Abstract
Living cells encapsulated in polymeric shells are receiving increasing attention because of their possible biotechnological and biomedical applications. The aim of this work is to evaluate how different polyelectrolyte coatings, characterized by different numbers of polyelectrolyte layers and by different polyelectrolyte conformations, affect the viability of encapsulated biological material. We demonstrate the ability to individually encapsulate HL-60 cells as well as rat pancreatic islets within polymeric shells consisting of different PE layers using the layer-by-layer process. Coating of HL-60 cells allows for surviving and functioning of cells for all applied PE as well as for different numbers of layers. The islets encapsulated in applied polyelectrolytes exhibited the lower level of mitochondrial activity as compared to non-encapsulated islets. Nevertheless, encapsulated islets exhibited comparable absorbance values during the whole period of culture. Polyelectrolyte coating seems to be a promising way of allowing capsule void volume minimization in a model of encapsulated biological material for local production of biologically active substances.
Introduction
Living cells encapsulated in polymeric shells are receiving increasing attention because of their wide range of possible applications in biotechnology and medicine.
Cells can be encapsulated using different techniques and devices like diffusive chambers, macrocapsules, and microcapsules. In medical applications, especially encapsulated islets transplantation Citation[1], Citation[2], the relatively large size of conventional microcapsules produces transplant volume not suitable for infusion into the portal vein of the liver Citation[3], Citation[4], the clinically preferred site for transplantation of pancreatic islets. A special case of microencapsulation is conformal coating, which allows one to minimize capsule void volume by deposition coatings that conform to the surface of cells.
Such coatings have been fabricated using a number of processes, including discontinuous gradient density centrifugation Citation[5], interfacial polymerization Citation[6], and emulsification Citation[7]. Conformal coatings may also be produced by layer-by-layer technique (LbL), using self-assembly processes of polymers involving electrostatic interactions. The alternate deposition of oppositely charged polyions onto charged substrates allows one to build up polyelectrolyte multilayers. It has been shown that polyelectrolyte multilayers can be tuned to generate thicknesses from 5 to about 50 nm, and alter capsule permeability, based on composition and layer number Citation[8]. Some authors have shown that polyelectrolyte layers are useful in generating nanotemplated cell capsules, e.g. poly(styrene sulfonate)/poly(allylamine) layers on erythrocytes Citation[9]. Yeast cells encased within poly(styrene sulfonate)/poly(allylamine) shells were able to maintain their viability, functionality, and normal exchange of nutrients and waste Citation[10], Citation[11]. Some authors demonstrated the ability to individually encapsulate mouse mesenchymal stem cells within polymeric shells consisting of hyaluronic acid and poly(L-lysine) using the LbL technique Citation[12]. LbL assembly has been used to modify platelet surfaces with antibodies as a means of investigating targeted-delivery mechanisms within the walls of blood vessel substitutes Citation[13] and for encapsulation of E.coli cells Citation[14]. Preliminary attempts concerning in vivo survival and function of nanoencapsulated cells have been made Citation[15].
The aim of the work is to evaluate how different polyelectrolyte (PE) coatings, characterized by different numbers of PE layers and by different PE conformations, affect the viability of encapsulated biological material. We demonstrate the ability to individually encapsulate HL-60 cells as well as pancreatic islets within polymeric shells using the LbL technique as a model of encapsulated in polymer shells biological material for future applications in local production of biologically active substances.
MATERIALS AND METHODS
Materials
Reagents: poly(allylamine hydrochloride) (MW 56 kD) (Sigma, USA); poly(styrenosulfonate, sodium salt) (MW 70kD) (Sigma, USA); poly-L-lysine hydrobromide (MW 15-30kD) (Sigma, USA); poly(ethylenimine) (MW 60 kD) (Aldrich, USA); propidium iodide (Sigma, USA); (3-4,5-dimethylthiazol-2-yl)-2,5-diphenyltetrazolium bromide) (Sigma, USA) (MTT); Histopaque ρ = 1,083 (Sigma, USA); microbeads – FluoroSpheres carboxylate modified microspheres yellow green fluorescent (Invitrogen Molecular Probes, USA).
Media: RPMI-1640 (Biomed, Poland); Hanks (Wytwórnia Surowic i Szczepionek, Poland); newborn calf serum NCS (Biochorom, UE); fetal bovine serum (FBS) (SAFC Biosciences, USA).
Culture media: RPMI-1640 suplemented 10% NCS (RPMI-1640/10%NCS) or 20% FBS (RPMI-1640/20%FCS).
Cell line: HL-60 promyelomonocytic human cell line; Jurkat–human lymphocytes T cell line.
Islets of Langerhans – isolated from pancreas of rats WAGFlow cytometry:The presence of organisms was assessed using FACS Calibur flow cytometer (Becton Dickinson Immunocytochemistry Systems, USA) equipped with an argon ion (488 nm) laser. The results were processed by the CellQuest software system (Becton Dickinson, USA). Organisms were separated from other events on light scatter characteristics.
AFM measurements: A nanoscope V AFM microscope (Veeco Instruments, Inc.) was used in imaging the surface of the samples. The silicon nitride cantilevers with a spring constant ca. 0.06 Nm−1 (manufacturer data) were applied for imaging in Contact Mode AFM (CM-AFM). Presented images were visualized in dedicated Nanoscope software.
