Abstract
PEGylation induced increase in colloidal osmotic pressure (COP) of Hb, one of the unique molecular properties of PEG-Hb conjugates, facilitates the neutralization of the vasoconstrictive activity of acellular Hb is a function of chemistry of conjugation of PEG-chains. The dependence of COP with the chemistry is a consequence of PEGylation induced weakening of interdimeric interactions. The conservative Extension Arm Facilitated (EAF) PEGylation exerts least influence on lowering the tetramer stability as compared to direct PEGylation. We have now designed a new, non-conservative EAF PEGylation that uses acylation chemistry to introduce the extension arm onto proteins to delineate the role of the extension arm and of the charge at the site of attachment in PEG-Hb conjugate on tetramer stability. The non-conservative EAF PEGylation does not lower the tetramer stability just as the non-conservative direct PEGylation of Hb. The impact of the extension arm in PEGylated Hb in terms of their structure and potential significance of higher tetramer stability of PEG-Hb conjugates generated by EAF-PEGylation in the in vivo toxicity and in the design of oxygen carrying plasma expander has been discussed.
Abbreviations | ||
COP | = | colloidal oncotic pressure |
4-PDS | = | 4,4′ – dithiodipyridine |
EAF | = | extension arm facilitated |
Hb | = | hemoglobin |
HPLC | = | high performance liquid chromatography |
PBS | = | phosphate buffered saline |
PEG | = | polyethelene glycol |
SEC | = | size exclusion chromatography |
Sulfo-EMCS | = | N-ε-maleimido caproyl sulfo succinimidyl ester |
TCEP | = | Tris (2-Carboxyethyl) phosphine. |
INTRODUCTION
The treatment of blood loss requires (i) plasma volume expansion and (ii) restitution of oxygen carrying capacity. Early studies of developing Hb based blood substitutes have essentially focused on the second aspect of treating the blood loss. The failure of earlier Hb derivatives that have been advanced as potential blood substitutes has been primarily attributed to their (i) vasoconstrictive activity in vivo, essentially resulting from the NO scavenging activity of Hb and (ii) autoxidation mediated in vivo toxicity of these products Citation[1–7].
Accordingly, new concepts of designing blood substitutes have emerged, particularly to overcome the vasoactivity of acellular Hb, and one significant strategy is to engineer the molecular properties of plasma expanders to Hb, i.e. convert Hb into oxygen carrying plasma expander Citation[8]. PEGylation of Hb has been now recognized as a unique approach to achieve this objective and generate a non-hypertensive and vasoinactive product Citation[9–11]. This novel concept has propelled protein chemists to develop new PEGylated Hb is and identify best PEG-conjugation chemistry to induce a desired level of solution properties to Hb to neutralize the vasoactivity with minimal perturbation to the structure/conformation and functional properties, i.e., to develop an ideal oxygen delivering resuscitation fluid Citation[12].
An extension arm facilitated (EAF) PEGylation that simplifies the PEGylation protocol by using the reaction of a maleimide with thiols has been developed by us. The reaction of thiols with maleimides is one of the best quantitative chemical modification reactions in protein chemistry. In this approach an aliphatic extension arm is engineered between the PEG-chains and the protein ε-amino group of surface Lys residues Citation[9], Citation[10], Citation[13], Citation[14]. A hexaPEGylated Hb, (SP-PEG5K)6-Hb developed by this protocol, is non-hypertensive and vasoinactive and appears to exhibit a slight vasodilator activity as well. This extension arm facilitated PEGylation platform has been used by Sangart, Inc. to generate their oxygen therapeutic, MP4, a hexaPEGylated Hb, and this product is in phase III clinical trial in Europe and phase II clinical trial in USA Citation[15].
