Abstract
Retinol (Vitamin A) is known to destabilize membranes. The effect of one of its natural derivatives, Retinoic Acid, was therefore studied on membrane stability, by inversely correlating this stability with Phospholipase A2 activity. Lipid metalloporphyrin interactions have been the object of intense research in recent times. Our results revealed that the administration of Retinoic Acid (50,000 I.U.) caused a tremendous induction of hepatic Phospholipase A2 activity in Wistar rats. However when Retinoic Acid and Tin-mesoporphyrin (50 µmol/kgbw) were co-administered, hepatic Phospholipase A2 activity was inhibited. This result clearly reveals a possible therapeutic application of this metalloporphyrin in alleviating membrane destabilization, connected with Retinoic acid administration.
INTRODUCTION
Vitamin A, a fat soluble vitamin, is amphipathic and capable of partitioning into the lipid bilayer portion of membranes and it is there that the initial site for the action of the vitamin may be found [Citation[1]]. Natural and synthetic Vitamin A derivatives (retinoids) control a multitude of essential biological functions, Retinoic Acid (RA) being one such retinoid. RA is a chemotherapeutic agent and has been used to treat many tumor types. The treatment of breast cancer by RA may be mediated by lipid peroxidation. Elevated RA concentrations lead to increased lipid peroxidation as measured by thiobarbituric acid reactive substances. It has been postulated that the mechanism of growth inhibition by RA may be through lipid peroxidation [Citation[2]]. It is known that Vitamin A destabilizes membranes, resulting in increases in permeability of erythrocytes [Citation[3]], lysosomes [Citation[4]] and artificial lipid bilayers [Citation[5]]. In the present investigation, the effect of RA independently and in conjunction with metalloporphyrins was studied on hepatic Phospholipase A2 activity and on the concentration of hepatic glycerides, total cholesterol and phospholipids.
Free Retinol is known to rapidly associate with lipid bilayers. Recent studies also indicate that retinol transfers across and between phospholipid bilayers [Citation[6]]. The correlation between RA administration and membrane stability has not been studied so far. To elucidate whether RA alters membrane stability, the effect of RA independently and in conjunction with metalloporphyrins was observed on Phospholipase A2 activity. It is a well-established fact that the stability of many membranes, such as those of lysosomes and mitochondria, might be in reciprocal relationship with the activity of endogenous phospholipases bound to these membranes [Citation[7]]. Phospholipase A2 detaches the fatty acid from the b-position of phosphatidylcholine and lysolecithin formed has a detergent effect that can produce membrane destabilization [Citation[8]].
Lipid metalloporphyrin interactions have also been investigated extensively by various scientists. The interaction between hemin and lipid bilayers has been examined by Schmitt et al. [Citation[9]] from the point of investigating: (a) changes in membrane permeability; (b) changes in lipid organization; (c) catalysis of lipid peroxidation. Hemin is tightly aggregated in the aqueous phase upon binding to the membrane. Such aggregates are responsible for the enhancement of permeability resulting in membrane disorder.
Due to the above reasons, we attempted to investigate a possible role of the metalloporphyrins SnMP and FeMP independently and in conjunction with RA on the stability of hepatic mitochondrial membranes.
MATERIAL AND METHODS
Chemicals
Bovine serum Albumin (crystallized and lyophilized) was obtained from Sigma. SnMP and FeMP were purchased from porphyrin products, Logan, Utah, USA. The compounds were analyzed for purity by thin layer chromatography on pre-coated silica gel plates (Merck) using the following system: water, n-propanol and pyridine (5.5: 0.1: 0.4) by volume and then used without further purification. All other chemicals and reagents were of highest analytical grades available commercially.
Animals and Treatment
Male Wistar rats weighing 150–200 g were used in the investigation. The rats were selected at random from the stock colony maintained in the animal house facility, Dr. B.R. Ambedkar Center for Biomedical Research, University of Delhi. The animals were reared on laboratory chow, fed ad libitum and had free access to water at all times. The room was maintained at 25±2°C with natural day-time and no light after 1900 h, until morning.
Animals were divided into 6 groups of 6 animals each. Each group was fasted for 12 h before the experiment.
Group I.Animals of this group were treated as control and were administered an equivalent amount of saline subcutaneously.
