Abstract
The article is devoted to the theoretical aspects of the development of the effective method for the removal of protein-bound uremic toxins. It is shown that the methods of flow and differential scanning microcalorimetry are sufficient enough for the evaluation of the degree of ligand loading of human serum albumin with protein-bound uremic toxins.
The molecules of albumin isolated from blood plasma of the patients being kept on chronic dialysis are demonstrating significant alterations of conformation and complex-forming properties, the correction of which by conventional methods of extracorporeal detoxification (exhaustive dialysis, treatment on synthetic SCN carbons) are practically ineffective. Deliganding of uremic albumin may be successfully performed on conventional carbon haemosorbents upon preliminary separation of blood plasma and its dilution with acetate buffer 1:1 at pH = 5.08. Treatment of the whole blood of patients onto new massfractal deliganding carbon, i.e., hemosorbents of HSGD trademark. These HSGD haemosorbents quite effectively could be used for restoration of main parameters of uremic HAS molecules conformation and ligand-binding activity simultaneously with hemodialysis upon the protection by locally performed citrate anticoagulation as an easier and cheaper method for the removal of protein-bound uremic toxins.
There is no doubt that the problem of extracorporeal removal of protein-bound uremic toxins is one of the most important tasks of the therapy of the terminal stage of chronic renal insufficiency [Citation[1]]. Some time ago is was stated that transport proteins of blood plasma of uremic patients and, first of all, serum albumin possess the decreased affinity to medicinal preparations due to their overloading with protein-bound endogenous metabolites. Let's note that, according to classification [Citation[2]], albumin possesses 3 main centers for binding pharmaceuticals. As noted in , the complex-forming properties of all these centers in albumin isolated from uremic plasma are suppressed, while the binding capacity of such plasma is decreased, respectively ().
Table 1. Binding capacity of albumin isolated from blood plasma of uremic patients
According to modern views [Citation[9]], the significant number of compounds being at some level protein-bound () is taking part in the realization of uremic toxicosis.
Table 2. Protein-bound uremic toxins
Let's select from that list 3 protein-bound uremic toxins () that are characterized by strong, medium and low affinity to human serum albumin, i.e. CMPF, indoxyl sulphate and hippuric acid, respectively [Citation[10]].
In the opinion of Tsutsumi et al. [Citation[11]], these 3 metabolites, i.e. CMPF (3-carboxy-4-methyl-5-propyl-2furanpropionoc acid), indoxyl sulphate (IS) and hippuric acid (HA), are the most important inhibitors of binding of pharmaceuticals with uremic HSA. CMPF is a typical representative of urofuranoid acids possessing strongly pronounced lipophylic properties and high (near 108 M−1) constant of association with HSA molecule. At normal state that metabolite is excreted in unaltered form in urine, being involved, due to its high affinity to albumin, in active tubular secretion [Citation[12]]. CMPF suppresses the activity of glutathione–S-transferase and production of iodine in rat hepatocytes [Citation[13]], possesses certain nephrotoxicity and inhibits erythropoiesis [Citation[14]]. That uremic toxin suppresses the binding of non-conjugated bilirubin and a number of medicinal preparations, including furosemide, with HSA [Citation[15]].
In healthy donors indoxyl sulphate (IS) is excreted in urine by tubular secretion. Accumulation of that metabolite possessing medium affinity to HSA, in the fluids of the body of uremic patients, is thought to be linked to cerebral disfunction, disturbance of iodine metabolism and acceleration of the progression of renal insufficiency associated, for example, with an increased index of glomerular sclerosis [Citation[16]]. Indoxyl sulphate is also suppressing hepatocyte' transport of thyroxine and formation of colonies of erythroblasts and lymphoblasts in cell cultures of bone marrow [Citation[17], Citation[18]]. Among preparations and metabolites the binding of which with HSA became negatively affected in the presence of elevated concentration of indoxyl sulphate, one may name diazepam, warfarin, L-tryptophane, furosemide, salicylates [Citation[19-22]].
