Abstract
A novel method of preparing collagen/β-tricalcium phosphate microspheres with chitosan as the mechanical strength enhancer has been developed in this study. The process involved firstly droplet formation by discharging a mixture of collagen, β-tricalcium phosphates and alginate into an aqueous solution of CaCl2 by extruding through an air jet-syringe at 4°C. The gel beads thus formed were collected and subsequently coated with chitosan to stabilize the surface of gel bead. Collagen within the gel beads was then reconstituted while the entrapped alginate was liquefied and drained by incubating in phosphate buffer at 37°C. Microspheres comprised of fibrillar collagen and well-dispersed β-tricalcium phosphate particulates were obtained by this process. And the mechanical strength of these microspheres was significantly enhanced by chitosan coating. These chitosan-coated collagen/β-tricalcium phosphate microspheres have an open fibrillar network structure with a great potential for future application as biodegradable bone grafting materials.
INTRODUCTION
Both type I collagen and β-triclacium phosphate (β-TCP) are biodegradable and exhibit osteoconductive property when implanted in the injured site of bone tissue [Citation[1]]. Although the composites of collagen and β-TCP were commonly made as blocks of sheet or sponge, microspheric composite is more versatile in medical applications. Microspheres can be injected to the damaged tissue for repair and are considered less invasive for surgical operation as compared with the block materials.
We have previously developed a method of preparing microspheres comprised of collagen and hydroxyapatite using an emulsion system of water in olive oil [Citation[2]]. These microspheres are excellent carriers for both BMP-2 and osteoblast cells, and have a great potential for bone graft applications [Citation[2], Citation[3]]. The preparation procedure, however, requires extensive purification steps to remove the residual oil from microsphereic surface. An alternative approach employing a concept similar to cell encapsulation with the alginate [Citation[4], Citation[5]] is to discharge a mixture of sodium alginate and monomeric collagen into an aqueous CaCl2 solution followed by the surface coating of the resulted gel beads with poly(lysine). After dissolution of alginate and reconstitution of collagen fibers, microspheres with collagen fibrils dispersed in liquid alginate and encapsulated by the polymeric complex membrane of poly(lysine)/alginate were obtained [Citation[6]]. These microspheres are not degradable in the host tissue and thus could not be used as the biodegradable grafting material for bone repair.
Chitosan is a cationic copolymer of glucosamine and N-acetylglucosamine obtained from chitin, which is a natural polymer derived from the shell of crustaceans. Chitosan are nontoxic, noncarcinogenic, and nonimunogenic. Recently, it has been shown to enhance bone formation [Citation[7]] and to have osteoconductive properties for bone regeneration that combine with calcium phosphates [Citation[8]].
This article describes a novel method using chitosan to stabilize the composite microspheres of collagen and β-TCP. The resulted microspheres have an open network structure on the surface and therefore are applicable for future as implant materials of bone repair.
MATERIALS AND METHODS
Materials
β-tricalcium phosphate (β-TCPs) were purchased from Merck-Schuchardt (Germany). Both alginate and chitosan were purchased from Sigma (St. Louis, MO, USA). The deacetylation degree of chitosan used was approximately 85%. All other chemicals used were reagent grade. Type I collagen was prepared from bovine skin according to the procedures described previously [Citation[9]] and stored in 0.5 M acetic acid at 4°C until use.
Preparation of the Chitosan-Coated Collagen/β-TCP Microspheres
Microspheres were prepared according to the procedure depicted in . Collagen was mixed with β-TCP with a weight ratio of 35:65 (w/w, collagen: β-TCP) in an aqueous solution of sodium alginate (1.2% w/w) at 4°C. Approximately 10 ml of this mixture was dripped into a 100 ml 1.5% (w/v) calcium chloride solution with mild agitation to form gel beads. The gel beads were washed with Ca-CHES buffer (1.1% CaCl2 in 0.1% 2-(N-cyclohexylamino) ethane-sulfuric acid, pH 7.2) for 5 min and then immersed in a solution (pH 5.5) containing 0.05 w/v% chitosan and 0.05% lactic acid for another 5 min. Alginate of the chitosan-coated gel beads were liquefied by incubating in 0.5 M phosphate buffer solution for 5 min at room temperature. The gel beads were then incubated in 0.02 M phosphate solution at 37°C for 24 h for the reconstitution of monomeric collagen into fibrillar network. The concentration of alginate in the solution was measured with a method described previously [Citation[10]]. The microspheres were washed with PBS repeatedly and stored in PBS solution at 4°C.
