrial pathway in COS-1 cells. And SARS N protein cannot induce apoptosis in hepatoma cell lines Huh-7 and HepG2. As to SARS M, S protein, it can not induce apoptosis in COS-1, Huh-7, HepG2 by methods used in this study.
MATERIALS AND METHODS
Construction of Recombinant Vectors
The vectors containing the SARS coronavirus nucleocapsid gene (N) (28105 ∼ 29373nt), matric gene (M) (26398 ∼ 27063nt), spike gene (S) (21492 ∼ 25259nt) (Gene Bank Accession No. AY274119) were cloned, sequenced and kindly provided from Professor Chen Jian Guo of Peking University Life Science Center. Primers for the amplification of N, M, S complete coding regions incorporation 5′BamHI and 3′KpnI restriction enzyme sites were 5′-ATCGGGATCCGACCATGTCTGATAATGGACC CCAATC-3′ and 5′-ATCAGGTACCTTATGCCTGAGTTGAATCAGCAG-3′, 5′-ATCGGGATCCGACCAT GGC AGACAACGGTACTATTAC-3′ and 5′-ATCAGGACCTTACTGTACTAGCAAA GCAATATTG-3′, 5′-ATCGGGATCCGACCATGTTTATTTTCTTATTATTTC-3' and 5′-ATCAGGTACCTTATGTGTAATGTAATTTGACACC-3′, respectively. The amplified product was purified by MiniElute PCR Purification kit (Qiagen, Valencia, CA), and then subcloned into pCDNA3.1(-)/His-MycB (Invitrogen, Carlsbad, CA). The sequences of N, M, S constructs were verified by DNA sequencing.
Mammalian Cell Culture and Cell Transfection
The COS-1 and Huh-7 were cultured in Dulbecco's modified Eagle's medium, HepG2 cell was cultured in minimum essential medium, supplemented with 10% heat-inactived fetal bovine serum and 100 units/ml penicillin, 100 mg/ml streptomycin, pH 7.4). All cells were cultured in humidified air with 5% CO2 incubator at 37°C. As many as 1 × 10Citation6 cells were transfected by lipofactamine 2000 (Invitrogen, Carlsbad, CA) according to the manufacturer's protocol. Briefly, the mixture of 8 micrograms of DNA and 16 µl(1:2) lipofectamine 2000 (Invitrogen) were added onto cells in 6 cm plates and cells were incubated in a 37°C, 5% CO2 incubator before harvested. This transfection protocol routinely yielded approximately 40 ∼ 50% transfection efficiency, as routinely monitored by co-transfecting cells with pEGFP-C3 plasmid. Mock transfected cells were transfected with the empty vector of pCDNA 3.1(-)/His-Myc B. After transfection, the cells were normally cultured for 24 hours, cultured under serum withdrawal for 24 ∼ 36 hours, and then harvested. The general caspase inhibitor, z-Val-Ala-Asp(OMe)-fluoromethylketone (z-VAD-fmk) (100 uM) (R&D Systems), was added to the culture media of transfected cells 24 hours before harvesting.
Western Blot
Cells were washed twice with ice-cold phosphate-buffered saline (PBS) and lysed in lysis buffer containing 50 mM Tris-HCl (pH 8.0), 150 mM NaCl, 1% Triton X-100, 1 µg/ml Aprotinin, 1 µg/ml Leupeptin and 1 µg/ml Pepstatin, 1 mM PMSF. After being incubated on ice for 20 minutes, the lysates were centrifuged at 10,000 g for 5 min at 4°C. The supernatant was transferred to a fresh tube. The protein concentration was measured using the BCA protein assay reagent (Pierce, USA) and stored at − 80°C for further analysis. For immunoblot analysis, protein samples were subjected to sodium dodecyl sulfate-polyacrylamide gel electrophoresis (SDS-PAGE) separation and transferred to nitrocellulose membranes (American Osmonies). Membranes were blocked in Tris-buffered saline containing 0.05% Tween-20 (TBS-T) containing 5% non-fat milk for 2 hours and incubated overnight at 4°C using the following primary antibodies: polyantibody to SARS-N (nucleocapsid) protein (1:200, Imgenex, San Diego, CA), polyclonal antibody to SARS-M (matix) protein (1 ug/ml, Imgenex), Monoclonal Antibody to SARS-S (spike) protein (2 ug/ml, Imgenex), polyclonal antibody to caspase-3 (Cell Signal Technology, Beverly, MA), polyclonal antibody to caspase-9 (1:1000, BD Biosciences, Palo Alto, CA), polyclonal antibody to cytochrome C (1:1000, Cell Signal Technology), monoclonal antibody to endogenous poly (ADP-ribose) polymerase (PARP) (1:1000, BD Biosciences), monoclonal antibody to actin (1:400, Santa Cruz Biotechonlogy, Santa Cruz Inc., USA), Blots were washed (three times for 10 minutes each) with TBS-T and incubated for 1 hour with the following corresponding secondary antibody prepared in TBST/5% non-fat milk: horseradish peroxidase-conjugated anti-rabbit immunoglobulin G (1:1000; Santa Cruz Biotechonlogy) and horseradish peroxidase-conjugated anti-mouse immunoglobulin G (1:2000; Santa Cruz Biotechonlogy). Signals were visualized by the enhanced chemiluminescence detection system (Amersham Pharmacia Biotechnology, UK) according to the manufacturer's instructions.