Hippuric acid (HA) is least of all tightly bound with HSA. Like CMPF and IS, it suppresses the production of iodine by hepatocytes, but for manifestation of such effect the concentration of that metabolite should be 10-fold higher than the concentration of CMPF. Among pharmaceuticals with which hippuric acid competes for transport sites of HSA, without a doubt salicylates should be mentioned [Citation[20]].
a presents the melting curve of purified (defatted) HSA received by the method of differential scanning microcalorimetry (DSMC). That method is very sensitive to the degree of HSA liganding, and therefore very suitable for evaluation of the loading of mentioned transport protein with different hydrophobic toxins and metabolites [Citation[23]]. As it follows from b, CMPF renders the strongest influence on the results of DSMC, increasing the temperature of denaturation of albumin and transforming unimodal curve of its melting to a bimodal one. One may see it especially clearly in , where upon the increase of CMPF/albumin relation to 2:1 the melting curve is practically identical to that for the complex “albumin–nonconjugated bilirubin” [Citation[24]].
Figure 1 Melting thermograms of defatted HSA before (a) and after (b) loading with protein-bound uremic toxins. Dotted lines describe the results of this curve mathematical deconvolution onto elementary peaks.
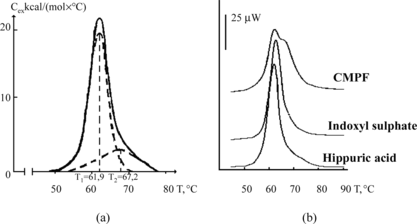
For evaluation of the degree of loading of discrete transport centers of albumin with hydrophobic metabolites, the method of flow microcalorimetry is being successfully used; it consists of the precise measurement of amount of heat excreted or absorbed during the process of complex-formation of albumin () with marker ligands addressed to certain binding sites of the molecule of that protein [Citation[25]]. Naturally, upon the increase of the degree of occupation of each concrete binding center, an absolute value of heat (enthalpy) of complex formation is decreasing. That is clearly seen in , where the addition of IS and HA (but not CMPF) causes the apparent alterations in the heats of binding of marker ligands, exactly salicylic acid and phenol red.
Table 3. Parameters of complex-formation for HSA with the 3 typical protein-bound uremic toxins
Table 4. Enthalpy of complex-forming abilities (kJ/M) of albumin preparations with marker ligands with respective affinity to binding centers of protein
In turn, the elevation of concentration (0, 60, 120, 180, 240 and 300 µmol) of CMPF, possessing trophy toward bilirubin's binding center on albumin molecule, notably elevates the content of free fraction of bromophenol blue (from 7 up to 24%) that is considered the standard marker of bilirubin's center, thus demonstrating the increased degree of occupation exactly of that center of the molecule by this uremic toxin.
As one may see from the mentioned examples, the methods of differential scanning and flow microcalorimetry in combination with the classic method of equilibrium dialysis provide complete information on the changes of molecular conformation and complex-forming activity of HSA molecules upon the loading by typical representatives of protein-bound uremic toxins in model studies and, consequently, they may be adequately used for evaluation of the respective alterations of HSA isolated from blood plasma of uremic patients. In particular, one should note that the method of isolation of HSA from blood plasma of uremic patients should be delicate enough, i.e. it should favor the preservation of “ligand coating” of the protein-carrier molecule [Citation[26]].
represents the differences in melting thermograms of albumin fractions isolated from blood plasma of healthy donors and uremic patients undergoing chronic hemodialysis treatment. That phenomenon has been studied in detail on 47 patients undergoing chronic alimentary dialysis from 6 months to 20 years [Citation[27]]. As follows from this picture, the most pronounced deviations in the shape of melting curves of albumin are observed in patients undergoing dialysis from 1 to 5 years. The analogous results have been obtained on enthalpy of binding of marker ligands (), and upon the study of spectra of fluorescence of tryptophane where the respective curve for the period of 1–5 years is the most different from that for HSA of healthy donors (data not presented).
Figure 3 Melting thermograms of albumin fraction isolated from blood plasma of uremic patients undergoing chronic alimentary hemodyalysis for 6 months–20 years.