Microscopic Examination of Microspheres
The microspheres were washed three times with PBS, fixed with glutaraldehyde (2.5% in PBS, pH 7.4) for 1–2 h and then post-fixed in 1% osmium tetroxide in PBS for 1–2 h. The fixed specimens were dehydrated through a series of aqueous solutions containing ethanol with increasing concentration up to 100% (v/v). The dehydrated microspheres were then critical-point dried, sputter coated with gold, and examined under a JSM 5300 scanning electron microscopy (JEOL).
RESULTS AND DISCUSSION
Sizes of Microspheres
The air flow rate of the collagen/β-TCP/alginate droplet generator () is a key parameter for controlling the diameter of alginate gel bead and therefore the sizes of the resulted chitosan-coated collagen/β-TCP microspheres. By increasing the air flow from 5 to 7.5 and 10 ml/min, the average diameter of the microspheres decreases from 1560 ± 70 µm to 835 ± 36 µm and 210 ± 12 µm (n = 300), respectively. Of these, the microspheres with diameters of about 200–300 µm are most useful as cell carriers considering the criteria of injectability for implantation.
Effects of Chitosan Coating and the Removal of Alginate from Gel Beads
Sodium citrate was commonly used to liquefy the intra-capsular alginate when preparing microcapsules of the alginate/poly-lysine system [Citation[5]]. However, in our case, the collagen/β-TCP/alginate gel beads without chitosan coating disintegrated when immersed in citrate buffer. When washed with phosphate buffer, alginate could be removed from the gel matrix but the resulted microspheres were very weak in mechanical strength and often fractured into pieces. Coating of the collagen/β-TCP/ alginate gel beads with chitosan appears to solve these problems. Incubation of the chitosan-coated collagen/β-TCP/alginate gel beads in PBS results in a slow release of alginate from the gel matrix, probably due to the removal of calcium from the gel bead by phosphate. This slower depletion of the alginate is advantageous by preventing the early-stage collapse of microsphere while allowing the collagen reconstitution to take place in the chitosan-coated gel beads. As shown in , only 28% alginate was released into PBS in 1 h, a time frame comparable with the reconstitution of collagen, which is initiated in less than 30 min. Although the release rate is slow, alginate can be almost completely removed from the gel bead after prolonged PBS treatment (). After 12 h of washing, the microspheres contained only ∼3% of the initial alginate.
Figure 2 Removal of alginate from chitosan-coated collagen/β-TCP/alginate gel beads by PBS. The chitosan-coated collagen/b-TCP/alginate gel beads were immersed in PBS 37°C. The amount of the alginate releasing from the gel beads was measured according to the method described by Halle [Citation[10]].
![Figure 2 Removal of alginate from chitosan-coated collagen/β-TCP/alginate gel beads by PBS. The chitosan-coated collagen/b-TCP/alginate gel beads were immersed in PBS 37°C. The amount of the alginate releasing from the gel beads was measured according to the method described by Halle [Citation[10]].](/cms/asset/66bac487-e6c6-4925-9c70-498b9363c808/ianb19_a_237751_f0002_b.gif)
Microstructure of Chitosan-Coated Collagen/β-TCP Microsphere
As observed under optical microscope, the particulates of β-TCP (dark spots due to non-transparent to light) dispersed well in the collagen matrix (). Detailed morphology analysis under scanning electron microscope showed that near the surface of the microsphere, collagen fibers with the sizes of 173 ± 23 nm (n = 40) interpenetrated each other but a large number of collagen fibers appeared to be compressed together. There also exist on the surface a few flat thin pieces of micrometer size, probably comprised of chitosan or alginate/chitosan complex (a). This flake-like structure was nevertheless rarely seen in the microspheric interior section where chitosan was not present. Different from the surface region, the collagen fibrils are smaller in diameter with sizes of 63 ± 10 nm (n = 40). The particles of β-TCP are also seen through the collagen matrix and a few of them associated with bundles of collagen fibers (white dots in b). Although the surface seems to be more dense in fibrillar arrangement, it nevertheless has an open surface network structure with some of the pores larger than 0.4 µm to 1.2 µm, capable of free transport of macromolecules with its surroundings.
