Abstract
Direct conjugation of bovine hemoglobin (Hb) and human serum albumin (HSA) with glutaraldehyde would result in a complex mixture of dimers Hb-Hb, Hb-HSA, HSA-HSA and other oligomers. To obtain a high yield of target Hb-HSA, modulation of the reaction environment was carried out. It was found that polyethylene glycol (PEG), a hydrophilic polymer, could improve the yield of Hb-HSA conjugate. The degree of improvement depended on the molecular weight and concentration of PEG. Under optimum condition of 9% (w/v) of PEG 4000, the reaction proceeded in a controlled mode with conversion yield of starting proteins to Hb-HSA increasing from 6% to 30%. The purity was about 88% of the total conjugates. Furthermore, the impurities were mainly tetrameric molecules of two Hb-HSA conjugates. The improvement could be attributed to the “micro-compartment” created by addition of polyethylene glycol, which brings HSA and Hb close together, thus increasing the chance of conjugation between the two molecules.
INTRODUCTION
Hemoglobin (Hb) is a molecule used as a starting material for blood substitutes [Citation[1]]. Due to its short circulation time and nephritic toxicity, much effort has been done in the modification of hemoglobin for the past decades. Recently, the conjugate of bovine hemoglobin and human serum albumin (HSA) has been proposed as a candidate blood substitute [Citation[2]]. The component proteins, albumin and hemoglobin, are the main functional composition of serum and red cells, respectively. Therefore, the conjugate is speculated to possess the volume-expanding function of serum and the oxygen-carrying function of red cells at the same time.
However, direct conjugation of bovine hemoglobin (Hb) and human serum albumin (HSA) normally results in the heterogeneous products consisting of dimers of Hb-Hb, Hb-HSA, HSA-HSA and other polymers, which were unacceptable, especially in therapeutic use. Two methods have been developed to ensure the consistency of the product. One of them utilized the expensive heterobifunctional crosslinker with two different functional groups to react with the reactant proteins specifically [Citation[3]]. In contrast, homobifunctional crosslinkers like glutaraldehyde have two identical reactive ends to couple the reactant proteins. This kind of cross-linker can be easily prepared, but it suffers from the identity of its two reactive ends, which always give rise to a broad range of poorly defined conjugates, as the early trial of Bonhard to conjugate hemoglobin and albumin [Citation[4]]. Thus, another solid-phase conjugation method was developed by Hu and Su [Citation[5]] to overcome this problem, in which albumin was first adsorbed by the solid phase and then reacted with glutaraldehyde to obtain mainly monomeric activated albumin by the spacing-out function of the solid phase, and then the activated albumin was collected and further coupled with hemoglobin. However, both methods were either time-consuming or expensive. Here, a novel method is proposed to conjugate Hb and HSA with homobifunctional reagent glutaraldehyde via regulating of the physical environment of the reactant proteins. Polyethylene glycol (PEG) was used as the modulation agent. The effect of the concentration and molecular weight of PEG on the conjugation reaction was investigated. In the presence of 9% (w/v) PEG 4000, the conversion rate of starting proteins to target Hb-HSA conjugate in the ratio of 1:1 was about 30%, compared with a conversion rate of 6% under the condition without PEG. Meanwhile, the content of byproducts was not increased. Therefore, conjugation between Hb and HSA was specifically improved by PEG. Moreover, the byproduct was found to be the tetramer consisting of two target Hb-HSA conjugates, instead of the common complex mixture composed of Hb-Hb dimer, HSA-HSA dimer and other conjugates.
MATERIALS AND METHODS
Proteins and Reagents
Human serum albumin (fraction V) and glutaraldehyde (50% v/v, Grade I) were obtained from Sigma. All other chemicals were analytical reagent grade. Water used in the experiments was prepared with a RiOs ultra-pure water system (Millipore, Bedford, MA).
Hemoglobin Preparations
Bovine blood erythrocytes were obtained freshly from a local slaughterhouse, followed by osmotic hemolysis and stroma removal with centrifugation at 30,000 g for 30 min. The stroma-free Hb solutions were prepared by ion exchange chromatography [Citation[6]].
Preparation of the Conjugate
HSA and Hb were dissolved in 20 mM sodium acetate buffer, pH 5.7. The final concentration was 2 mg/ml for HSA and 2 mg/ml for Hb. The proteins were mixed with glutaraldehyde (GA) at room temperature for 2 h in a molar ratio of 1:30 protein to GA. Polyethylene glycol powder with different molecular weight was added into the reaction system with concentrations varying from 3% to 9% (w/v).