Group II:50, 00 I.U. RA dissolved in groundnut oil was given to this group.
Group III:Animals in this group were administered 50 mmol/kgbw of SnMP subcutaneously.
Group IV:Animals in this group were administered 50 mmol/kgbw of SnMP subcutaneously along with 50,000 I.U. RA orally.
Group V:Animals in this group were administered 250 mmol/kgbw of FeMP subcutaneously.
Group VI:Animals in this group were given 250 mmol/kgbw of FeMP subcutaneously, along with 50,000 I.U. RA orally.
The solutions of FeMP and FePP were prepared fresh in small volumes in the dark, due to their photosensitivity. For making 1 ml of the solution of the porphyrin, the porphyrin was dissolved in 0.2 ml of .02 N NaOH and the volume was then made up by Potassium phosphate buffer (pH 7.4). RA was dissolved in groundnut oil.
Tissue Preparation
The animals were sacrificed using ether as an anesthetic. The liver was dissected out and cleaned with saline. Approximately 1 g of the tissue was kept for lipid estimation and the remaining was homogenized in a Potter-Elvejhem type glass homogenizer (pH 7.4) in three volumes of 0.1 M potassium phosphate buffer (pH 7.4) having 0.25 M sucrose according to the method of Hogeboom and Umbeit [Citation[10]]. All the homogenates were centrifuged at 10,000 g for 20 minutes. The pellet was dissolved in 2 ml of the above buffer and stored as the phospholipase A2 fraction.
Phospholipase A2 activity was assayed using phosphatidylcholine by the method of Bligh and Dyer [Citation[11]]. An incubation mixture consisting of 3 mmol (0.1 ml) of phosphatidylcholine, 5.8 mmol (0.3 ml) of sodium deoxycholate at pH 7.4 (0.2 M sodium phosphate buffer, 0.3 ml) and 3 mg of the phospholipase A2 fraction in a total volume of 0.8 ml. After 60 minutes of incubation at 37°C, the reaction was stopped by adding 2.5 volumes of methanol. 20 ml of chloroform, methanol in water in the ratio 1: 2: 1.8 was added to each tube and the contents were filtered. The upper layer was separated and removed and the lower layer was stored and estimated as phospholipids [Citation[12]].
Isolation of Lipids
For estimation of lipids, the tissue was weighed. Approximately 1 g of tissue was homogenized with 10 ml of methanol, then 20 ml of chloroform was added and the process continued for another two minutes. After filtering, the sediment was washed once more with chloroform (20 ml) and once with methanol (10 ml). The combined filtrates were transferred to the measuring cylinder and one quarter of the total volume of filtrate of 0.88% KCl in water was added. The mixture was shaken thoroughly and allowed to settle. The upper layer was removed by aspiration, and one quarter of the volume of the lower layer of water methanol was added. The purified lipid was obtained from the lower layer.
Estimation of Total Cholesterol
The total cholesterol levels were evaluated by the method of Zlatkis et al. [Citation[13]].
Estimation of Glycerides
The glyceride levels were evaluated by the method of Van Handel and Zilversmit [Citation[14]].
Estimation of Phospholipids
The levels of the various phospholipids and total phospholipid content were evaluated by the method of Ames [Citation[15]].
Estimation of Proteins
Protein concentrations were estimated by the method of Lowry et al. [Citation[16]].
Statistical Analysis
Statistical analysis of the data was performed using student's t-test. Data are expressed as Mean±SEM.
RESULTS AND DISCUSSION
Effect on the Concentration of Total Cholesterol
There was a negligible effect in the total cholesterol content when RA was administered. SnMP administration resulted in a moderate enhancement by ∼ 21% in total cholesterol content. There was a ∼ 1 fold stimulation when RA and SnMP were co-administered. When FeMP was administered, there was a tremendous increase in total cholesterol levels ().
Effect on the Concentration of Total Hepatic Glyceride Concentration
When RA was administered, there was a decrease of ∼ 68% in monoglyceride (mg) concentration, stimulation by ∼ 1 fold in the diglyceride (dg) content, and a significant increase in the triglyceride (tg) and esterified cholesterol (ec) concentration. When RA and SnMP were co-administered, there was a decline of ∼ 62% in mg concentration, a decrease of ∼ 39% in the dg concentration and, once again, extremely high concentrations of fc, tg and ec were observed.