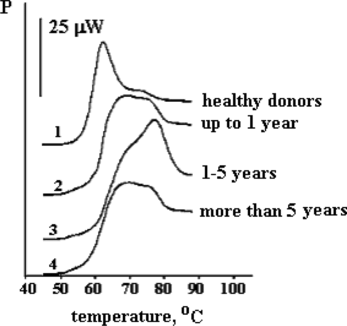
Table 5. Enthalpy of complex-formation of marker ligands (ΔHc, kJ/Mol) with albumin of healthy donors and uremic patients undergoing hemodialysis of different duration
At first glance, the fact that upon increased duration of alimentary hemodyalysis in uremic patients the conformation and complex-forming characteristics of uremic albumin are becoming evidently better is paradoxical. Nevertheless, it could be explained that not only is plasma albumin content an important prognostic factor in prediction of survival rate of dialysis patients [Citation[28]], but also functional abilities of that transport protein should be taken into account.
All abovementioned points on the expediency of the active extracorporeal removal of protein bound uremic toxins that may be substantially narrowed down to the development of the methods of normalization of conformational and transport functions of the molecule of uremic albumin. Nevertheless, that task is complex enough. More than 10 years ago, Dr. John Daugirdas [Citation[29]] has described the different problems for the removal of hydrophobic uremic toxins that have been formulated as three questions of the “dialysis doctor”:
To what extent the protein-bound compounds possessing toxic properties are removed upon standard dialysis, high-flax-dialysis or hemofiltration, and isn't it better to remove these compounds using peritoneal dialysis;
If some protein-bound components are removed improperly, is there the proof for the fact that their accumulation in the body causes toxic effects. Also, one should distinguish toxicity caused by protein-bound toxins as they are, and toxicity that depends on the occupation by them of special sites on serum albumin, and the elevation of concentration of free fractions of other toxic compounds displacing uremic metabolites from their normal bonds with transport protein;
Do the practical methods for elevating the removal of protein-bound components during hemodialysis exist? Are there some other additive methods, including diet, that allow one to decrease plasma concentration of toxic protein-bound components in patients with uremia?
The first two questions somehow have found their explanation in multiple studies on the problem of protein-bound uremic toxins [Citation[30], Citation[31], Citation[23]], but the third question of the “dialysis doctor” still remains unanswered.
The reason for the inefficiency of conventional dialysis is the discrepancy of the size of pores of dialysis membrane and the size of protein molecules containing tightly-bound ligands. Moreover, the use of heparin activating lipoprotein lipase during dialysis is leading to enhancement of liganding of HSA molecule by non-esterified fatty acids and “deterioration” of the mode of melting curves of uremic albumin (the shift of the maximum of curve to the right) due to elevation of its loading with these hydrophobic metabolites (data not presented).
As follows from , the binding capacity of transport sites of albumin, especially of the site for middle-chained fatty acids (octanoate) slightly decreases. The parameters of binding for HSA of the patients undergoing constant peritoneal dialysis are practically not different from those in patients before hemodyalysis, except unexpected increase of functional potency of the center of long-chained fatty acids tested by SDS.
Table 6. Enthalpy of complex formation (kJ/M) of albumin preparations with marker ligands for respective affine binding centers of protein
At the same time, the shape of melting curves of HSA of patients undergoing hemodialysis is practically similar to that in patients undergoing peritoneal dialysis, demonstrating the inefficacy of both methods for the removal of the mostly tightly bound to albumin uremic toxins [Citation[32]].
As follows from , neither “depleting” (24 hours) laboratory dialysis of blood plasma of uremic patients, nor its purification on conventional synthetic carbon SCN haemosorbents, alters significantly the shape of melting curves of albumin, nor its complex-formation properties. At the same time, it is known that one may achieve sufficiently good purification of serum albumin on standard activated carbons using pH-dependent induction of conformation of the protein [Citation[33]].
Figure 4 Demonstration of inefficacy of exhaustive dialysis and contact with SCN carbons of blood plasma of uremic patients for the removal of protein-bound toxins obtained by the methods of differential (a, b, c) and flow microcalorimetry (d).