The non-hypertensive PEGylated Hb generated by the EAF PEGylation platform carries only six copies of PEG-5K chains. Surprisingly, it's COP is comparable to that of non-hypertensive decaPEGylated bovine Hb of Enzon, which carries 10 copies of PEG-5K chains. The PEG-conjugation chemistry used in two systems are very distinct; the results suggest that chemistry of conjugation dictates the solution properties of PEGylated Hb and not the amount of PEG conjugated to the protein Citation[16]. Besides the difference in the chemistry of conjugation between the EAF-PEGylation and the Enzon PEGylation approach, these two non-hypertensive PEGylated products also differ in the presence of an extension arm between the PEG-chain and the protein. The products generated by EAF PEGylation, Cys-93(β) is also modified directly, i.e., without the extension arm. Therefore, it may be argued that a stronger interaction between the PEG-chains and the protein molecular surface in the samples PEGylated directly, induces a higher increase in the COP of PEG-Hb conjugate. The presence of the extension arm sandwiched between the PEG-chains and protein surface reduces the efficiency of the PEG-chains to increase the COP by a given mass of PEG. In an attempt to understand this molecular phenomenon, we have now conjugated PEG-chains directly to the amino groups of Hb (and/or αα -fumaryl Hb) without the PEGylation of Cys-93(β) and also without the use of extension arm. This is performed using two different PEG-conjugation chemistries (), namely reductive alkylation and acylation chemistry based PEGylation protocols Citation[10], Citation[17]. A detailed investigation of these PEGylated Hbs generated by direct PEGylation has established that these PEGylated products are essentially dimeric in solution. This reflects that direct PEGylation impacts a significant influence on inter-dimeric interactions of Hb (i.e. tetramer stability). A comparison of the molecular radius of the hexaPEGylated forms of uncrosslinked and intramolecularly crosslinked Hb generated by EAF-PEGylation with those of the products generated by two direct PEGylation platforms is presented in . It may be seen that the molecular radius of the hexaPEGylated Hbs generated from intramolecularly crosslinked Hb is significantly higher than that of the product generated from uncrosslinked Hb when direct PEGylation is used for surface decoration. However, the difference in molecular radius is only marginal when the surface decoration is achieved using EAF-PEGylation. As discussed in detail by Hu et al. Citation[18] and Li et al. Citation[19], the smaller molecular radius of hexaPEGylated Hb generated from the uncrosslinked Hb reflects PEGylation induced weakening of interdimeric interactions in Hb, and this is accompanied by higher COP (a result for higher number of particles, i.e. dissociated species). The surface decoration of Hb using acylation chemistry based direct PEGylation induced a higher increase in the PEGylation induced COP of Hb as compared to that by reductive alkylation, suggesting a role for the surface positive charge at the site of PEGylation in the PEGylation induced increase in COP.
Figure 1. Schematic representation of three types of linkages in PEGylated Hbs investigated for the correlation of PEGylation induced COP of Hb with the chemistry of conjugation.
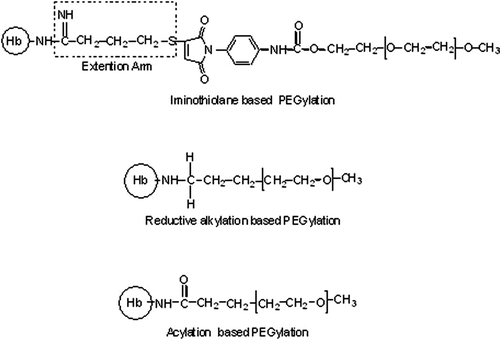
Table 1. Influence of PEG-conjugation platform on the molecular radius of hexaPEGylated Hb and of αα-fumaryl intramolecular crosslinked Hb
In the iminothiolane mediated EAF PEGylation, the extension arms are introduced onto Hb in conservative mode, i.e., without altering the positive charge of the ε-amino groups at the sites of linking the extension arms. It is conceivable that an extension arm attached to the protein in a non-conservative mode may also reduce the PEGylation induced weakening of the interdimeric interactions in Hb and hence the PEGylation induced increase in COP. Accordingly, we have now designed a new, non-conservative extension arm facilitated PEGylation platform, which combines the elements of acylation chemistry based PEGylation and the thiol maleimide chemistry based PEGylation. In this new approach, Hb is first modified with N-ε-maleimido caproyl sulfo succinimidyl ester (Sulfo-EMCS) to introduce desired number of maleimide groups on Hb and the product is then PEGylated using excess thio-PEG5K (). In an attempt to restrict the PEGylation of Hb only at the amino groups, the thiol of Cys-93(β) of Hb are reversibly protected as mixed disulfide with thiopyridine before modifying with sulfo-EMCS. We refer to this new approach as non-conservative EAF Maleimide Thio-PEG based PEGylation. The molecular properties of hexaPEGylated (thio succinimido caproyl-PEG-Hb) product generated from Hb and αα-fumaryl Hb have been compared with that of direct PEGylation products generated using SPA-PEG as well as with that of iminothiolane mediated EAF-PEGylation. The results have established that extension arm facilitated PEGylation neutralizes the direct PEGylation induced weakening of inter-dimeric interactions.