When FeMP was administered, there was ∼ 36% decrease in the concentration of mg, an increase of ∼ 20% in the concentration of dg, an elevation of ∼62% in the concentration of tg and ∼1 fold increase in the concentration of both fc and ec. When FeMP and RA were co-administered there was a tremendous elevation in the concentration of all the glyceride fractions ().
Effect on the Concentration of Total Hepatic Phospholipid Concentration
On RA administration there was a decline of ∼ 26% in phosphatidylethanolamine (pe) concentration, a decrease of ∼ 27% in sphingomyelin (sp) concentration, an elevation of ∼ 1 fold in the concentration of phosphatidyl serine (ps) and a moderate rise of ∼ 32% in the concentration of phosphatidylinositol (pi).
On SnMP administration an increase of ∼ 16% in the concentration of pe and an elevation of ∼ 90% in pc concentration was observed. A moderate rise of ∼ 25% was observed in the concentration of sp, a rise of ∼ 26% and ∼ 53% was observed in the concentrations of ps and pi, respectively.
RA administration in conjunction with SnMP resulted in an increase of ∼ 26% in the concentration of pe, an elevation of ∼ 61% in pc concentration, a decline of ∼ 29% in the concentration of sp and an elevation of ∼ 1 fold in the concentration of pi. On FeMP administration there was a ∼ 41% elevation in the concentration of pe, and ∼ 1 fold elevation in pc content. An increase of ∼ 1 fold in the concentration of ps and ∼ 20% increase in the concentration of pi was observed. When FeMP and RA were coadministered there was a ∼ 40% increase in the concentration of pe, a rise of ∼ 54% in the concentration of pc, and an increase of ∼ 75% in pi concentration ().
Effect on the Concentration of Total Phospholipids
When RA was administered there was a slight increase in the concentration of total phospholipid. SnMP administration resulted in an increase of ∼ 21% in the concentration of total phospholipid. However, when RA and SnMP were co-administered, there was an enhancement of ∼ 1 fold in total phospholipid concentration. The coadministration of FeMP and RA led to a ∼ 4 fold increase in the concentration of total phospholipid ().
Effect on Hepatic Phospholipase A2 Activity
There was a tremendous elevation in Phospholipase A2 activity by ∼ 5 fold when RA was administered (). On independent administration of SnMP, hepatic Phospholipase A2 was stimulated by ∼ 2 fold. FeMP administration resulted in a significant increase of ∼ 6 fold in hepatic Phospholipase A2 activity. Co-administration of RA and FeMP caused, once again, a tremendous elevation of Phospholipase A2 activity. The administration of SnMP and RA, however, led to a decline of ∼ 33% in the activity of hepatic Phospholipase A2.
Effects on Total Mitochondrial Protein Concentration
On Retinoic Acid administration there was a decline of ∼ 63% in the concentration of total hepatic mitochondrial protein concentration. On co-administration of Retinoic Acid and SnMP there was a stimulation of ∼ 77% in protein concentration. There was a sharp decline of ∼ 87% when SnMP was administered. There was a moderate decline by ∼ 28% when RA was administered in conjunction with FeMP ().
Figure 7 Effect of RA (50,000 IU), SnMP (50 µmol/kgbwt), and FeMP (250 µmol/kgbwt) on total mitochondrial protein concentration.
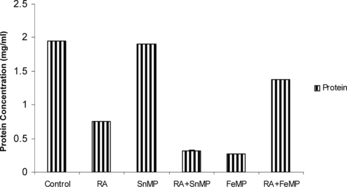
The biological activities of Retinoic Acid and several other Retinoids are mediated by Transcriptional activation by Retinoic Acid Response Element (RARE) and inhibition of AP-1 activity, acting through distinct nuclear receptors, namely the Retinoic Acid Receptors (RARs) and the Retinoid X receptors (RXRs). For RA to mediate its transcriptional effect (at the nuclear level) it must interact with cellular phospholipid membranes and thus affect Phospholipase A2 activity. A model of enzyme action proposed by Verger et al. [Citation[17]] states that Phospholipase A2 binds first to the lipid-water interface. This produces an interfacially activated enzyme, which subsequently binds the phospholipid substrate. The substrate is then hydrolyzed to produce lysophospholipids and the free acid.