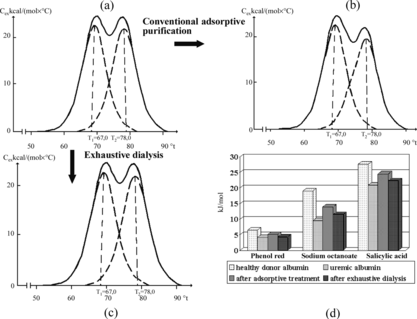
It was demonstrated that albumin preparations isolated from blood plasma of uremic patients pre-treated by purification on conventional SCN-2K carbonic haemosorbent at acidic range of pH (pH = 3) improved the activity of the majority of binding sites towards specific marker ligands (). However, the treatment of plasma at pH = 3 is too “rigorous” to preserve the native state of all proteins of uremic plasma. At the same time, at least one legalized medical technology where the adsorptive purification of blood plasma occurs at significantly lowered pH is known [Citation[34]]. That technology, known as HELP and directed on the removal of atherogenic lipoproteins of low and very low density, results in: 1) separation of plasma; 2) dilution of plasma with acetate buffer (pH = 5.08) at the relation 1:1; 3) precipitation of LPLD with heparin; and 4) removal of the precipitate and heparin. Naturally, in that case we are interested only in the part concerning acidification of blood plasma.
Table 7. Influence of adsorptive purification of uremic plasma at different pH on the value of enthalpy (kJ/M) of complex formation of uremic HSA with marker ligands
As follows from , the melting curve of uremic albumin isolated from plasma that underwent the dilution with acetate buffer (pH = 5.08) and the next purification on SCN carbons look somehow better than that obtained upon purification at pH = 3, while the purification at pH = 7 is practically ineffective. That result is in accordance with the data of flow microcalorimetry () and the data obtained by equilibrium dialysis with the dye methyl red.
Figure 5 Melting thermograms of HSA isolated from uremic plasma before (a) and after adsorptive purification at pH = 7.2 and 3.0 (b and d), and after dilution with acetate buffer (pH = 5.08) with the next purification on carbonic adsorbent (c).
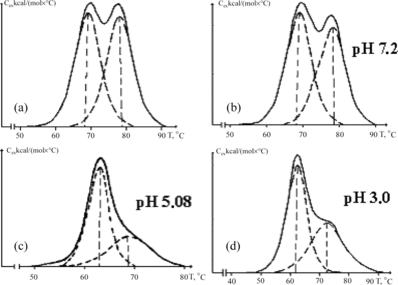
Table 8. Enthalpy of complex formation (kJ/M) of marker ligands with preparations of HSA isolated from uremic plasma treated by purification on the adsorbent at different pH values and acetate buffer dilution (ACB)
So, in principle, the procedure parallel to the dialysis may be performed (see scheme in ) when blood plasma of the uremic patient is being diluted in an “on-line” regimen by acetate buffer, undergoes the treatment onto carbonic adsorptive columns and returns to the main contour before the entrance to the traditional system for bicarbonate dialysis, that is responsible apart from other functions for the normalization of pH and removal of the excess of acetate and water. However, such a system, despite its apparent perspective for solving the tasks for the removal of protein-bound uremic toxins, still is considered too bulky and expensive for it to be reasonable from a practical point of view.
Figure 6 Principal scheme for the purification of blood plasma of uremic patients with the use of acetate buffer.
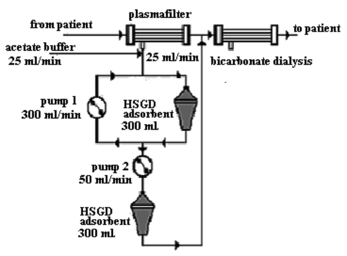
Recently, we have developed a new type of carbonic haemosorbents specially designed for the removal of protein-bound compounds [Citation[35]]. presents the results of transformation of conventional synthetic carbon obtained by carbonization and activation of phenolformaldehyde resins to the haemosorbent special toward protein-bound metabolites (nonconjugated bilirubin) that preserves the original properties toward free-soluble compounds of low molecular weight (creatinin) upon a somewhat elevated capacity to the marker of compounds of middle molecular weight substance–vitamin B12.