Cell Death Triggered by N is Accompanied by Exposure of PS
To characterize N-induced cell death further, we detected PS surface exposure by FITC/annexin-V staining (purchased from BD Biosciences) of cells 24–36 hours after transfection. Plasma membrane integrity was simultaneously assessed by PI dye exclusion using two-color fluorescence-activated cell sorting (FACS) analysis. Simultaneously, the integrity of the membrane in these cells was maintained, based on the finding that the PI-positive population was similar to that observed in mock-transfected cells.
Electron Microscopy
For transmission electron microscopy, cells were initially fixed in 0.1 M sodium phosphate buffer containing 2.5% glutaraldehyde, pH 7.4. Next, cells were fixed in 0.1 M sodium phosphate buffer containing 1% OsO4, pH 7.2. Cells were embedded into Ultracut (Leica, Germany) and sliced into 60 nm sections. Ultrathin sections were stained with uranyl acetate and lead citrate, and examined with a JEM-1230 transmission electron microscope (JEOL, Japan).
Caspase Cleavage Assay
Activation of caspase-3 and PARP cleavage was detected by Western blot in 18% or 10% SDS-PAGE gel. Caspase-3 is responsible for cellular dysfunction and structural destruction apoptosis [Citation[13]]. Caspase-3 activation yields a 17 kDa product and the cleavage of PARP by activated caspase-3 yields a 85 KDa product. Caspase-9 activity was also measured by decrease of procaspase-9.
Measurement of Intracellular Reactive Oxygen Species (ROS) and Mitochondria Membrane Potential (ΔΨm)
ROS levels were determined by measuring the fluorescence of the oxidized form of the probe 2,7-dichlorofluorescein diacetate (DCF-DA, Molecular Probes Inc. Eugene, OR, USA) [Citation[15]]. Changes of ΔΨm have been considered to be an indicator of mitochondrial damage. 3′3′-dihexyloxacarbocyanine (DioC6), Molecular probes, (USA) was used to evaluate changes in ΔΨm. Cell suspensions (5 × 10Citation5) were incubated for 15 minutes at 37°C in 1 ml PBS containing 2.5 µM DCF-DA or 2.5 uM DioC6. Cellular fluorescence intensity was subsequently analyzed with a FACS Calibur flow cytometer. Results were expressed as the mean fluorescence intensities of all cells representing ROS production or mitochondrial membrane potential estimated by the DioC6 uptake. For each analysis, 20,000 events were recorded. The data were analyzed using CellQuestTM software (Becton Dickinson, FACScan).
Detection of Cytochrome C Release
The release of mitochondrial cytochrome C was determined by Western blot. Mitochondria were freshly isolated from COS-1 cells by differential centrifugation. At the end of treatments, cells were collected with trypsin, washed with PBS, and then resuspended in ice-cold homogenizing buffer (250 mM sucrose, 20 mM Hepes-KOH (pH 7.5), 10 mM KCl, 1.5 mM MgCl, 1 mM EDTA, 1 mM ethyleneglycol-bis-(L-aminoethylether)- N,N,NP,NP-tetraacetic acid (EGTA), 1 mM DTT, 1 mM PMSF, 1 mg/ml aprotinin and 1 mg/ml leupeptin). After 30 min incubation on ice, cells were homogenized with a glass dounce homogenizer (40 strokes). The homogenate was subjected to a series of centrifugation (1,000 g for 10 min, then 10,000 g for 20 min, and finally 100,000 g for 60 min, 4°C) for collection of supernatant. After the last centrifugation, the supernatant was a cytosolic fraction and the pellet was a mitochondrial fraction. Protein was loaded into 18% SDS-PAGE gel for electrophoresis.