Analysis of the Conjugate
Samples of the reaction was taken and applied to a TSK-GEL G3000SW column (Toyo Soda, Tokyo, Japan), mounted on a Waters HPLC system. The column was eluted by 50 mM phosphate buffer containing 0.1 M Na2SO4 (pH 7.0) at the flow rate of 0.5 ml/min and monitored at dual wave lengths of 280 nm and 405 nm. (hemoglobin has special absorbance at 405 nm). Data were collected using a Waters 2996 integrator and relative areas of peaks were calculated by integration.
Measurement of the Molecular Weight
TSK-GEL G3000SW column was mounted on an Agilent 1100 HPLC system and connected with the multi-angle laser light scattering (MALLS, Wyatt Technology) detector. The MALLS instrument was calibrated by standard monomer HSA. Astra V.4.9 software was applied to calculate the molecular weights.
SDS-PAGE
Based on the method of Laemmli [Citation[7]], the samples were diluted with one volume of SDS sample buffer containing 0.1 M dithiothreitol and then boiled for 5 min, followed by electrophoresis for 1 h at 200 V. The gel was stained in Coomassie Blue R-250 and then destained.
Isoelectric Focusing (IEF)
IEF was performed as described by Evans et al. [Citation[8]] on PhastSystem (Pharmacia LKB). The pH range was achieved by mixing ampholytes (pH 3–9.5 and pH 4–6) in the ratio 4:1. The gel was stained in Coomassie Blue R-250 and then destained.
Oxygen Equilibrium Binding Measurements
O2 equilibrium curves were measured on a Hemox Analyzer (TCS, PA) at 37°, using the Hemox buffer (pH 7.4). The O2 pressure was measured by a Clark O2 electrode (Yellow Springs Instrument, OH) and simultaneously the Hb saturation was calculated via a dual-wave length spectrophotometer. Values for P50 (the O2 pressure at which Hb is half saturated) are obtained from the O2 equilibrium curves. The Hill coefficient is calculated from the Hill plot (log Y/(1 − Y) vs. log P) where Y is fractional saturation of the Hb with O2 and P is the O2 pressure in mm Hg.
RESULTS
Identification of the Target Conjugate of Hb and HSA in 1:1 Ratio
The identification of the target conjugate of Hb and HSA in 1:1 ratio is very difficult, due to the similarity between the target conjugate and the byproducts, which may comprise Hb-Hb dimer, HSA-HSA dimer, and other polymers with similar charge and molecular weight. Therefore, we used combination methods of high performance size exclusion chromatography (SE-HPLC), SDS-PAGE and MALLS to identify different species.
First, native HSA and Hb were characterized by SE-HPLC ( and ). As shown in , the monomer HSA was eluted at 15.7 min, while the HSA-HSA dimer and HSA aggregate at 13.6 min and 9.3 min, respectively (co-existence of a little quantity of HSA-HSA dimer and aggregate with the HSA monomer is a physical character of HSA). As shown in , the native Hb was eluted at 18 min. However, after being treated with glutaraldehyde (shown in ), Hb-Hb dimer was formed and eluted at 15.4 min, while the intra-crosslinked Hb was eluted at 17.4 min with short retention time compared with native Hb. It is noteworthy that the Hb-Hb dimer () and native HSA () have the approximate elution time; however, the Hb-Hb dimer has much higher absorption at 405 nm than that of native HSA. Therefore, based on the elution behavior of the native HSA, native Hb, intra-crosslinked Hb, HSA-HSA dimer and Hb-Hb dimer, the target conjugate of HSA and Hb in 1:1 ratio is speculated to be eluted at about 14.5 min between those of HSA-HSA dimer at 13.6 min and Hb-Hb dimer at 15.4 min.
Figure 1 The SE-HPLC profile of the native HSA to detect the elution times of the native HSA, HSA-HSA dimer, and HSA aggregate. The real line and the dashed line showed the adsorbance at 280 nm and 405 nm, respectively.
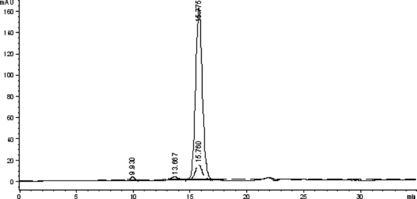
Figure 2 The SE-HPLC profile of the native Hb to detect the elution times of the native Hb and Hb-Hb dimer. The real line and the dashed line showed the absorbance at 280 nm and 405 nm, respectively.