MATERIALS
Hemoglobin purified from the outdated blood from the blood bank was used for the preparation of PEGylated Hb. Adult human hemoglobin from the blood lysate was purified by DE52 ion exchange chromatography as per method explained by Manjula et al. Citation[20]. 4, 4′ dithiodipyridine (4-PDS) and Tris (2-Carboxyethyl) phosphine (TCEP) purchased from Sigma-Aldrich, St. Louis, MO, USA. [N-ε- Maleimidocaproyloxy] sulfosuccinimide ester (Sulfo-EMCS) was obtained from Pierce Chemicals, Rockford, IL, U.S.A. and thio PEG5000 from NOF, Tokyo, Japan. Phosphate Buffered Saline pH 7.4 used as universal buffer for the reaction.
METHODS
Non-conservative Extension Arm Facilitated PEGylation
The new PEGylation protocol involves multiple steps as discussed below:
Step 1: Preparation of Dithiopyridyl Hb (DTP-Hb) and Dithiopyridyl αα-fumaryl Hb (DTP-ααHb): Hemoglobin (0.25 mM) was reacted with 20-fold molar excess of 4-PDS at pH7.4 for overnight and at 4 °C. The unreacted 4-PDS was removed from the mixed disulfide of Hb with thiopyridine by dialysis and disappearance of free thiols confirmed using 4-PDS method Citation[21]. This mixed disulfide of Hb (DTP-Hb) and of intramolecularly crosslinked Hb (DTP-ααHb) were further concentrated using the Amicon concentrators.
Step 2: Attachment of desired number of maleimido caproyl chains on the reactive amino groups of DTP- Hb and DTP-αα Hb: Dithiopyridyl Hb (or DTP-αα Hb) (0.5 mM) was incubated with 10-fold molar excess of sulfo-EMCS in PBS buffer pH 7.4 at 4 °C for 2 hr. Reaction mixture was then dialyzed extensively at 4 °C for the removal of un-reacted sulfo NHS or its hydrolytic product. The dialyzed sample was then concentrated to 1 mM solution using Amicon concentrator.
Step 3: Reaction of Maleimido Caproyl DTP-Hb (or DTP-ααHb) with Thio PEG-5000: Dithiopyridyl Hb (or its intramolecularly crosslinked form) that has acylated with caproyl chains moieties carrying maleimide groups at their distal ends (0.5 mM) was reacted with 5 mM thio PEG5000 at pH 7.4 (PBS) overnight at 4 °C to achieve the surface decoration of Hb with PEG-chains. Unreacted PEG5K remaining in the solution was removed by dialysis. The PEGylated products were referred as thiosuccinimido caproyl PEG5K dithiopyridyl Hb [(TSC-PEG5K)-DTP- Hb] and thiosuccinimido caproyl PEG5K dithiopyridyl αα fumaryl Hb [(TSC-PEG5K)-DTP- ααHb].
Step 4: Regeneration of the thiol groups of (TSC-PEG5K)-DTP-Hb (or DTP-ααHb) by reduction using Tris (2-Carboxyethyl) phosphine (TCEP): TCEP was dissolved in PBS by adjusting the pH to 7.4 and preparing a concentration of 100 mM solution. (TSC-PEG5K)-DTP-Hb and (TSC-PEG5K)-DTP-ααHb were reacted with 20-fold molar excess of TCEP for 2 hours at 4°C. Excess TCEP and leaving thiopyridone is removed by dialysis. Free thiols after reduction were estimated by 4–PDS method Citation[21]. The PEGylated sample were concentrated further to 4% and stored in −80°C.