Statistical Analysis
Data are given either as the mean±S.D. from at least three sets of independent experiments, or the numbers of experiments indicated in the legends as one representative of three experiments giving similar results. Student's t-test was used to assess the significance. P < 0.05 was considered to be statistically significant. *P < 0.05, **P < 0.01
RESULTS
Confirmation of SARS N, M, S Protein in Western Blot
To verify the expression of the SARS coronavirus N, M, S proteins in three cell lines, transiently transfected cells were lysed and analyzed by western blot ().
Figure 1 Detection of SARS coronavirus M, S, N protein in COS-1, Huh-7, HepG2 by Western blot analysis. The results are representative of at least three independent experiments. M, N protein is detected in 15% SDS-PAGE gel, S protein is detected in 10% SDS-PAGE gel. In mock (pCDNA 3.1-) transfected cells, no land can be detected in the corresponding site.
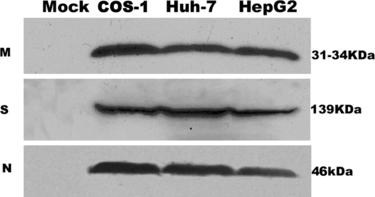
Death Triggered by SARS N Protein is Accompanied by Exposure of Phosphatidylserine Externalization (PS)
A biochemical hallmark of apoptotic cell death is the translocation of PS from the cytoplasmic surface of the cell membrane to the external cell surface [Citation[16]]. Exposure of PS at the surface of apoptotic cells is easily determined by flow cytometry using fluorescence-labeled annexin V, which specifically binds PS [Citation[17]]. To characterize SARS N protein induced cell death further, we detected PS surface exposure by FITC/annexin-V staining of cells under 24 hours serum withdrawal in three cell lines. Plasma membrane integrity was simultaneously assessed by PI dye exclusion using two-color fluorescence-activated cell sorting (FACS) analysis. In SARS coronavirus N plasmid transfected cells, the proportion of annexin-V positive/PI-negative cells was evidently increased verus mock transfected (transfected with empty vector alone) in COS-1 cells, P < 0.01. Simultaneously, the integrity of the membrane in these cells was maintained, based on the finding that the PI-positive population was similar to that observed in mock transfected cells. In SARS coronavirus N transfected HepG2 and Huh-7 cells, the proportion of annexin-V positive/PI-negative cells was not evidently increased () (P > 0.05) and under prolonged serum withdrawal of 36 hours, the apoptosis rate still has no evidently increased (data not shown). In SARS coronavirus M, S transfected three cell lines, the proportion of annexin-V positive/PI-negative cells was insignificantly increased even for 36 hours serum withdrawal (data not shown).
Figure 2 Annexin V detection of apoptosis induced by M, S and N protein of SARS coronavirus in COS-1, HepG2, Huh-7 cells. Cells were analyzed by flow cytometry with FITC-labeled annexin V and propidium iodide under 24 hours serum withdrawal. (A) Panels are transfected by mock M, S and N of SARS coronavirus from left to right in COS-1, HepG2, Huh-7 cells. In each panel (bottom left) viable cells (PI −, annexin-V/FITC −). (Bottom right) Early apoptotic cells (PI −, annexin-V/FITC +). (Top right) Non-viable, late apoptotic/necrotic cells (PI +, annexin-V/FITC +). (Top left) Necrotic cells(PI +). Numbers in the quadrants indicate the proportions of cells in the corresponding areas. Data from one representative experiment are presented. Experiments were performed in triplicate, with similar results. (B) Data are means±S.D. of triplicates from representative experiments. In COS-1 cells, apoptosis induced by SARS coronavirus N protein is 28.75%±1.93, **p < 0.01 vs. mock transfected.