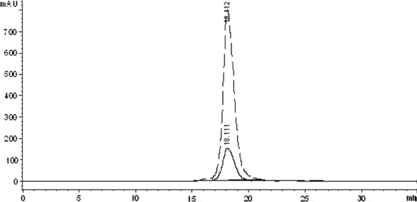
Figure 3 The SE-HPLC profile of the Hb treated by glutaraldehyde to detect the elution time of the intra-crosslinked Hb and inter-crosslinked Hb-Hb dimer. The real line and the dashed line showed the adsorbance at 280 nm and 405 nm, respectively.
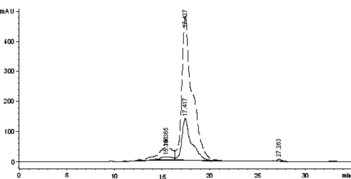
SDS-PAGE was used to detect the covalent linkage of HSA or the subunits of Hb (shown in ). Lane 1 indicated that after being treated with glutaraldehyde, the band of crosslinked HSA-HSA dimer with the molecular weight of about 130 kDa appeared, while the band of covalently crosslinked subunits of Hb with molecular weight of about 30 kDa appeared (as shown in lane 3). Therefore, based on the SDS-PAGE profile of the native HSA, native Hb, intra-crosslinked Hb, HSA-HSA dimer, and Hb subunits covalently crosslinked, the band of the target conjugate of HSA and Hb in 1:1 ratio is speculated to appear with the molecular weight of about 80 kDa and 96 kDa between those of native HSA and HSA-HSA dimer.
Optimization of the Reaction Condition in the Absence of PEG
The conjugation reaction in the absence of PEG was optimized. The conjugation reaction was obviously affected by pH value (shown in ). The results demonstrated that the byproducts could not be inhibited via condition optimization. Furthermore, the pH value between the pI of HSA and that of Hb promoted the specific conjugation, probably as a result of electrostatic interaction of the reactant proteins. Therefore, the average pH value (5.7) between the pI of HSA (4.7) and that of Hb (6.8) was chosen as the working pH. Under the optimum condition, the conversion rate of the starting proteins to the target conjugate was about 6%, while the conversion rate to the byproducts was 5%. The chromatogram is shown in b.
Figure 6 The effect of pH value on the content of conjugate or byproducts in the conjugation reaction. The conjugation reaction was carried out at 4°C for 1 h under different pH values, and the reaction was stopped by addition of excessive lysine solution. The components were determined by TSK G3000 column. The yields of the byproducts and target 1:1 Hb-HSA conjugate were estimated by integrating the chromatograms of the reaction products. The results were obtained from the average of three repeated measurements.
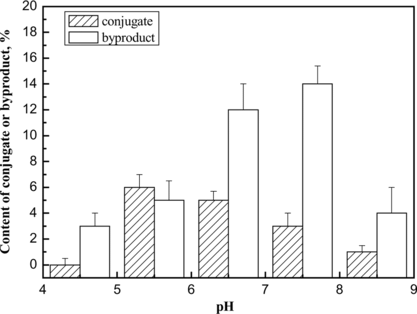
Optimization of the Reaction Condition in the Presence of PEG
PEG with varying concentration and molecular weight was used to regulate the physical environment of the protein solution of bovine hemoglobin and human serum albumin, before the addition of glutaraldehyde to chemically couple them. The yield and composition of the resulting products were determined by size-exclusion high performance chromatography and multi-angles laser light scattering (MALLS). In addition, since PEG was also a common protein precipitator, the total recovery of the reactant proteins was detected for the potential loss during the reaction process. The results were shown in , indicating that 9% (w/v) PEG4000 was optimum for the conjugation of hemoglobin and albumin.
Figure 7 Effect of concentration and molecular weight of PEG on the conjugation reaction of Hb and HSA. The optimum condition is annotated by arrow.