The Importance of Chitosan Coating
Chitosan coated on the surface of collagen/β-TCP/alginate gel beads plays two important roles for the fabrication of microspheres. It stabilizes the gel beads during alginate extraction by PBS and additionally strengthens the resulted collagen/β-TCP microspheres. As shown in , microspheres obtained from the process without chitosan coating were weak and collapsed when placed on the glass slide (, right). On the other hand, the chitosan-coated collagen/β-TCP microspheres are stronger, although compressed to flat on the contact side with glass (, left), but capable of fully restoring their spheric shape when re-immersed in PBS.
Figure 5 Optical micrographs of the microspheres on the glass slide: (left) microspheres with chitosan coating; (right) a microspheres without chitosan coating.
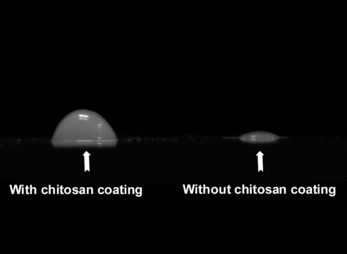
In this study, chitosan—a positive charged polysaccharide—was employed to replace poly(lysine) as the coating material of the alginate-containing micro gel beads. If poly(lysine) were to be used in this study, we will end up with a microcapsule, which provides immunoisolation but became non-biodegradable. The macroporous structure on the surface of these microspheres provides accessible routes with cells, macromolecules and nutrients in the surrounding environment. Therefore, the microspheres developed in this study could be used for cell carriers or biodegradable biomaterials. The use of chitosan is especially beneficial for bone graft application since chitosan has been reported to possess osteoconductive property.
This work has been supported in part by a grant from NHRI (NHRI-EX94 & 95-9322EI), Taiwan, ROC.
REFERENCES
- Grimes, J.S., Bocklage, T.J., Pitcher, J.D. (2006). Collagen and biphasic calcium phosphate bone graft in large osseous defects. Orthopedics 29: 145–148.
- Wu, T.J., Huang, H.H., Lan, C.W., Lin, C.H., Hsu, F.Y., Wang, Y.J. (2004). Studies on the microspheres comprised of reconstituted collagen and hydroxyapatite. Biomaterials 25: 651–658.
- Wang, Y.J., Lin, F.H., Sun, J.S., Huang, Y.C., Chueh, S.C., Hsu, F.Y. (2003). Collagen-hydroxyapatite microspheres is a good carrier for bone morphogenic protein-4. Artif. Organs. 27: 161–167.
- Lim, F., Sun, A. (1980). Microencapsulated islets as bioartificial endocrine pancreas. Science 20: 908–910.
- Wong, H., Chang, T.M. (1986). Bioartificial liver: Implanted artificial cells microencapsulated living hepatocytes increases survival of liver failure rats. Int. J. Artif. Organs. 9: 335–336.
- Hsu, F.Y., Tsai, S.W., Wang, F.F., Wang, Y.J. (2000). The collagen-containing alginate/poly(L-lysine)/alginate microcapsules. Art. Cells, Blood Subs., and Biotech. 28: 147–154.
- Mukherjee, D.P., Tunkle, A.S., Roberts, R.A., Clavenna, A., Rogers S., Smith, D. (2003). An animal evaluation of a paste of chitosan glutamate and hydroxyapatite as a synthetic bone graft materials. J. Biomed. Mater. Res. B Appl. Biomater. 67: 603–609.
- Lee, J.Y., Seol, Y.J., Kim, K.H., Lee, Y.M., Park, Y.J., Rhyu, J.C., Chung, C.P., Lee, S.J. (2004). Transforming growth factor (TGF)-beta1 releasing tricalcium phosphate/chitosan microgranules as bone substitutes. Pharm. Res. 21: 1790–1796.
- Len, C.H., Wang, F.F., Wang, Y.J. (2003). Osteogenic enrichment of bone-marrow stromal cells with the use of flow chamber and type I collagen-coated surface. J. Biomed. Mater. Res. 66A: 38–46.
- Halle, J.P., Landry, D., Fournier, A., Beaudry, M., Leblond, A. (1993). Method for the quantification of alginate in microcapsules. Cell Transplant. 2: 429–436.