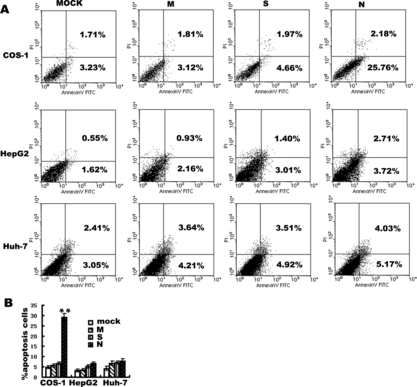
Ultrastructural Electron Microscope Evidence
At 24 hours serum withdrawal, ultrastructural analysis revealed the condensation of chromatin in SARS coronavirus N transfected COS-1 cells (), while in mock transfected COS-1 cells, the condensation of the chromatin was not evident in the nucleus. Under the same conditions, ultrastructural analysis revealed no obvious change in the nucleus induced by SARS coronavirus N protein in HepG2 and Huh-7 cells. As to SARS coronavirus M, S transfected HepG2 and Huh-7, there was not evident condensation of chromatin (data not shown).
Figure 3 Electron microscopy (EM) of SARS N transfected three cell lines. a1: Mock transfected COS-1 cell with a normal nucleus, well-preserved cytoplasm, containing well-preserved mitochondria. a2: SARS coronavirus N transfected COS-1 cell shows large dark mass of electron dense material in the nucleus, which clumped into a uniform sphere with a degenerated cytoplasm, comparable to that of mock transfected cells. b1: mock transfected HepG2. b2: N transfected HepG2.c1: mock transfected Huh-7 .c2:N transfected Huh-7. There is no obvious change in N transfected cells HepG2 and Huh-7 cells. Magnification: a1, b1, b2, c1,c2 (5000 ×), a2 (6700 ×).
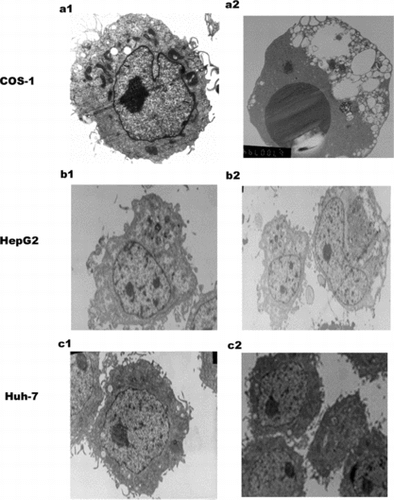
SARS-N-Induced Cell Death is Caspase-Dependent
To determine whether PS externalization is related to caspase-3-mediated proteolysis, we analyzed the activation of effector caspases, such as caspase-3, which is responsible for the proteolytic cleavage of a diverse range of structural and regulatory proteins in apoptosis [Citation[12]]. To confirm the activation of caspase-3, Western blots were performed in SARS coronavirus N and mock transfected cells approximately 24 hours serum withdrawal. As a result, we can detect activated caspase-3 and PARP cleavage in lysates of SARS coronavirus N protein overexpressing cells but not or a little in those of mock cells (). The pan-caspase inhibitor, z-Val-Ala-Asp(OMe)-fluoromethylketone (z-VAD-fmk) 100 uM, effectively inhibited this caspase-3 activity and PARP cleavage.
Figure 4 Western blotting analysis of activation of caspase-3 and cleavage of PARP in COS-1. Cells were mock or SARS coronavirus N transfected without or with z-VAD-fmk 100 uM. Caspase-3 and PARP were analyzed by separation of total proteins on 18% and 10% SDS-polyacrylamide gels, respectively, for three times.