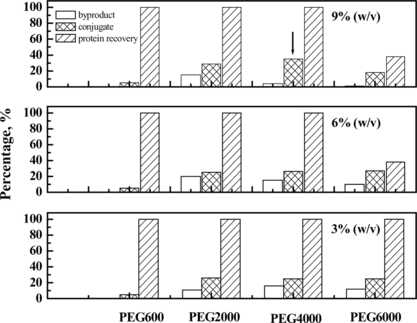
As shown in a, the conversion rate of the starting proteins to conjugates was greatly improved by adding PEG compared with the control reaction in the absence of PEG. SDS-PAGE showed in the presence of PEG the formation of HSA dimer (about 130 kDa in , Lane 5) was completely inhibited compared with the control reaction without PEG. Moreover, since Hb-Hb dimer was not detected in the preparation obtained in the presence of PEG, it could be concluded that the self-polymerization by-reaction of reactant proteins was effectively inhibited in the macromolecular crowding environment created by PEG. It is noteworthy that the molecular weight of the byproduct (, peak 1) was about twice that of 1:1 conjugate (, peak 2) as detected by MALLS (shown in and summarized in ). Therefore, under optimum condition of 9% (w/v) of PEG 4000, the reaction proceeded in a controlled mode with conversion yield of starting proteins to Hb-HSA increasing from 6% to 30%. The purity was about 88% of the total conjugates. Furthermore, the impurities were mainly tetrameric molecules of two Hb-HSA conjugates.
Table 1. Summary of the molecular weight parameters of the peaks corresponding to detected by MALLS
Figure 8 The profiles of the reaction mixture prepared in presence of PEG (A) and in absence of PEG (B), respectively. The samples were loaded on a size-exclusion high performance TSK-GEL G3000SW column, followed by elution at a flow rate of 0.5 ml min-1 50 mM PBS containing 0.1 M Na2SO4.
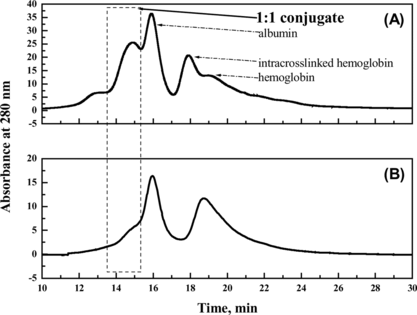
Figure 9 Characterization of the reaction mixture using SDS-PAGE. Lane1: native hemoglobin; lane 2: native albumin; lane 3: standard marker; lane 4: reaction mixture in the presence of PEG; lane 5: reaction mixture in the absence of PEG. All samples were boiled for 5 min. The stacking and running gels were 5 and 12% in acrylamide, respectively. The numbers on the left side of the gel indicated molecular weight of marker.
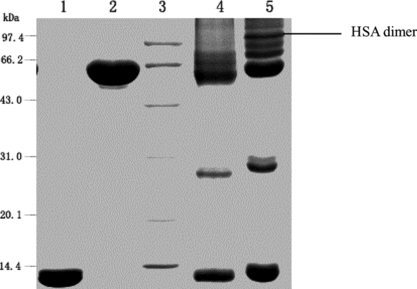
Figure 10 SE-HPLC and MALLS analyses of reaction mixture obtained under crowding condition by PEG. LS was laser signal; AUX1 was refractive index; AUX2 was absorption at 280 nm. The MALLS instrument was calibrated by standard monomer HAS. Astra V.4.9 software was used to calculate the molecular weights (the results are summarized in ). The flow rate was 0.4 ml/min. Peak 1 was the byproduct; Peak 2 was the target conjugate of 1:1 Hb-HSA; Peak 3 and peak 4 were the unreacted HSA and unreacted Hb, respectively.
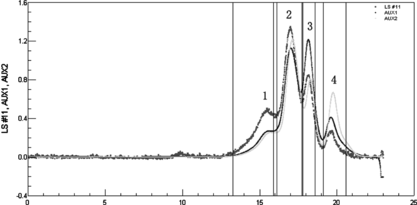
The unreacted hemoglobin was removed by ion exchange chromatography as described in literature [Citation[3]]. The O2 equilibrium curves of the product fractions were compared (). The P50 and Hill coefficient are summarized in , which indicated that the bioactivity of hemoglobin was impaired by the coupling of HSA. The change of these parameters might affect tissue acid-base balance, oxygen delivery to tissues and CO2 transport to the lungs [Citation[9]]. The introduction of PEG to the reaction environment was demonstrated to minimize the impairment. The P50 value and Hill coefficient of the product fraction obtained in the presence of PEG were 19.1 mmHg and 2.0, respectively, with comparison to 10.3 mmHg and 1.6 of that obtained in absence of PEG. This improvement was presumably due to the stabilization of PEG molecules to proteins [Citation[10]].
