Abstract
In this study, uricase was immobilized by a glutaraldehyde/gelatine crosslinking procedure onto polypyrrole film. The Km value for immobilized enzyme 0.44 mM was much higher than that of the free enzyme 0.39 mM. Vmax values were 8.4 × 10−2 mM/dak and 7.1 × 10−2 mM/dak for free and immobilized enzyme, respectively. The optimal pH values for free and immobilized enzymes were 8.5 and 8.0, respectively. The optimum temperature for both free and immobilized uricase was 35°C and 55°C. The enzyme activity after storage for 7 weeks was found to be 42% and 49% of the initial activity values for free and immobilized enzymes, respectively. The amperometric current obtained after 30 measurements at a constant uric acid concentration of 5.0 × 10−5 M was found to be 77.7% of initial activity.
INTRODUCTION
Immobilization of enzymes on the electrode surface is a key factor in fabricating a biosensor and various immobilization methods have been employed in preparation of reagentless amperometric enzyme electrodes [Citation[1]].
Many methods have been described for the attachment of enzymes to supporting structures. The activity of an immobilized enzyme depends on a number of factors, which can be summarized as size and shape of the carrier material, the nature of the immobilization method, the composition of the carrier material and the specific conditions during immobilization. The success of an immobilized enzyme for practical applications depends strongly on the properties of the carriers employed [Citation[2]].
Among the numerous advances reported in this field, the immobilization of enzymes on electrodes in the design and optimization of biosensors has attracted great interest. The conventional techniques for enzyme immobilization include entrapment by ion-exchange polymers [Citation[3]], conducting polymers, and nonconducting polymers [Citation[4]], sol–gels [Citation[5]], and cross-linking in bovine serum albumin–glutaraldehyde [Citation[6]].
In recent years, mediators and conducting polymers have been used as a matrix for immobilizing the enzymes [Citation[7], Citation[8]]. The mediators are also known to have an important role in biosensors [Citation[9]]. Intrinsically conducting polymers with conjugated double bonds have been viewed as attractive advanced materials for electronic devices [Citation[10]], electrochromic displays [Citation[11]], chemical and biochemical sensors [Citation[12]], drug release systems, rechargeable batteries [Citation[13]] and for modifying electrode surfaces for electrical wiring of biomolecules [Citation[14]]. Among the conducting polymers used for the immobilization of biomolecules, for example, polyacetylene, polythiophene, polypyrrole (PPY), polyindole and polyaniline [Citation[15], Citation[16]].
Glutaraldehyde has been extensively used as an enzyme immobilizing agent for many years [Citation[17-19]]. Although there are many discussions on the nature of the solution species and structures responsible for its properties, it is generally accepted that reactions between the carbonyl group of glutaraldehyde and the amino functions of the enzyme take place yielding a Schiff base [Citation[20], Citation[21]].
The electrochemical immobilization of the enzyme together with the pyrrole and glutaraldehyde may increase the useful lifetime of the enzyme electrode by formation of cross-linkages between the functional reagent and amino groups from enzyme into the conducting polymer [Citation[22]].
The native enzyme has a relative molecular mass of 124,000 and consists of four identical or similar-sized subunits of 31,000 each [Citation[23]].
Uricase, which is denoted as Uox, catalyzer in vivo oxidation of uric acid in the presence of oxygen as an oxidizing agent, producing allantoin and CO2 as oxidation products of uric acid and hydrogen peroxide as a reduction of O2.
The amperometric detection of uric acid can be made by electrochemical oxidizing the produced H2O2.
In this study, uricase was immobilized by a glutaraldehyde/gelatine crosslinking procedure onto polypyrrole film. Effects of the immobilization process on enzyme activity, kinetic parameters, storage and reuse capability of the enzyme were investigated.
MATERIAL AND METHODS
Equipment and Reagents
The electrochemical studies were carried out using Epsilon EC electrochemical analyzer using a three-electrode cell. The working electrode was a Pt plate (0.5 cm2). The auxiliary and the reference electrodes were a Pt wire and Ag/AgCl, respectively. The pH values of the buffer solutions are measured with ORION Model 720 A pH/ionmeter. Temperature control was achieved with Grant W14 thermostat.