Analysis of hexaPEGylated hemoglobins (both crosslinked and uncrosslinked) were carried out by HPLC and SEC for their comparison with other hexaPEGylated Hb was done as explained in earlier publications Citation[10], Citation[22]. Colloidal Osmotic Pressure (COP) and viscosity of hexaPEGylated Hb's at 4% and hydrodynamic molecular radius (Rh) were measured as per Manjula et al. Citation[10].
RESULTS
Non-conservative Extension Arm Facilitated PEGylation of Hb
Hemoglobin is first modified with 4,4′-dithiodipyridine at Cys-93(β) to generate the mixed disulfide, DTP-Hb. This reversible protection of thiol prevents the modification at Cys-93(β) by the maleimide moiety of sulfo-EMCS and only NHS ester end of the reagent will react with the alpha and/or the ε-amino groups of Hb. The extension arms with maleimide moiety at the distal end are linked to Hb through an isopeptide bond. The maleimide group is the targeted site for PEGylation with thio-PEG5K. The maleimido caproyl dithiopyridyl Hb is reacted with thio-PEG5K and the PEG-Hb conjugate, (TSC-PEG5K)-DTP-Hb is generated.
The disulfide bonds between the cysteine 93(β) of Hb and thiopyridine is cleaved by reducing reagent TCEP. The new PEGylated Hb is referred to as (TSC-PEG5K)-Hb, is surface decorated with PEG chains, and has 2 free thiols on Cys-93(β). In a similar fashion, a hexaPEGylated derivative of αα-fumaryl Hb has also been generated and is referred to as (TSC-PEG5K)-αα Hb.
Characterization of (TSC-PEG5K) –Hb
The change in the hydrodynamic volume of Hb as a result of the new non-conservative EAF PEGylation has been assessed by size exclusion chromatography (A) and compared with that of hexaPEGylated Hb, (SP-PEG5K)6-Hb, generated using earlier conservative EAF PEGylation Citation[10]. Unmodified Hb elutes around 60 minutes, as a sharp peak in SEC (curve a). The new, non-conservative EAF PEGylation modifies Hb quantitatively. The new PEGylated product elutes much earlier than the unmodified Hb (curve b). The product elutes as a symmetrical peak at the position corresponding to the position of the Hb oligomerized to a molecular mass of approximately 256 kD Citation[12]. This elution pattern of the new product compares well with that of (SP-PEG5K)6-Hb (curve c), suggesting this product also carries six copies of PEG5K chains. Accordingly this product is referred to as hexa-thiosuccinimido-caproyl-PEG5K Hb, (TSC-PEG5K)6-Hb.
Figure 3. Influence of loss of charge at the site of attachment of extension arm on the hydrodynamic volume (A) and of HPLC patterns (B) of Hb and of intramolecularly crosslinked Hb. Comparison of hexaPEGylated (TSC-PEG5K)6-HbA and (TSC-PEG5K)6-ααHbA with (SP-PEG5K)6-HbA by SEC (A) and HPLC (B). Curve a, Hb; curve b, (TSC-PEG5K)6-Hb; curve c, (SP-PEG5K)6-Hb; curve d, ααHb; curve e, (TSC-PEG5K)6-ααHb.
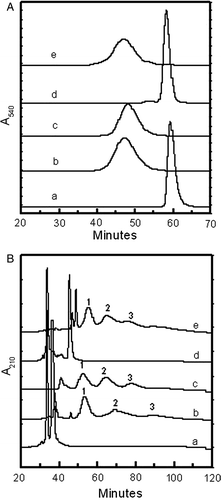
The HPLC pattern of the new product, (TSC-PEG5K)6-Hb, has been compared with that of unmodified Hb and of (SP-PEG5K)6-Hb in B. The HPLC pattern confirms the modification of both α and β-chains of Hb by the non-conservative PEGylation, with the generation of two major components (components 1 and 2) eluting around 55 minutes and 70 minutes, respectively. These, presumably, represent the mono and diPEGylated globin chains (curve b). After the diPEGylated globin peak, the presence of very small peak of triPEGylated globin chains (component 3) is also noted. The HPLC pattern of the hexaPEGylated Hb generated by our earlier conservative EAF PEGylation, (SP-PEG5K)6-Hb is also given for comparison (curve c). This sample also contains three PEGylated globin peaks Citation[22], Citation[23]. The distribution of these PEGylated globin peaks and the absence of much unmodified globin peaks suggests that the product contains about six PEG chains conjugated to Hb in this product, consistent with the above conclusion that this is a hexaPEGylated Hb. The HPLC pattern of the new PEGylated Hb is comparable to that of hexaPEGylated Hb, (SP-PEG5K)6-Hb. Since (SP-PEG5K)6-Hb has been shown to be essentially a tetramer under the conditions of SEC, it may be concluded that by engineering an aliphatic extension arm (caproyl chain) between the PEG chains in a non-conservative mode on the side chain amino groups of Hb, and then connecting it to a PEG-chain essentially inhibited PEGylation induced weakening of the interdimeric interactions of Hb.