Table 9. Transformation of conventional phenolformaldehyde carbon (sample 1) into deliganding form (sample 2)
These haemosorbents produced on the base of pyrolysis of nitrogen-containing synthetic resins under the mark HSGD (HaemoSornenta Granulated Deliganding) possess so-called fractal, i.e. “broken,” structure of the innate surface where instead of standard hierarchy branching of pores (macropores-mesopores-micropores), the co-existence of all their types in each unit of the adsorbent's volume is supposed [Citation[36]]. The degree of fractality of carbonic adsorbents has been evaluated by an index α obtained by analysis of the curves of small-angle neuron scattering (SANS).
As follows from , enhancement of the values of coefficient α from −3.51 to −2.39 (the range where, possibly, the transition from superficially fractal to mostly mass-fractal structure occurs) correlates with the sharp (more than 100-fold) increase of sorption of nonconjugated bilirubin from 3% solution of HSA. Conventional carbonic haemosorbents of SCN class (α = − 3.51) possess moderate adsorptive capacity by that index (near 0.6–1.0 mg/g).
Table 10. Correlation between the degree of fractality of carbonic adsorbent (α) and adsorption of nonconjugated bilirubin (mg/g) from albumin-containing solution
High adsorptive capacity of carbonic haemosorbents of HSGD trade-mark may be explained, for example, by the short diffuse way passed by the complex “carrier-ligand” from the place of its primary position to the place of adsorptive binding of ligand and the wide range of the sizes and shapes of adsorbent pores placed in each micro-volume of granules, which allows one to find their appropriate configuration responding to actual conformational state of protein-ligand complex.
Application of HSGD haemosorbents allows the creation of the original device for effective pre-hepatocytic removal of bile components and their precursors (apparatus “artificial liver”) [Citation[24]].
The ideology of the use of deliganding massfractal haemosorbents is, apparently, reasonable enough for also solving the tasks for the removal of non-protein-bound and weakly protein-bound uremic toxins. Comparative measurement of standard capacity of two trademark haemosorbents towards uric acid, which is presented mostly in a free form, demonstrated the high preference of HSGD over SCN haemosorbents (1750 and 490 mg/g, respectively). As follows from A, the contact with HSGD haemosorbent completely restores the normal shape of melting curves of albumin loaded with CMPF and effectively purified the model mixtures of HSA supplemented by CMPF, indoxyl sulphate and hippuric acid in 4 hours microcolumn experiments (B). Experimental time of adsorption has been selected, taking into account the duration of hemodialysis session.
Figure 7 Melting thermograms of CMPF-HSA complex before (1) and after (2) the contact with deliganding HSGD haemosorbent (a) and decrease of concentration of CMPF, indoxyl sulphate (IS) and hippuric acid (HA) after sorptional purification on HSGD carbons in microcolumn experiments (b).
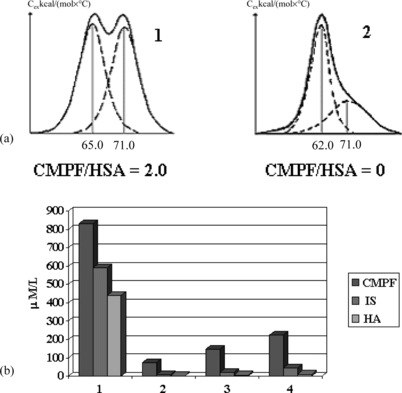
As follows from , the treatment of the total plasma of uremic patients on HSGD haemosorbents leads to the restoration of practically normal shape of melting curves of uremic albumin and complex-forming capacity of its binding sites that undoubtedly points to the high level of purification of blood plasma of uremic patients from protein-bound toxins. It's necessary to note that all the abovementioned is related only to blood plasma of dialysis patients not suffering from diabetes because, in the last case, the restoration of normal conformation-acceptor properties of albumin is hindered due to the presence of covalent bonds between that protein and final products of glycation and oxidation [Citation[37], Citation[38]].
Figure 8 Melting curves and complex forming ability of HSA obtained from blood plasma of healthy donors and non-diabetic uremic patients preliminarily treated on HSGD haemosorbents.