Uricase (EC 1.7.3.3, purified from the microorganism and with an activity of 10 unit·ml−1) and uric acid were purchased from Sigma. Pyrrole and ferrocene was supplied from Fluka, tetrabutylammonium tetraflouroborate from Aldrich and acetonitrile from Merck. All other chemicals were obtained from Sigma. All the solutions were prepared by the use of bi distilled water.
Preparation of Pt/PPy-Fc Film Electrode
The electrode was immersed in a 5 mL solution of 0.2 M pyrrole, 0.1 M tetrabutylammonium tetraflouroborate, 10 mM ferrocene in acetonitrile [Citation[24]]. The solution was purged with nitrogen in order to remove the oxygen. The electropolymerization of pyrrole upon the electrode surface was achieved by the cyclic voltammetric scans between 0.0 and + 1.0 V at a scan rate of 50 mV/s. The electrode was washed with acetonitrile and then buffer solution after the coating process.
İmmobilizaton of Uricase on Pt/PPy-Fc Film Electrode
50 µL uricase enzyme (10 Unit/mL) was dropped upon each other surface of platinium/polypyrrole-ferrocenium (Pt/PPy-Fc) electrode. The film was taken out of the enzyme solution on the remaining enzyme, solution was completely wiped off from the film and it was dried under ambient conditions. Then, 100 µL/cm2 of 5 mg/ml gelatin solution (prepared in water and incubated 30 min at 37°C before use) was uniformly spread over the enzyme adsorbed Pt/PPy-Fc electrode dried at room temperature. This electrode was then dipped into a glutaraldehyde solution (1.25% in water) for 30 s for crosslinking and washed immediately with plenty of borate buffer and dried at room temperature for severel hours [Citation[25]]. İmmobilized enzyme electrode was kept in a refrigerator at the in borate buffer when it was not in use.
Effect of Glutaraldehyde on the Enzyme Activity
The effect of the amount of glutaraldehyde on the enzyme activity was evaluated. For this purpose, uricase was immobilized using crosslinking procedure. Then, different amounts of glutaraldehyde solution were prepared with dilution glutaraldehyde solution of 25% (v/v). Preparing film electrodes were dipped in a known period glutaraldehyde solution of 5%, 2.5%, 1.25%, 0.625% (v/v), respectively. Then, amperometric response currents were measured, showing a significant increase in the signal when 1.25% (v/v) of glutaraldehyde and 30 s period were employed in the enzyme electrode. The use of higher amounts of this chemical didn't improve the response for the immobilized uricase, which exhibited a decrease in the signal, probably due to the increase in the PPY film thickness, making the diffusion of the electroactive species through the electrode more difficult. Immobilized uricase useful lifetime was decreased when glutaraldehyde concentrations from 1.25 to 5% (v/v) were employed. As is well known, glutaraldehyde dissolved in aqueous solution is polymerized to give several kinds of polymers, the degree being higher for the higher the glutaraldehyde concentration [Citation[26]]. If the amount of the polymerized glutaraldehyde molecules not involved in the cross linking reactions increases in the resulting UOx network, they might disturb diffusion of uric acid. For all studies a glutaraldehyde concentration of 1.25% (v/v) was chosen for enzyme immobilization, where the immobilized uricase showed the highest response and a good lifetime.
Enzyme Assay
The immobilized and free uricase activities were determined by amperometric measurement. Solution containing free or immobilized enzyme 5 mL in aqueous buffer solution containing 0.05 M borate was added to the cell. The solution of steady-state background current (ia) was allowed to decay at a constant value at + 0.7 V(Ag/AgCl). Then the aliquots uric acid stock solutions were added to the cell using a micropipette, and stirred for 10 min. Final concentration of uric acid was 5·10−5 M in the cell. The current (ib) of uric acid solutions was measured after 400 s following the application of a constant potential of + 0.7 v (vs Ag/AgCl). The current values (ΔI = ib − ia) were plotted.
Scanning Electron Microscopy (SEM) Studies
In these experiments, Scanning Electron Microscope JEOL Model 6360 instrument was used.