The influence of the presence of αα fumaryl intra-molecular crosslink as reflected in the enhanced hydrodynamic volume of Hb on non-conservative extension arm facilitated PEGylation of Hb is seen in curve e. Intra-molecularly cross linked Hb (curve d) elutes in the same position as the uncross linked Hb (curve a). The non-conservative extension arm facilitated PEGylation of αα-fumaryl Hb increased the hydrodynamic volume of cross-linked Hb (curve e) to the same level as that of uncross linked Hb (curve b). This is reminiscent of the pattern generated on conservative EAF PEGylation of Hb, and is very distinct from the pattern of hexaPEGylated Hb generated by direct PEGylation. Direct PEGylation of uncross linked Hb, irrespective of whether it is carried out in a conservative or non-conservative mode, results in a product with a lower hydrodynamic volume as compared to that generated from the intramolecular cross linked Hb Citation[18]. The non-conservative extension arm engineered between the PEG-chains and the protein amino groups eliminates this differential influence, just as seen with conservative extension arm facilitated PEGylation.
Influence of Intra-molecular Crosslink in Hb on its Molecular Radius as Result of HexaPEGylation in a Non-conservative EAF PEGylation Mode
The molecular radius of the hexaPEGylated Hb generated by non-conservative EAF PEGylation (TSC-PEG5K)6-Hb is around 5.4 nm, and that generated from αα-fumaryl Hb is around 5.7 nm, as shown in . This difference is small as compared to the differences in the molecular radius of Hb as a result of the presence of intramolecular crosslink when direct PEGylation approaches are used. Those differences are in the range of 1 to 1.2 nm as shown in the .
Table 2. Influence of non-conservative extension arm on the PEGylation induced COP, viscosity and molecular hydrodynamic radius of hexaPEGylated Hb
Influence of Intra-molecular Cross-links on the Non-conservative EAF PEGylation Mediated HexaPEGylation Induced COP and Viscosity of Hb
Colloidal osmotic pressure of the hexaPEGylated sample generated by the non-conservative EAF PEGylation approach is comparable to the COP of the hexaPEGylated sample prepared by iminothiolane mediated EAF PEGylation () and is significantly lower than that seen on acylation chemistry based direct PEGylation of Hb. The lower COP of samples with comparable levels of PEGylation reflects the lower number of molecules in the solution. This confirms that by engineering an extension arm between the PEG chains and amino groups of Lys residues of Hb, even though it is in a non-conservative mode, reduces the impact of the PEGylation on interdimeric interactions of the tetramer.
Viscosity of the hexaPEGylated hemoglobin is also comparable to the other type of EAF PEGylation and is less than the hexaPEGylated Hb obtained by direct PEGylation, as shown in .
DISCUSSION
PEGylation of proteins and peptides, particularly surface decoration with multiple copies of PEG-chains has become an excellent protocol to enhance the efficacy of proteins with therapeutic values as very useful drugs Citation[9], Citation[24], Citation[25]. Extension Arm Facilitated PEGylation was developed by us to increase the accessibility of the side chains of proteins to the macromolecular PEG reagents and to take advantage of the quantitative aspect of the reaction of maleimides with thiols. The hexaPEGylated Hb generated by EAF PEGylation platform using PEG5K maleimide is non-hypertensive and vasoinactive.