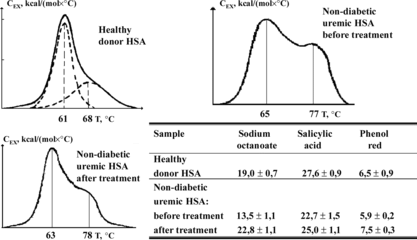
So, for the removal of protein-bound toxins from the body of the majority of patients undergoing chronic supportive dialysis, the next easy scheme not requiring separation of plasma and its dilution with acetate buffer may be proposed (). The problem of regulation of the levels of heparinization, which are essentially different for dialysis and haemocarboperfusion [Citation[35]], is easily solved in that scheme using additional citratization of blood passing through haemosorbent [Citation[36]] with the next removal of the excess of citrate and restoration of ion balance by dialysis unit.
Figure 9 Principal scheme for the removal of protein-bound uremic toxins from blood of patients with renal insufficiency (from patient – pump 1 300 ml/min – pump 2, 30 ml/min – three-substituted citrate – deliganding adsorbent – to patient – dialysator).
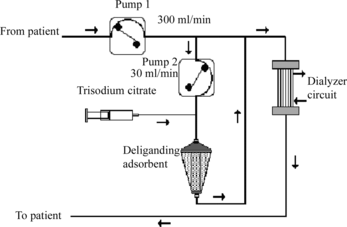
An application of these HSGD haemosorbents in the treatment of children with multi-organ failure caused by heavy poisoning with death cap allows one to reduce up to 6-fold the lethality compared with the variant of therapy based on the use of conventional carbonic haemosorbents ().
Table 11. HSGD carbons in the therapy of heavy forms of poisoning of children with death cap
All this is making realistic the perspectives of effective application of carbonic mass-fractal and combined adsorbents, not only in the therapy of acute and chronic renal insufficiency, but also the control of other heavy clinical states related, in particular, to the manifestation of the syndrome of systemic inflammatory response and poly-organic insufficiency.
CONCLUSIONS
The methods of flow and differential scanning microcalorimetry are appropriate for evaluation of the level of loading of human serum albumin with protein-bound uremic toxins.
The molecule of albumin isolated from blood plasma of the patients undergoing chronic hemodialysis demonstrates significant alterations of conformation and complex-forming properties, the correction of which by routinely used methods of extracorporeal detoxification (dialysis, treatment on synthetic carbons of SCN type) is practically impossible.
Deliganding of uremic albumin may be successfully performed on conventional carbonic hemosorbents upon preliminary separation of plasma and its dilution with acetate buffer at the relation 1:1 at pH = 5.08.
A potentially easier and economically more acceptable scheme for the removal of protein-bound uremic toxins relays in the treatment of the whole blood of patients on deliganding haemosorbents of HSGD carbons performed simultaneously with hemodyalysis upon the protection of locally done citrate anticoagulation.
This work was supported by INTAS-04-082-7065 grant program.
REFERENCES
- Boure, T., Vanholder, R. (2004). Biochemical and clinical evidence for uremic toxicity. Artif. Organs. 28: 248–253.
- Sudlow, G., Birkett, D.J., Wade, D.N. (1976). Further characterization of specific drug binding sites on human serum albumin. Mol. Pharmacol. 12: 1052–1061.
- Dasgupta, A., Paul, A., Wells, A. (2001). Uremic sera contain inhibitors that block digitoxin-valproic acid interaction. Am. J. Med. Sci. 322: 204–208.
- Sakai, T., Yamasaki, K., Sako, T., Kragh-Hansen, U., Suenaga, A., Otagiri, M. (2001). Interaction mechanism between indoxyl sulfate, a typical uremic toxin bound to site II, and ligands bound to site I of human serum albumin. Pharm. Res. 18: 520–524.
- Viani, A., Rizzo, G., Carrai, M., Pacifici, G.M. (1992). The effect of ageing on plasma albumin and plasma protein binding of diazepam, salicylic acid and digitoxin in healthy subjects and patients with renal impairment. Br. J. Clin. Pharmacol. 33: 299–304.
- Mabuchi, H., Nakahashi, H. (1988). Displacement by anionic drugs of endogenous ligands bound to albumin in uremic serum. Ther. Drug Monit. 10: 261–264.