RESULTS AND DISCUSSION
SEM of Pt/Polypyrrole-Ferrocenium Film
It was shown that SEM of Pt/PPy-Fc film resembled a cauliflower-like structure (). This state gives proof of cover with polypyrrole of surface.
SEM of Pt/Polypyrrole-Ferrocenium-Uricase
SEM picture of Pt/PPy-Fc Uricase is shown in . It was shown that SEM picture of Pt/Polypyrrole-Ferrocenium-Uricase has a different shape needle crystal. This picture, compared with , shows that the cauliflower-like structure has changed. This state was attributed to interaction with uricase of the polypyrrole structures. Needle crystals are shown in instead of the cauliflower-like structure in . This state was attributed to belong to the uricase of the needle crystals.
SEM of Pt/Polypyrrole-Ferrocenium-Uricase-Gelatine
SEM picture of Pt/PPy-Fc-Uricase-Gelatine is shown in . This picture, compared with the preceeding picture, shows the change of the needle crystal structure. The surface is covered with gelatine.
SEM of Pt/Polypyrrole-Ferrocenium-Uricase-Gelatine-Glutaraldehyde
SEM picture of Pt/PPy-Fc-Uricase-Gelatine-Glutaraldehyde is shown in . It is seen to change the shape of the surface. This state can be attributed to the cross linking of uricase and gelatine with glutaraldehyde.
Effect of pH
Optimum pH for free and immobilized uricase was determined by measuring the activity of free and immobilized enzymes in buffers of different pH values ranging from pH 6.5–9.5 at 25°C. The effect of pH on the activity of free and immobilized uricase is given in . The current is enhanced by increasing the solution pH in the range of pH 6.5 to 8.5 for free enzyme at a constant uric acid concentration of 5.0 × 10−5 M. The maximum response current was observed at pH 8.5 for free enzyme. These values are similar with literature values [Citation[23], Citation[27], Citation[28]]. The amperometric response is enhanced by increasing the solution pH in the range of pH 6.5 to 8.0 for immobilized enzyme at a constant uric acid concentration of 5.0 × 10−5 M. The maximum response current was observed at pH 8.0 for immobilized enzyme [Citation[25]]. These values are similar to those of the solution phase enzyme [Citation[29-31]]. Shifts in pH optimum with immobilization have been found for many enzymes. Anionic supports tend to shift the pH optimum toward the alkaline side; cationic supports tend to shift the pH optimum toward the acidic side. This was attributed to the fact that the used polymer and the type of immobilization were different [Citation[32]].
Effect of Temperature
The effects of temperature on the activity of free and immobilized uricase are given in . In case of temperature studies, free and immobilized enzymes were incubated in the reaction mixtures at different temperatures ranging from 20 to 70°C. The activities of free and immobilized enzyme were plotted against respective temperature.
The response current increased gradually with temperature (), and free enzyme reached a maximum value at 35°C while the maximum level of immobilized enzyme was found at 55°C [Citation[25]]. Over these values, a sharp decrease in the enzyme electrode response was observed, probably due to the enzyme thermal denaturation, as has also been reported by others authors [Citation[33]]. The activity of both free and immobilized uricase was shown to be strongly dependent on the temperature. But when the temperature is too high, the enzyme will easily be denatured and lose its enzymatic activity. Although the response was highest at 55°C, for practical reasons room temperature (25°C) is recommended to be used in order to prolong the lifetime of the immobilized enzyme since most enzymes can easily be denatured at high temperature. If comparing with the optimum temperature of free uricase, which has been reported to be 30°C, it seems that the immobilizing support stabilizes to the enzyme, becoming less susceptible to the temperature-induced conformational changes [Citation[34]].