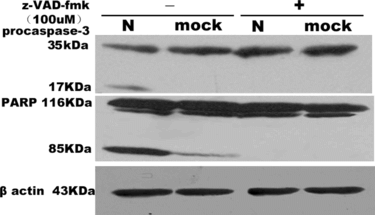
Monitoring Generation of Reactive Oxygen Species (ROS) and Disruption of Mitochondria Membrane Potential (ΔΨm)
An increasing amount of evidence has shown that mitochondria play central roles in the regulation of apoptotic cell death [Citation[10], Citation[11]]. To address the possibility that the SARS coronavirus N protein induced apoptosis could be related to contributions from the mitochondrial pathway, COS-1 cells withdrew serum for the indicated time periods after 24 hours' transfection. As early indicators of mitochondrial impairment, ROS production and ΔΨm were examined using the specific fluorescence probes, DiOC6 and DCF-DA, which is oxidized to fluorescence dye DCF in cells. As indicated in A, there is a moderate right shift of the DCF fluorescence in SARS coronavirus N transfected group comparing mock transfected group, indicating the increase in ROS generation. There is also a moderate left shift of the DiOC6 fluorescence curves in SARS coronavirus N transfected group comparing mock transfected group, indicating the decrease in ΔΨm (B). This is indicative that SARS coronavirus N protein induced mitochondrial dysfunction. The change of ROS and ΔΨm were gradually significant in time course. Under 24 h serum withdrawal, the increase of ROS was P < 0.01 (**) and decrease of ΔΨm was P < 0.05 (*) versus mock transfected COS-1.
Figure 5 Flow cytometry analysis of the effect of SARS coronavirus N protein on production of ROS (A) and decrease of ΔΨm (B) in COS-1 cells. Transfected cells were subjected to redox-sensitive fluorescent dye DCF-DA (A) and ΔΨm -sensitive fluorescent dye DiOC6 (B). Fluorescence related to intracellular ROS production and loss of ΔΨm were both measured in the fluorescence signal in the FL1 channel. Transfected cells were subjected to 12 hours, 18 hours, 24 hours serum withdrawal. The mean fluorescence intensity of cells with reduced DiOC6 or increased DCF staining is shown. In the left three panels of A and B, every experiment performed in triplicate with similar results and data of the panels are presented from a representative experiment. The right panels are the statistic results, which are means±S.D. from at least three experiments, *represented P < 0.05, **represented P < 0.01 compared to mock transfected cells. A.U. means Arbitrary Units.
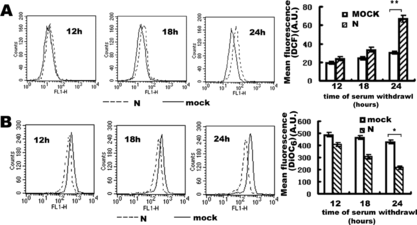
Release of Mitochondrial Cytochrome C and Activation of Caspase-9
Next, the effect of SARS coronavirus N protein on the cytochrome C release from mitochondria into the cytosol was demonstrated in COS-1 cells. In the meantime, we observed the activation of caspase-9, which is expressed as the decrease of procaspase-9. With time increased, the release of cytochrome C and caspase-9 activation became obvious. So cytochrome C release and activation of caspase-9 are time-dependent (A). It is also observed that pan-caspase inhibitor, z-VAD-fmk, also inhibit caspase-9 activation (B.)
Figure 6 The release of cytochrome C into cytosol and activation of caspase-9 in COS-1 cells by 15% SDA-PAGE. (A) The decrease of cytochrome C in mitochondrial, the release of cytochrome C in the cytosol, activation of procaspase-9 were examined in a time-dependent course. Lane 1, 2, 3 represents serum withdrawal for 12, 18, 24 hours, respectively, in SARS coronavirus N or mock-transfected COS-1 cells. (B) Effect of z-VAD-fmk on the activation of caspase-9 induced by N or mock-transfected COS-1 in the absence or presence of z-VAD-fmk (100 uM) under 24 hours' serum withdrawal. β Actin was loaded as loading controls. Pictures are representative of one of three similar results.