Table 2. Comparison of bioactivity of conjugates prepared under crowding and non-crowding conditions
Underlying Mechanism Speculation
PEG is a polymer having a number of magic applications in biotechnology, including protein precipitation, two-phase partitioning, cell fusion, protein refolding, etc. [Citation[11-14]]. Moreover, it is also a macromolecular crowding agent with special volume exclusion property and influence on proteins [Citation[15]]. Noga Kozer and Gideon Schreiber pointed out that the protein diffusion was changed dramatically by the high molecular mass polymers, such as PEG, which formed a flexible network in solution and made it into a porous medium [Citation[16]]. In this investigation, the optimum concentration and molecular weight of PEG () fitted well with the role of forming the flexible and porous networks. Presumably, the network structure played a key role in the controlling of the conjugation reaction.
It is assumed that the direct influence of PEG on the conjugation reaction is the increase of local concentration of the reactant proteins as a result of the volume exclusion effect through the crowding agent PEG. However, simple increase of starting protein concentration in the absence of PEG could not bring about such benefit. It just increased the byproduct formation and reduced the target conjugated as indicated in . The improvement of specific conjugation and inhibition of by-reaction might be assumed to be caused by the “micro-compartment” created by the addition of polyethylene glycol, which brings HSA and Hb close together. Thus, the chance of conjugation between HSA and Hb is increased.
Figure 12 Effect of protein concentration on the content of conjugate or byproducts in the conjugation preparation obtained in the absence of PEG, compared with the conjugation product prepared in the presence of PEG at the apparent protein concentration of 2 mg/ml, to investigate the effect of PEG on the conjugation reaction.
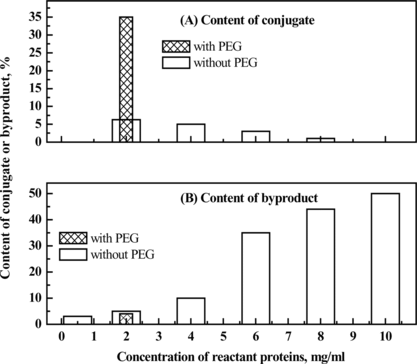
The MALLS detection revealed () that the molecular weight of the byproducts was twice that of the target conjugate. Since the micro-compartment facilitates the formation of Hb-HSA, there is a possibility that one conjugate may further react with another to form a byproduct consisting of two conjugates. Isoelectric focusing has revealed () that there is a broad band at pH 5.7 for the reaction products between the unreacted Hb (pI 6.8) and the unreacted HSA (pI 4.7). We have reason to believe that this band contains the target conjugate of Hb-HSA and the byproduct of the two conjugates.
Figure 13 Isoelectric focusing of Hb and/or HSA treated with glutaraldehyde. Lane 1: HSA reacted with glutaraldehyde; Lane 2: Hb reacted with glutaraldehyde; Lane 3: the resulting mixture of the conjugation reaction; Lane 4: standard marker; Lane 5: native Hb; Lane 6: native HSA. Employing conditions described in Materials and Methods.
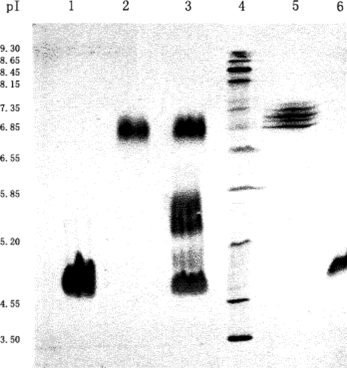
DISCUSSION
Preparation of well-defined conjugate of bovine hemoglobin and human serum albumin could be realized via the modulation of the physical environment of the reactant proteins by PEG. The reaction process is assumed as the following (). Firstly, Hb and HSA molecules with opposite charge form a pair, which is micro-compartmented into the same “pocket” of the porous network created by PEG with suitable molecular weight and concentration. Secondly, when glutaraldehyde is added into the reaction system, the Hb-HSA pair is chemically crosslinked by glutaraldehyde. The volume-exclusion of PEG increases the local concentration of the reactant proteins and thus also improved the yield of conjugate by controlling the conjugation reaction. Moreover, since the PEG favors the folded, native configuration of the hemoglobin in the reaction process, the bioactivity of hemoglobin is maintained ().
Figure 14 A scheme of the presumable conjugation process assisted by PEG. Step 1: Hb and HSA were dissolved; Step 2: the pH value was adjusted to pH 5.7 (the average of pI of Hb and that of HSA), and Hb molecule was positively charged and HSA was negatively charged; Step 3: PEG was added to adjust the physical environment of Hb and HSA. The oppositely charged Hb and HSA were assumed to form an electrostatic-interacting pair, and micro-compartmented into one pore in the network of PEG with proper concentration and molecular weight; Step 4: Glutaraldehyde was added, and conjugation of Hb and HSA in 1:1 ratio occurred, which simultaneously lost its net charge and then might further couple to the byproduct comprising Hb and HSA in 2:2 ratio (dimer of the target conjugate).