- Calvo, R., Carlos, R., Erill, S. (1985). Underestimation of albumin content by bromocresol green, induced by drug displacers and uremia. Int. J. Clin. Pharmacol. Ther. Toxicol. 23: 76–78.
- Priborsky, J., Takayama, K., Nagai, T. (1998). Influence of acute uraemia on percutaneous absorption of nonsteroidal anti-inflammatory drugs. Acta. Univ. Palacki. Olomuc. Fac. Med. 41: 35–38.
- Vanholder, R., Glorieux, G., Lameire, N. (2005). New insights in uremic toxicity. Contrib. Nephrol. 149: 315–324.
- Sakai, T., Takadate, A., Otagiri, M. (1995). Characterization of binding site of uremic toxins on human serum albumin. Biol. Pharm. Bull. 18: 1755–1761.
- Tsutsumi, Y., Deguchi, T., Takano, M., Takadate, A., Lindup, W.E., Otagiri, M. (2002). Renal disposition of a furan dicarboxylic acid and other uremic toxins in the rat. J. Pharmacol. Exp. Ther. 303: 880–887.
- Henderson, S.J., Lindup, W.E. (1992). Renal organic acid transport: Uptake by rat kidney slices of a furan dicarboxylic acid which inhibits plasma protein binding of acidic ligands in uremia. J. Pharmacol. Exp. Ther. 263: 54–60.
- Lim, C.F., Bernard, B.F., de Jong, M., Docter, R., Krenning, E.P., Hennemann, G. (1993). A furan fatty acid and indoxyl sulfate are the putative inhibitors of thyroxine hepatocyte transport in uremia. J. Clin. Endocrinol. Metab. 76: 318–324.
- Niwa, T., Yazawa, T., Kodama, T., Uehara, Y., Maeda, K., Yamada, K. (1990). Efficient removal of albumin-bound furancarboxylic acid, an inhibitor of erythropoiesis, by continuous ambulatory peritoneal dialysis. Nephron 56: 241–245.
- Takamura, N., Maruyama, T., Otagiri, M. (1997). Effects of uremic toxins and fatty acids on serum protein binding of furosemide: Possible mechanism of the binding defect in uremia. Clin. Chem. 43: 2274–2280.
- Niwa, T., Ise, M., Miyazaki, T. (1994). Progression of glomerular sclerosis in experimental uremic rats by administration of indole, a precursor of indoxyl sulfate. Am. J. Nephrol. 14: 207–212.
- Lim, C.F., Bernard, B.F., de Jong, M., Docter, R., Krenning, E.P., Hennemann, G. (1993). A furan fatty acid and indoxyl sulfate are the putative inhibitors of thyroxine hepatocyte transport in uremia. J. Clin. Endocrinol. Metab. 76: 318–324.
- Kawashima, Y. (1989). Study on the uremic protein binding inhibitors as uremic toxin: Toxic effect on erythroid colony formation, lymphocyte blast formation and renal function. Nippon. Jinzo. Gakkai. Shi. 31: 1151–1161.
- McNamara, P.J., Lalka, D., Gibaldi, M. (1981). Endogenous accumulation products and serum protein binding in uremia. J. Lab. Clin. Med. 98: 730–740.
- Bowmer, C.J., Lindup, W.E. (1982). Decreased drug binding in uraemia: Effect of indoxyl sulphate and other endogenous substances on the binding of drugs and dyes to human albumin. Biochem. Pharmacol. 31: 319–323.
- Ikeda, K., Yoshitomi, H., Nakayama, T., Goto, S., Kimura, T. (1984). Plasma protein binding of frusemide in renal failure rabbits: Investigation of endogenous protein binding inhibitors. J. Pharm. Pharmacol. 36: 663–667.
- Niwa, T., Takeda, N., Tatematsu, A., Maeda, K. (1988). Accumulation of indoxyl sulfate, an inhibitor of drug-binding, in uremic serum as demonstrated by internal-surface reversed-phase liquid chromatography. Clin. Chem. 34: 2264–2267.