The Effect of Substrate Concentration on Enzyme Activity
When a biocatalyst is immobilized, kinetic parameters Km and Vmax undergo variations with respect to the corresponding parameters of the free form, revealing an affinity change for the substrate. These variations are attributed to several factors, such as protein conformational changes induced by the support, steric hindrances and diffusional effects. These factors may operate simultaneously or separately, alternating the microenvironment around the bound enzyme. The effect of immobilization on kinetic parameters was studied by measuring the rates of uricase reaction by free and immobilized uricase at various concentrations of uric acid. The Lineweaver–Burk plot for the free and immobilized uricase is given in . The Km and Vmax values were calculated from the slope and intercept of the straight lines, respectively. The Km constant, a specific parameter of enzymes, was computed by the use of 1/[uric acid]) − 1/V graph (Lineweaver–Burke plot). Km and Vmax values were found as 0.39 mM, 8, 4·10−2 mM/minute and 0.44 mM, 7.1 mM/minute [Citation[25]], respectively, for free and immobilized enzyme. The apparent Km value of free uricase was found to be lower than that of immobilized uricase. The Km value, which shows the affinity of the enzyme electrode, was 0.44 mM and the values cited in literature were 0.088 mM, 0.170 mM and 0.90 mM, 3.80 mM [Citation[30], Citation[35], Citation[36]]. Since the enzymatic reactions take place through a carrier medium the affinity of the enzyme towards the substrate is not the same in every carrier media. This increase in apparent Km value might be either due to structural changes in the enzyme induced by the applied immobilization procedure or due to the lower accessibility of the substrate to the active site of the immobilized enzyme. This higher Km value can be attributed to the diffusional resistance of the carrier against substrate and/or product and less porous structure of the membrane [Citation[37]].
Figure 7 The effect of uric acid concentration upon the amperometric response of free and immobilized uricase (Lineweaver-Burk Plot).
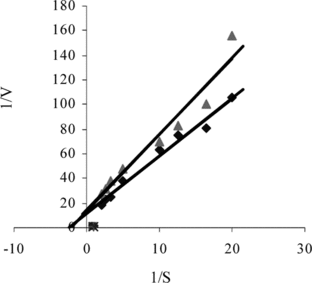
The decrease in Vmax value as a result of immobilization should be related with the increase in Km value, since an increase in the Km value leads to a decrease in the affinity of the enzyme for its substrate [Citation[38]].
Repeated Use Capability
The ability of the immobilized enzyme layer to retain activity after repeated analyte exposure is important for reusability. In order to test the reproducibility of the enzyme electrode prepared, the current changes obtained after subsequent usage were plotted against the number of measurements. The amperometric current obtained after 30 measurements at a constant uric acid concentration of 5.0 × 10−5 M was found to be 77.7% of initial activity. The activity of immobilized enzyme after repeated use is given in [Citation[25]]. This shows that the reproducibility of the uric acid electrode was highly satisfactory. This improved stability of immobilized uricase can be attributed to the improved resistance to denaturation and conformational changes of the enzyme in buffer solution, as a result of the crosslinking procedure of the uricase molecules to film electrode.
Storage Stability
Another issue concerning the practical use of enzyme is the storage stability, or the ability of the immobilized enzyme to retain activity over a long period of time. Prepared immobilized enzyme retained 49% [Citation[25]] of its original activity after 7 weeks of storage, showing good activity preservation. Free enzyme retained 42% of its original activity after 7 weeks of storage, showing good activity preservation.
There was no noticeable decrease in the response during the first five weeks. There was a rapid decrease in current values between the fifth and seventh weeks. İmmobilized enzyme showed 49% of the initial amperometric response at the end of the seventh week. This indicates that the immobilized enzyme prepared can be used for quite a long time.
The properties and the optimum working conditions of the immobilized enzyme and free enzyme are summarized in .
Table 1. The properties and the optimum working conditions of immobilized enzyme and free enzyme
CONCLUSION
In conclusion, uricase was immobilized onto polypyrrole film for this study, demonstrating:
It gives highly reproducible results (the immobilized enzyme maintains 77.7% of the initial amperometric response at the end of the 30th measurement).
It has a satisfactory storage stabilization (the immobilized enzyme and free enzyme maintains 49% and 42% of the initial amperometric response at the end of the 7th week, respectively).
The Km and Vmax values of free and immobilized uricase in polypyrrole -ferrocene film were 0.39, 0.44 mM and 7.1 × 10−2 mM/minute, 8.4 × 10−2 mM/minute, respectively.