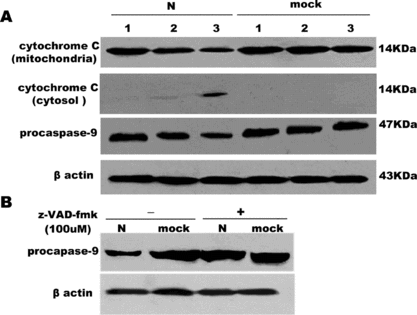
DISCUSSION
The outbreak of SARS caused a mortality rate of more than 10% as of August 2003. Lung, liver, and immune damage can be observed in SARS patients. These clinical symptoms may be directly or indirectly caused by apoptosis observed in pathological tissues of SARS patients. Lymphopenia is caused by apoptosis of lymphocytes. Liver impairment may be induced by apoptosis of hepatocytes, which was reported in three SARS patients. Inducing apoptosis can contribute to virus pathogenesis and inhibiting apoptosis can be beneficial for virus persistence. In many RNA viruses, virus-induced apoptosis is a common phenomenon. As a member of RNA viruses, some coronaviruses infection, such as mouse hepatitis virus [Citation[18]], feline infectious peritonitis virus [Citation[19]], transmissible gastroenteritis coronavirus [Citation[20]] and human coronavirus strain 229E [Citation[21]] can induce apoptosis. Apoptosis was observed in Vero E6 cells infected by SARS coronavirus [Citation[4]]. Previous research proved that many products of virus genomes can modulate apoptosis. The exact functions of SARS structure and non-structure proteins have not been clearly elucidated. In studies, many molecular aspects of the SARS nucleocapsid (N) protein have been reported, including self-dimerization [Citation[6]], RNA-binding capabilities [Citation[7]]. It is demonstrated that SARS coronavirus N protein can activate AP-1 signal transduction passway [Citation[8]], which is a regulator of a wide variety of cellular processes, including cell proliferation, differentiation, and apoptosis. So, N protein of SARS coronavirus may be important to modulate virus production and virus life cycles. Also, it is reported that SARS coronavirus N protein can induce apoptosis in COS-1 cells in the absence of growth factors. In this study, we observed that apoptosis was induced by SARS N protein (about 30%) under serum withdrawal in COS-1 cells, while in mock transfected COS-1 cells there was not significant apoptosis. Apoptosis was characterized by typical phosphatidylserine (PS) externalization () and chromatin condensation () in COS-1. At the same time, we have not observed obvious apoptosis induced by SARS coronavirus M, S protein in COS-1, HepG2, Huh-7 cells monitored by Annexin V () and electron microscopy (data not shown). However, in cultured liver cells HepG2 and Huh-7, SARS coronavirus N protein can not induce obvious apoptosis in the serum starving conditions ( and ) by the above methods. We agree with Surjit's opinion that we can not rule out the possibilities that this phenomenon may be due to the different abilities of different cell lines on tolerating stress.
Next, we focused our research on apoptosis pathway of SARS coronavirus N protein in COS-1 cells under serum withdrawal. Surjit and his colleagues have also demonstrated that N protein of SARS coronavirus induced apoptosis in COS-1 by activating caspase-3 and 7 [Citation[9]]. We confirmed that SARS N protein can activate caspase-3 and cleave its substrate of PARP. Since the general caspase inhibitor z-VAD-fmk inhibited the activation of caspase-3 and proteolysis of PARP, it is tempting to speculate that N protein of SARS coronavirus is able to initiate apoptosis using a caspase-dependent pathway ().
It is not clearly elucidated how caspase cascade is activated by N protein of SARS coronavirus in COS-1 cells. Caspases, a family of cysteine proteases, are critical mediators of programmed cell death, and thus far, 14 family members have been identified. Under normal conditions, caspases are present in the cytosol as inert proenzymes that pose no danger to the cells. Stimulated by apoptosis signal, caspases are activated in sequential cascade of cleavage by other caspase family members [Citation[11]]. Two main potentially interacting, cascade-initiating pathways converge on the activation of the downstream effector caspases (terminators such as caspase-3,7), that act to kill the cell by cleaving death substrates. The first of these pathways is through activation of caspase-8, which mediates signal transduction downstream of death receptors located on the plasma membrane. The second pathway is through activation of caspase-9, which mediates apoptotic signals after mitochondrial damage. In the latter process, caspase-9 is activated by an interaction with Apaf-1 associated with cytochrome C release from mitochondria, and it activates caspase-3 and caspase-7 to apoptosis. Cytochrome C release plays a key role in caspase-9-mediated apoptosis [Citation[10], Citation[22], Citation[23]]. Since mitochondria occupy a central role in the cascade of apoptosis, we further have research on mitochondria impairment. In the earlier stage of the mitochondrial impairment, increase of ROS and loss of the ΔΨm are the two early events. Some apoptotic stimuli induce the opening of the mitochondrial permeability transition (MPT) pore, resulting in increase of ROS and loss of the ΔΨm, swelling of the mitochondrial matrix, and consequent rupture of the outer mitochondrial membrane and the release of proapoptotic proteins, such as cytochrome C into cytoplasm [Citation[10], Citation[23], Citation[24], Citation[25]]. So, firstly we monitored ΔΨm and ROS under serum withdrawal in SARS N protein induced cell death (A and B). In SARS N transfected COS-1 cells, we observed a moderate right shift of the DCF fluorescence curves meaning the increase production of ROS and a moderate left shift of the DiOC6 fluorescence curves, meaning the decrease of ΔΨm in time course, demonstrating that mitochondrial are involved in the regulation of apoptotic cell death. Secondly, our experiments proved that the decrease of cytochrome C in mitochondria, the increase of cytochrome C in cytosol and the decrease of procaspase-9 in cytosol were all in time course by Western blot analysis (A). We also demonstrated that the release of cytochrome C into cytosol and activation of procaspase-9 became evident at 24 hours serum withdrawal. The activation of procaspase-9 can be inhibited by z-VAD-fmk(100 uM) at serum withdrawal for 24 hours (B).