- Sarnatskaya, V.V., Lindup, W.E., Niwa, T., Ivanov, A.I., Yushko, L.A., Tjia, J., Maslenny, V.N., Korneeva, L.N., Nikolaev, V.G. (2002). Effect of protein-bound uremic toxins on the thermodynamic characteristics of human albumin. Biochem. Pharmacol. 63: 1287–1296.
- Nikolaev, V.G., Sarnatskaya, V.V., Bardakhyvskaya, K.I., Susak, Ya. M., Snejkova, E.A., Chernomyz, V.D., Tsymbalyuk, R.S., Uvarov, V.Yu., Gurina, N.M. (2003). Efferent Therapy 9: 26–39 (in Russian).
- Coassolo, P., Sarrazin, M., Sari, J.C., Briand, C. (1978). Microcalorimetric studies on the binding of some benzodiazepine derivatives to human serum albumin. Biochem. Pharmacol. 27: 2787–2792.
- Vasileva, R., Jakab, M., Hasko, F. (1981). Application of ion-exchange chromatography for the production of human albumin. J. Chromatogr. 30: 279–284.
- Sarnatskaya, V.V., Ivanov, A.I., Nikolaev, V.G., Rotellar, E., von Appen, K., Haspar, M., Maslenny, V.N., Klinkmann, H. (1998). Structure and binding properties of serum albumin in uremic patients at different periods of hemodialysis. Artif. Organs. 22: 107–115.
- Port, F.K. (1994). Morbidity and mortality in dialysis patients. Kidney Int. 46: 1728–1737.
- Daugirdas, J.T., Schneditz, D. (1995). Protein bound toxins in uremia. ASAIO J. 41: M719–M724.
- Vanholder, R., De Smet, R., Glorieux, G., Argiles, A., Baurmeister, U., Brunet, P., Clark, W., Cohen, G., De Deyn, P.P., Deppisch, R., Descamps-Latscha, B., Henle, T., Jorres, A., Lemke, H.D., Massy, Z.A., Passlick-Deetjen, J., Rodriguez, M., Stegmayr, B., Stenvinkel, P., Tetta, C., Wanner, C., Zidek, W. (2003). European Uremic Toxin Work Group (EUTox). Review on uremic toxins: classification, concentration, and interindividual variability. Kidney Int. 63: 1934–1943.
- Ward, R.A. (2005). Protein-leaking membranes for hemodialysis: A new class of membranes in search of an application? J. Am. Soc. Nephrol. 16: 2421–2430.
- Ivanov, A.I., Sarnatskaya, V.V., Maslenny, V.N. (1997). Comparative study of albumin termodynamic parameters in ESRD patients treated by HD and CAPD. Abstracts of 3rd Intern. Symp. on Uremic Toxicity, Nagoya, Japan, 175.
- Chen, R.F. (1967). Removal of fatty acids from serum albumin by charcoal treatment. J. Biol. Chem. 242: 173–181.
- Nascimento, M.M., Pasqual, D.D., dos Santos, J.E., Riella, M.C. (2002). Six years of treatment with the HELP system of a patient with familial hypercholesterolemia. Braz. J. Med. Biol. Res. 35: 775–782.
- Sarnatskaya, V.V., Lindup, W.E., Walther, P., Maslenny, V.N., Yushko, L.A., Sidorenko, A.S., Nikolaev, A.V., Nikolaev, V.G. (2002). Albumin, bilirubin, and activated carbon: New edges of an old triangle. Artif. Cells Blood Substit. Immobil. Biotechnol. 30: 113–126.
- Certificate of the state registration, number 2647/2004 on 26.03.2004.
- Agalou, S., Ahmed, N., Thornalley, P.J., Dawnay, A. (2005). Advanced glycation end product free adducts are cleared by dialysis. Ann. N. Y. Acad. Sci. 1043: 734–739.
- Yang, X.B., Hou, F.F., Wu, Q., Zhou, H., Liu, Z.R., Yang, Y., Zhang, X. (2005). Increased levels of advanced oxidation protein products are associated with atherosclerosis in chronic kidney disease. Zhonghua Nei Ke Za Zhi 44: 342–346.