Subsequent studies of hexaPEGylated Hbs generated by direct PEGylation, either by acylation chemistry or reductive alkylation chemistry based PEGylation, exhibit a higher COP as compared to the product generated by EAF-PEGylation Citation[18], Citation[19]. Originally this was thought to be a reflection of the dependence of PEGylation induced increase in COP of PEG conjugates on the chemistry of conjugation. An alternate, and equally valid explanation is that higher COP of hexaPEGylated Hbs generated by direct PEGylation reflects the dissociation of the PEGylated tetramers to PEGylated dimers, as a consequence of PEGylation induced weakening of the interdimeric interactions in the hexaPEGylated Hb. PEGylation studies with intramolecularly cross linked Hb have confirmed this Citation[18], Citation[19]. Further confirmation of this is that when the hexaPEGylated Hb is generated by conservative EAF PEGylation, the influence of intramolecular crosslinking on the molecular radius of hexaPEGylated Hb is very limited and influence is significantly higher as a consequence of direct PEGylation. Apparently, PEGylation induced weakening of inter dimeric interactions is very minimal when the sample is PEGylated by EAF PEGylation. Presumably, the extension arms sandwiched between the protein amino groups and the PEG-chains buffer the influence of PEGylation on the structure/conformation of the water filled central cavity of Hb.
Of the two direct PEGylation protocols that we have investigated to generate the hexaPEGylated Hb, acylation mediated direct PEGylation induced a highest level of increase in COP and this is essentially inhibited by the presence of intramolecular cross-links. The present study now establishes that an extension arm, engineered into Hb through acylation chemistry (non conservative mode), can also buffer the influence of direct acylation mediated PEGylation on the tetramer stability. The loss of charge at the site of direct PEGylation is not responsible for the direct PEGylation induced lowering of the tetramer stability.
The studies presented here clearly establish that the COP of (TSC-PEG5K)6-Hb is comparable to that of (SP-PEG-5K)6-Hb, suggesting that in the extension arm facilitated PEGylation, the loss of the positive charge at the site of attachment of the extension arm has very limited influence on the PEGylation induced COP of the protein.
It may be noted that hemospan (MP4), a hexaPEGylated derivative of Hb generated by the conservative extension arm facilitated PEGylation, is in Phase III clinical trial, and surprisingly has not shown any toxicity so far. On the other hand, vascular leakage of Enzon decaPEGylated bovine Hb has been reported and this decaPEGylated bovine Hb has been generated by a direct PEGylation platform Citation[26]. Accordingly, it is conceivable that the tetramer stability of this PEGylated Hb is lower relative to hexaPEGylated Hb generated by extension arm facilitated PEGylation platform and vascular leakage studies may have contribution by the lower tetramer stability of the Enzon decaPEGylated bovine Hb. The weakening of the interdimeric interactions can increase the rate of autoxidation and thereby increase the damage to the tissues. Further comparative studies of the toxicity of products generated by direct PEGylation and extension arm facilitated PEGylation a protocol is needed to establish the possible role of extension arm in the absence of significant levels of toxicity of MP4, and PEGylated Hbs in general.
Earlier studies have established that extension arm facilitated PEGylation is a very simple and cost-effective PEGylation platform to generate non-hypertensive PEGylated Hb. The present study exposes an unanticipated advantage of extension arm facilitated PEGylation, namely the propensity of the extension arms of PEGylated Hb prepared by this approach to buffer the impact of the PEGylation on the structure/conformation and interactions within the water-filled central cavity of Hb. We have recently compared the loss of accessible protein surface area as a result of direct PEGylation and by EAF-PEGylation using PEG-5K. These studies have established that the PEG-chains conjugated through EAF-PEGylation result in a lower levels loss of accessible surface area (Prabhakaran and Acharya; manuscript in preparation). Thus it is anticipated that perturbation of the protein hydration layer of Hb by EAF PEGylation is considerably lower as compared to that by direct PEGylation. This is, presumably, reflected as lowered impact on the conformation of water filled central cavity of Hb, i.e., tetramer stability of the hexaPEGylated Hb.
Acknowledgements
The authors of this paper would like to thank the National Institute of Health for its financial support by Grants HL71064 and HL58512. The authors of this article would like to thank Drs. Parimala Nacharaju, Tao Hu, Dongxia Li, Fantao Meng, and Maria Lobo for their valuable comments and help in preparing this manuscript.