Our results clearly demonstrate that RA tremendously enhances Phospholipase A2 activity in male Wistar rats. However the co-administration of RA and SnMP inhibits Phospholipase A2 activity. SnMP may therefore be a potential therapeutic agent for preventing membrane lysis brought about by RA.
Our results also demonstrate that RA has only negligible effect on the concentration of total cholesterol. On SnMP administration there was a moderate rise in the concentration of total cholesterol. Co-administration of RA and SnMP produced an ∼ 1 fold increase in the concentration of total cholesterol, thereby enhancing membrane stability. Our results provide a prototype to pursue further studies in this direction.
The author (MT) wishes to acknowledge the financial assistance provided by CSIR. The author (RC) thankfully acknowledges the University Grants Commission of India (UGC, India) for the Career Award and the Rockefeller Foundation, USA, for the Biotechnology Career Award.
REFERENCES
- Dingle, J.T., Lucy, J.A. (1965). Vitamin A, carotenoids and cell function. Biol Rev Camb Philos Soc. 40: 422–61.
- Crowe, D.L. (1998). Retinoic acid receptor beta induces terminal differentiation of squamous cell carcinoma lines in the absence of cyclin-dependent kinase inhibitor expression. Cancer Res. 58(1): 142.
- Dingle, J.T., Lucy, J.A. (1962). Studies on the mode of action of excess of vitamin A. 5. The effect of vitamin A on the stability of the erythrocyte membrane. Biochem. J. 84: 611.
- Dingle, J.T., Lucy, J.A., Fell, H.B. (1961). Studies on the mode of action of excess of vitamin A. 1. Effect of excess of vitamin A on the metabolism and composition of embryonic chick-limb cartilage grown in organ culture. Biochem. J. 79: 497.
- Fell, H.B., Dingle, J.T., Webb, M. (1962). Studies on the mode of action of excess of vitamin A. 4. The specificity of the effect on embryonic chick-limb cartilage in culture and on isolated rat-liver lysosomes. Biochem. J. 83: 63.
- Stillwell, W., Nahmias, S. (1983). Effect of retinol and retinoic acid on P/O ratios of coupled mitochondria. Biochem Int. Mar. 6(3): 385–92.
- Rossi, C.R., Sartoelli, L., Siliprendi, N. (1980). On the biochemical significance of phosphoserine: a working hypothesis. Ital J Biochem. 29(1): 21–6.
- Chandra, R., Beri, R., Misra, U.K. (1988). Effect of spermine on membranolytic effect of vitamin A in rats. Int J Vitam Nutr Res. 58(1): 13–5.
- Schmitt, T.H., Frezzatti, W.A., Schreier, S. (1993). Hemin-induced lipid membrane disorder and increased permeability: a molecular model for the mechanism of cell lysis. Arch. Biochem. Biophys. 307: 96.
- Hogeboom, G.H. (1955). in Methods in Enzymology, S.P. Colowick, S.P. Kaplar, Eds. Academic Press Inc.: New York, Vol. 1, p. 16.
- Bligh, E.G., Dyer, W.J. (1959). A rapid method of total lipid extraction and purification. Canad. J. Biochem. Biophys. 37: 911.
- Zlatkis, A., Zak, B., Boyle, A.J. (1953). A new method for the direct determination of serum cholesterol. J. Lab. Clin. Med. 41: 486.
- Hanel, H.K., Dam, H. (1954). Alimentary production of gallstones in hamsters. 5. Cholesterol synthesis from 2-C-14-acetate by the liver in vivo. Acta Pathol Microbiol Scand. 35(5): 423–425.
- Van Handel, E., Zilversmit, D.B. (1957). Micromethod for the direct determination of serum triglycerides. J. Lab. Clin. Invest. 50: 152.
- Ames, B.N. (1966). in Methods in Enzymology, and V. Gruisberg, Eds., Academic Press, Inc.: New York, 8, p. 115.
- Lowry, O.H., Rosenbrough, N.I., Farr, A.L., Randell, R.J. (1951). Protein measurement with the Folin phenol reagent. J Biol Chem, 193: 265.
- Lange, Y., Murasaki, M.F. (1987). Cholesterol is not synthesized in membranes bearing 3-hydroxy-3-methylglutaryl coenzyme A reductase. J. Biol. Chem. 262: 4433–4436.