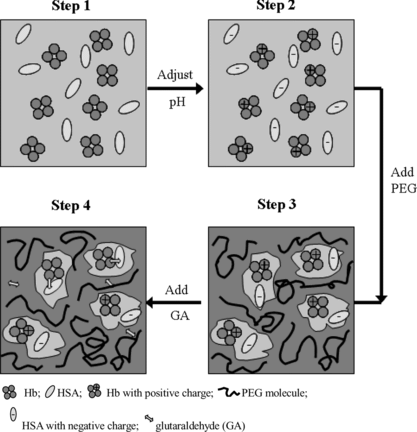
Compared with the conventional methods such as heterobifunctional cross-linking and multi-step cross-linking, this method is facile and effective. The method presented in this article can be further extended to the preparation of other conjugates through optimization of the molecular weight and concentration of polyethylene glycol.
The authors are thankful for the financial support from the Natural Science Foundation of China (Grant No. 20636010 and 20536050).
REFERENCES
- Chang, T.M.S. (1999). Future prospects for artificial blood. Trends Biotechnol. 17: 61–67.
- Lu, X.L., Zheng, C.Y., Shi, X.D., Wang, Y.Q., Suo, X.Y., Yu, P.Z., Xu, Y.H., Ma, T.M., Su, Z.G. (2005). Conjugate of bovine hemoglobin and human serum albumin as a candidate for blood substitute: characteristics and effects on rats. Art. Cells, Blood Subs. and Biotech. 33: 83–99.
- Hu, T., Su, Z.G. (2002a). Bovine serum albumin-bovine hemoglobin conjugate as a candidate blood substitute. Biotechnol. Lett. 24: 275–278.
- Bonhard, K.H., Boysen, U.F. (1981). Preparation of coupled hemoglobin molecules. US Patent 4336348.
- Hu, T., Su, Z.G. (2002b). A solid phase adsorption method for preparation of bovine serum albumin-bovine hemoglobin conjugate. J. Biotechnol. 100: 267–275.
- Lu, X.L., Zhao, D.X., Su, Z.G. (2004). Purification of hemoglobin by ion exchange chromatography in flow-through mode with PEG as an escort. Art. Cells, Blood Subs. and Biotech. 32: 209–228.
- Laemmli, U.K. (1970). Cleavage of structural protein during the assembly of the head of batcteriophage T4. Nature 227: 680–685.
- Evans, C.A., Farrar, W.L. (1997). Purification of the renaturable protein kinase PK55 by preparative isoelectric focusing and high performance electrophoretic chromatography and the generation of PK55 monoclonal antibodies by in vitro B cell stimulation. CAT Inst. 16: 497–502.
- Alayash, A.I., Summers, A.G., Wood, F., Jia, Y. (2001). Effects of glutaraldehyde polymerization on oxygen transport and redox properties of bovine hemoglobin. Arch. Biochem. Biophys. 391: 225–234.
- Zimmerman, S.B., Minton, A.P. (1993). Macromolecular crowding: biochemical, biophysical, and physiological consequence. Annu. Rev. Biophys. Biomol. Struct. 22: 27–65.
- Ellis, R.J. (2001). Macromolecular crowding: obvious but underappreciated. Trends. Biochem. Sci. 26: 597–604.
- Rohwer, J.M. (1998). Implications of macromolecular crowding for signal transduction and metabolite channeling. Proc. Natl. Acad. Sci. 95: 10547–10552.
- Tellam, R.L., Sculley, M.J., Nichol, L.W., Wills, P.R. (1983). The influence of poly(ethylene glycol) 6000 on the properties of skeletal-muscle actin. Biochem J. 213: 651–659.
- Bradley, A.J., Scott, M.D. (2004) Separation and purification of methoxypoly (ethylene glycol) grafted red blood cells via two-phase partitioning. J. Chromatogr. B. 807: 163–168.
- Minton, A.P. (1981). Excluded volume as a determinant of macromolecular structure and reactivity. Biopolymer 20: 2093–2120.
- Kozer, N., Schreiber, G. (2004). Effect of crowding on protein-protein association rates: fundamental differences between low and high mass crowding agents. J. Mol. Biol. 336: 763–774.