We acknowledge the support of this project by Gazi University Research Fund (FEF 05/2002-28).
REFERENCES
- Cosnier, S., Szunerits, S., Marks, R.S., Novoa, A., Puech, K., Perez, E., Rico-Lattes, I. (2001). A comparative physical study of two different hydrophilic synthetic latex matrices for the construction of a glucose biosensor. Talanta. 55: 889–897.
- Hasselberger, F.X., Allen, B., Paruchuri, E. K., Charles, M., Coughlin, R.W. (1974). Immobilized enzymes Lactase bonded to stainless steel and other dense carriers for use in fluidized bed reactors. Biochemical and Biophysical Research Communications 57: 1054–1062.
- Mizutani, F., Yabuki, S., Katsura, T. (1993). Amperometric enzyme electrode with fast response to glucose using a layer of lipid-modified glucose oxidase and Nafion anionic polymer. Anal. Chim. Acta. 274: 201–207.
- Castillo-Ortega, M.M., Rodriguez, D.E., Encinas, J.C., Plascencia, M., Mendez-Velarde, F.A., Olayo, R. (2002). Conductometric uric acid and urea biosensor prepared from electroconductive polyaniline-poly(n-butyl methacrylate) composites. Sensors and Actuators. B 85: 19–25.
- Liu, Z.J., Liu, B.H., Kong, J.L., Deng, J.Q. (2000). Probing trace phenols based on mediator-free alumina sol-gel derived tyrosinase biosensor. Anal. Chem. 72: 4707–4712.
- Jin, L.T., Ye, J.S., Tong, W., Fang, Y.Z. (1993). A study of biosensors based on a glassy – carbon electrode modιfιed with nafion and methyl violegen. Mikrochimica Acta. 112: 71–75.
- Gerard, M., Chavbev, A., Malhotra, B.D. (2002). Application of conducting polymers to biosensors. Biosensors and Bioelectronics 17: 345–359.
- Reiter, S., Habermüller, K., Shuhman, W. (2001). A reagentless glucosebiosensor based on glucose entrapped into osmium-complex modified polypyrrole films. Sensors and Actuators B. 79: 150–156.
- Chavbey, A., Malhotra, B.D. (2002). Mediated biosensors. Biosens. Bioelectron. 17: 441–456.
- Potember, R.S., Hoffmann, R.C., Hu, H.S., Cocchiaro, J.E., Viands, C.V., Murphy, R.A., Poehler, T.O. (1987). Conducting organics and polymers for electronic and optical devices. Polymer. 28: 574–580.
- Skotheim, T.A. (1986). Handbook of Conducting Polymers, Marcel Dekker: New York, 1: pp. 673–687.
- Sadik, O.A. (1999). Bioaffinity sensors based on conducting polymers: a short review. Electroanalysis 11: 839–844.
- Bidan, G. (1992). Electroconducting conjugated polymers: new sensitive matrices to build up chemical or electrochemical sensors. Sensors and Actuators B 6: 45–56.
- Garnier, F., Youssoufi, H.K. (1994). Enzyme recognition by polypyrrole functionalized with bioactive peptides. J. Am. Chem. Soc. 116: 8813–8814.
- Shinohara, H., Chiba, T., Aizawa, M. (1988). Enzyme microsensor for glucose with an electrochemically synthesized enzyme polyaniline film. Sensors and Actuators. 13: 79–86.
- Bartlett, P.N., Cooper, J.M. (1993). A review of the immobilization of enzymes in electropolymerized films. J. Electroanal. Chem. 363: 1–12.
- Cass, A.E.G. (1990). Biosensors. A Practical Approach, IRL Press: New York.
- Kuwabata, S., Okamoto, T., Kajiya, Y., Yoneyama, H. (1995). Preparation and amperometri glucose sensιtιvιty of covalently bound glucose-oxιdase to (2-aminoethyl)ferrocene on an Au electrode. Anal. Chem. 67: 1684–1690.
- Khan, G.F., Wernet, W. (1996). Platinization of shapable electroconductive polymer film for an improved glucose sensor. J. Electrochem. Soc. 143: 3336–3342.