In all, our results suggest that N-induced apoptosis in COS-1 is mediated by activation mitochondrial pathway after serum withdrawal. We thus demonstrate that the increase generation of the ROS in these cells, and consequent loss of ΔΨm, cytochrome C release, and caspase-3 and caspase-9 activation in SARS coronavirus N plasmid transfected COS-1 cells. Admittedly, our knowledge about SARS coronavirus is limited; there is some enigma about its pathogenesis mechanism, which may be caused by complex factors. More efforts are needed to explore the underlying mechanism of SARS and more time is needed to test our understandings about such severe infectious diseases.
The author acknowledges Professor Chen Jian Guo of Peking University Life Science Center for providing the vectors containing SARS coronavirus M, S, N gene. This work was supported by Basic Research of China (Grants 2005CB522902).
REFERENCES
- Nicholls, J.M., Poon, L.L., Lee, K.C., Ng, W.F., Lai, S.T., Leung, C.Y., Chu, C.M., Hui, P.K., Mak, K.L., Lim, W, Yan, K.W., Chan, K.H., Tsang, N.C., Guan, Y., Yuen, K.Y., Peiris, J.S. (2003). Lung pathology of fatal severe acute respiratory syndrome. Lancet. 361: 1773–1778.
- Ding, Y., Wang, H., Shen, H., Li, Z., Geng, J., Han, H., Cai, J., Li, X., Kang, W., Weng, D., Lu, Y., Wu, D., He, L., Yao, K. (2003). The clinical pathology of severe acute respiratory syndrome (SARS): A report from China. J Pathol. 200: 282–289.
- Lang, Z., Zhang, L., Zhang, S., Meng, X.L.J., Song, C., Sun, L., Zhou, Y. (2003). Pathological study on severe acute respiratory syndrome. Chin Med J (Engl). 116: 976–980.
- Yan, H., Xiao, G., Zhang, J., Hu, Y., Yuan, F., Cole, D.K., Zheng, C., Gao, G.F. (2004). SARS coronavirus induces apoptosis in Vero E6 cells. J Med Virol. 73: 323–331.
- Marra, M.A., Jones, S.J., Astell, C.R., Holt, R.A., Brooks-Wilson, A., Butterfield, Y.S., Khattra, J.K., Asano, S.A., Barber, S.Y., Chan, A., Cloutier, S.M., Coughlin, D., Freeman, N., Girn, O. L., Girffith, S.R., Leach, M., Mayo, H., McDonald, S.B., Montgomery, P.K., Pandoh, A.S., Petrescu, A.G., Robertson, J.E., Schein, A., Siddiqui, D.E., Smailus, J.M., Stott, G.S., Yang, F., Plummer, A., Andonov, H., Artsob, N., Bastien, K., Bernard, T.F., Booth, D., Bowness, M., Czub, M., Drebot, L., Fernando, R., Flick, M., Garbutt, M., Gray, A., Grolla, S., Jones, H., Feldmann, A., Meyers, A., Kabani, Y., Li, S., Normand, U., Stroher, G.A., Tipples, S., Tyler, R., Vogrig, D., Ward, B., Watson, R.C., Brunham, M., Krajden, M., Petric, D.M., Skowronski, C., Upton, and R.L., Roper. (2003). The genome sequence of the SARS-associated coronavirus. Science. 300: 1399–1404.