References
- Kavdia M., Tsoukias N. M., Popel A. S. Am J Physiol Heart Circ Physiol 2002; 282(6)H2245–2253
- Resta T. C., Walker B. R., Eichinger M. R., Doyle M. P. J Appl Physiol 2002; 93(4)1327–1336
- Malhotra A. K., Kelly M. E., Miller P. R., Hartman J. C., Fabian T. C., Proctor K. G. J Trauma 2003; 54(5)915–924
- Fitzpatrick C. M., Savage S. A., Kerby J. D., Clouse W. D., Kashyap V. S. J Am Coll Surg 2004; 199(5)693–701
- Motterlini R., Foresti R., Intaglietta M., Winslow R. M. Am J Physiol 1996; 270(1 Pt 2)H107–114
- Motterlini R., Vandegriff K. D., Winslow R. M. Transfus Med Rev 1996; 10(2)77–84
- Winslow R. M. Annu Rev Med 1999; 50: 337–353
- Intaglietta M., Cabrales P., Tsai A. G. Annu Rev Biomed Eng 2006; 8: 289–321
- Acharya S. A., Friedman J. M., Manjula B. N., Intaglietta M., Tsai A. G., Winslow R. M., Malavalli A., Vandegriff K., Smith P. K. Artif Cells Blood Substit Immobil Biotechnol 2005; 33(3)239–255
- Manjula B. N., Tsai A. G., Intaglietta M., Tsai C. H., Ho C., Smith P. K., Perumalsamy K., Kanika N. D., Friedman J. M., Acharya S. A. Protein J 2005; 24(3)133–146
- Vandegriff K. D., Malavalli A., Wooldridge J., Lohman J., Winslow R. M. Transfusion 2003; 43(4)509–516
- Manjula B. N., Tsai A., Upadhya R., Perumalsamy K., Smith P. K., Malavalli A., Vandegriff K., Winslow R. M., Intaglietta M., Prabhakaran M., Friedman J. M., Acharya A. S. Bioconjug Chem 2003; 14(2)464–472
- Nacharaju P., Boctor F. N., Manjula B. N., Acharya S. A. Transfusion 2005; 45(3)374–383
- Nacharaju P., Manjula B. N., Acharya S. A. Artif Cells Blood Substit Immobil Biotechnol 2007; 35(1)107–118
- Winslow R. M. Artif Organs 2004; 28(9)800–806
- Conover C. D., Linberg R., Shum K. L., Shorr R. G. Artif Cells Blood Substit Immobil Biotechnol 1999; 27(2)93–107
- Hu T., Prabhakaran M., Acharya S. A., Manjula B. N. Biochem J 2005; 392(Pt 3)555–564
- Hu T., Manjula B. N., Li D., Brenowitz M., Acharya S. A. Biochem J 2007; 402(1)143–151
- Li, D., Hu, T., Manjula, B. N., and Acharya, S. A. (2008). Biochim Biophys Acta 11: 11 ( Epub ahead of print).
- Manjula B. N., Acharya S. A. Methods Mol Med 2003; 82: 31–47
- Grassetti D. R., Murray J. F., Jr. Arch Biochem Biophys 1967; 119(1)41–49
- Ananda K., Nacharaju P., Smith P. K., Acharya S. A., Manjula B. N. Analytical Biochemistry 2008; 374(2)231–242
- Li D., Manjula B. N., Acharya A. S. Protein J 2006; 25(4)263–274
- Awasthi V. D., Garcia D., Klipper R., Goins B. A., Phillips W. T. J Pharmacol Exp Ther 2004; 309(1)241–248
- Asanuma H., Nakai K., Sanada S., Minamino T., Takashima S., Ogita H., Fujita M., Hirata A., Wakeno M., Takahama H., Kim J., Asakura M., Sakuma I., Kitabatake A., Hori M., Komamura K., Kitakaze M. J Mol Cell Cardiol 2007; 42(5)924–930
- Baldwin A. L. Blood and the intestinal microcirculation: extravasation and ultrastructural alterations. Advances in Blood Substitutes: Industrial Opportunities and Medical Challenges, R. M. Winslow, K. D. Vandergriff, M. Intaglietta. Birkhaüser, Boston 1997; 19–37