- Margel, S. (1984). Characterization and chemistry of polyaldehyde microspheres. Science. Polym. Chem. Ed. 22: 3521–3533.
- Walt, D.R. (1994). Again, the chemistry of enzyme and protein immobilization with glutaraldehyde. Trac-Trends Anal. Chem. 13: 425–430.
- Junior, L.R., Neto, G.O., Fernandes, J.R., Kubota, L.T. (2000). Determination of salicylate in blood serum using an amperometric biosensor based on salicylate hydroxylase immobilized in a polypyrrole–glutaraldehyde matrix. Talanta. 51: 547–557.
- Alamillo, J.M., Cárdenas, J., Pineda, M. (1991). Purification and molecular properties of urate oxidase from Chlamydomonas reinhardtii. Biochimica et Biophysica Acta. 1076: 203–208.
- Arslan, F., Yaşar, A., Kiliç, E. (2006). Preparation of Pt/polypyrrole–ferrocene hydrogen peroxide sensitive electrode for the use as a biosensor. Russian Journal of Electrochemistry 42: 137–140.
- Çete, S., Yaşar, A., Arslan, F. (2006). An amperometric biosensor for uric acid determination prepared from uricase immobilized in polypyrrole film. Artifical Cells, Blood Substituted, and Biotechnology 34: 367–380.
- Kuwabata, S., Nakaminami, T., Ito, S., Yoneyama, H. (1998). Preparation and properties of amperometric uric acid sensors. Sensors and Actuators B 52: 72–77.
- Montalbini, P., Redondo, J., Cabellero, J.L., Cardenas, J., Pineda, M. (1997). Uricase from leaves: Its purification and characterization from three different higher plants. Planta. 202: 277–283.
- Jianguo, L., Gaoxιang, L., Hong, L., Xiukai, Z. (1994). Purification and properties of uricase from candida sp. and its application in uric acid analysis in serum. Applied Biochemistry and Biotechnology 47: 57–63.
- Uchiyama, S., Sakamoto, H. (1997). İmmobilization of uricase to gas diffusion carbon felt by electropolymerization of aniline and its application as an enzyme reactor for uric acid sensor. Talanta. 44: 1435–1439.
- Nakamura, N., Murayama, K., Kinoshita, T. (1986). Immobilization of uricase on protamine bound to glass beads and its application to determination of uric acid. Analytical. Biochemistry. 152: 386–390.
- Akyιlmaz, E., Sezgintürk, M.K., Dinçkaya, E. (2003). A biosensor based on urate oxidase-peroxidase coupled enzyme system for üric acid determination in urine. Talanta. 61: 73–79.
- Telefoncu, A. (1999). Biyosensörler, Biyokimya Lisansüstü Yaz Okulu, Haziran 20–26 Ege Üniversitesi: Kuşadasι, pp. 136–137.
- Haouz, A., Glandieres, J.M., Alpert, B. (2001). Involvement of protein dynamics in enzyme stability. The case of glucose oxidase. FEBS Lett. 506: 216–220.
- Kang, L.Y., Neoh, E.T. (2002). Covalent immobilization of glucose oxidase on microporous membranes prepared from poly(vinylidene fluoride) with grafted poly (acrylic acid) side chains. J. Membr. Sci. 208: 361–374.
- Kuwabata, S., Nakaminami, T., Ito, S., Yoneyama, H. (1998). Preparation and properties of amperometric uric acid sensors. Sensors and Actuators B52: 72–77.
- Gasper, S., Habermüller, K., Csöregi, E., Schuhmann, W. (2001). Hydrogen peroxide sensitive biosensors based on plant peroxidases entrapped in Os-modified polypyrrole films. Sensors. Actuat. B72: 63–68.
- Karim, M.R., Hashinaga, F. (2002). Preparation and properties of immobilized pummelo limonoid glucosyltransferase. Process Biochemistry 38: 809–814.
- Gürsel, A., Alkan, S., Toppare, L., Yağcι, Y. (2003). Immobilization of invertase and glucose oxidase in conducting H-type polysiloxane/polypyrrole block copolymers. Reactive Functional Polymers 57: 57–65.