- Surjit, M., Liu, B., Kumar, P., Chow, V.T., Lal, S.K. (2004a). The nucleocapsid protein of the SARS coronavirus is capable of self-association through a C-terminal 209 amino acid interaction domain. Biochem Biophys Res Commun. 317: 1030–1036.
- Huang, Q., Yu, L., Petros, A.M., Gunasekera, A., Liu, Z., Xu, N., Hajduk, P., Mack, J., Fesik, S.W., Olejniczak, E.T. (2004). Structure of the N-terminal RNA-binding domain of the SARS Cov nucleocapsid. Biochemistry 43: 6059–6063.
- He, R., Leeson, A., Andonov, A., Li, Y., Bastien, N., Cao, J., Osiowy, C., Dobie, F., Cutts, T., Ballantine, M., Li, X. (2003). Activation of AP-1 signal transduction pathway by SARS coronavirus nucleocapsid protein. Biochem Biophys Res Commun. 311: 870–876.
- Surjit, M., Liu, B., Jameel, S., Chow, V.T., Lal, S.K. (2004). The SARS coronavirus nucleocapsid protein induces actin reorganization and apoptosis in COS-1 cells in the absence of growth factors. Biochem J. 383: 13–18.
- Green, D.R., Reed, J.C. (1998). Mitochondria and apoptosis. Science 281: 1309–1312.
- Desagher, S., Martinou, J.C. (2000). Mitochondria as the central control point of apoptosis. Trends Cell Biol. 10: 369–377.
- Thornberry, N.A., Lazebnik, Y. (1998). Caspases: Enemies within. Science 281: 1312–1316.
- Porter, A.G., Janicke, R.U. (1999). Emerging roles of caspase-3 in apoptosis. Cell Death Differ. 6: 99–104.
- Salvesen, G.S., Dixit, V.M. (1997). Caspases: intracellular signaling by proteolysis. Cell. 91: 443–446.
- Kane, D.J., Sarafian, T.A., Anton, R., Hahn, H., Gralla, E.B., Valentine, J.S., Ord, T., Bredesen, D.E. (1993). Bcl-2 inhibition of neural death: Decreased generation of reactive oxygen species. Science 262: 1274–1277.
- Fadok, V.A., Bratton, D.L., Frasch, S.C., Warner, M.L., Henson, P.M. (1998). The role of phosphatidylserine in recognition of apoptotic cells by phagocytes. Cell Death Differ. 5: 551–562.
- Smith, J.D., Waelde, C., Horwitz, A., Zheng, P. (2002). Evaluation of the role of phosphatidylserine translocase activity in ABCA1-mediated lipid efflux. J Biol Chem. 277: 17,797–17,803.
- Nishioka, W.K., and Welsh, R.M. (1993). B cells induce apoptosis via a novel mechanism in fibroblasts infected with mouse hepatitis virus. Nat. Immunol. 12: 113–127.
- Haagmans, B.L., Egberink, H.F., Horzinek, M.C. (1996). Apoptosis and T-cell depletion during feline infectious peritonitis. J Virol. 70: 8977–8983.
- Eleouet, J.F., Chilmonczyk, S., Besnardeau, L., Laude, H. (1998). Transmissible gastroenteritis coronavirus induces programmed cell death in infected cells through a caspase-dependent pathway. J Virol. 72: 18–24.
- Collins, A.R. (2002). In vitro detection of apoptosis in monocytes/macrophages infected with human coronavirus. Diagn Lab Immunol. 9: 1392–1395.
- Zou, H., Henzel, W.J., Liu, X., Lutschg, A., Wang, X. (1997). Apaf-1, a human protein homologous to C. elegans CED-4, participates in cytochrome c-dependent activation of caspase-3. Cell. 90: 405–413.
- Zou, H., Li, Y., Liu, X., Wang, X. (1999). An APAF-1 cytochrome C multimeric complex is a functional apoptosome that activates procaspase-9. J. Biol. Chem. 274: 11,549–11,556.
- Simon, H.U., Haj-Yehia, A., Levi-Schaffer, F. (2000). Role of reactive oxygen species (ROS) in apoptosis induction. Apoptosis. 5: 415–418.
- Jing, X., Ueki, N., Cheng, J., Imanishi, H., Hada, T. (2002). Induction of apoptosis in hepatocellular carcinoma cell lines by emodin. Jpn J Cancer Res. 93: 874–882.