Methods
Evaluation of polyelectrolites cytotoxity: the culture of Jurkat cells in presence of different polyelectrolite layers adsorbed on the membrane support.
The Jurkat cells were cultured (5% CO2, 37°C) in culture medium RPMI-1640/10%NCS in the presence of polyelectrolytes adsorbed on the biocompatible membranes as a support to assess the polyelectrolyte cytotoxity. The biocompatible polypropylene surface modified by siloxanes hollow fibers Citation[16] was applied as support.
The supports with adsorbed polyelectrolytes poly-L-lysine (PLL), poly-allylamine (PAH) layer, bilayer consisted of poly-L-lysine layer and poly-ethylenimine layer (PLL/PEI), or poly-L-lysine layer and poly-styrenosulfonate layer (PLL/PSS) were immersed in cell suspension. Cells were cultured for 5 or 8 days. As a negative control, the Jurkat cells were cultured without addition of support material with adsorbed polyelectrolite. After 5- or 8-day culture the cells’ viability was assessed in cytochemical reaction using propidium iodide (PI) in flow cytometer.
Coating of HL-60 with polyelectrolytes
Cationic layer deposition: 5×106 HL-60 cells were incubated with PLL or PAH solution in 0,1 M NaCl at concentration 1mg/ml at pH 7.2. After 6 min deposition time cells were washed 2 times with RPMI-1640 at 1000 rpm for 3 minutes to remove unadsorbed polyelectrolyte.
Anionic layer deposition
Encapsulated in polycation HL-60 cells were incubated with PEI or PSS solution in 0,1 M NaCl at concentration 1mg/ml at pH 7.2. After 4 min deposition time they were washed 2 times with RPMI-1640 at 1000 rpm for 3 minutes to remove unadsorbed polyelectrolytes. The process was repeated to obtain more than one bilayer consisting of cationic and anionic layers.
Encapsulated cells were cultured (37°C, 5%CO2) in culture medium RPMI-1640/20%FCS for 8 days. The mitochondrial activity of cells was assessed after 1, 5, or 8 days of culture in MTT test. The yield of nanocapsules was assessed as well. The encapsulation was performed with PLL/PEI or PLL/PSS or PAH/PEI bilayers.
Coating of rat pancreatic islets with polyelectrolytes
250 rat pancreatic islets of Langerhans were incubated in polyelectrolyte suspension as described for HL-60 cells to deposit polyelectrolyte layers.
Encapsulated islets were cultured (37°C, 5%CO2) in culture medium RPMI-1640/10%NCS up to 8 days. The mitochondrial activity of cells was assessed in MTT test. Encapsulation was performed by coating with polyelectrolyte PAH layer or PAH/PSS or PLL/PEI bilayer.
MTT test
The (3-4,5-dimethylthiazol-2-yl)-2,5-diphenyltetrazolium bromide (MTT) assay was performed. Encapsulated cells were seeded at a density of 10000 cells or 10 islets per well in a 96-well plate and incubated in a culture medium RPMI-1640/20%FCS or RPMI-1640/10%NCS, respectively. The MTT solution in concentration 5mg/ml was added to the culture in a 1:10 dilution of the medium and 2-hour incubation was performed. Then the suspension was centrifugated (1000 rpm, 3min). The formazan crystals were dissolved with 100 µl DMSO added to the pellet. After 10 minutes of shaking an absorbance was measured at 540 nm in spectrophotometer.
AFM measurements
Encapsulated cells were visualized in 3D form in dedicated Nanoscope software. Cells were placed on the Nucleopore polycarbonate membrane (Whatman Ltd.) of 800 nm pore size by gravity drying method. Contact mode AFM images were obtained at room temperature.
AFM measurements of average layer thickness were performed using microbeads of 0,2 µm diameter. A mechanical trapping in a microporous membrane was used to immobilize encapsulated microbeads. Nonencapsulated microbeads were applied as a reference. Contact mode AFM images were obtained at room temperature.
Islets isolation using a modified Lacy and Kostianovsky method Citation[17]
Rat pancreatic islets were isolated by intraductal injection of 10 ml of 1000 U/ml collagenase solution in Hanks/1%FCS to common bile duct before pancreas resection. Pancreas digestion was performed in a water bath at 37°C by putting it onto plastic Petrischale. Then, the tissue was transferred to a 50 ml centrifugation tube containing 20 ml of cold Hanks/1%FCS. The enzyme was washed out twice by Hanks/1%FCS (4°C, 600×g, 5 minutes). The digested material was filtered through a sterile mesh (500-nm pores), transferred to a 10 ml centrifugation tube filled with Hanks 1%FCS, and centrifugated (600×g, 5 minutes). Islet purification was performed by discontinuous density gradient centrifugation with 1,083 g/ml Histopaque. Then, the islets were washed by Hanks/1%FCS (800×g, 16 min.) and suspended in culture medium RPMI-1640/10%FCS at pH = 7.4.
RESULTS
Evaluation of Polyelectrolytes Cytotoxity
The cytotoxity of polyelectrolytes was assessed using Jukat cell line. It was observed that the number of viable Jurkat cells (organisms with cell membrane impermeable for PI), cultured in the presence of support with adsorbed polyelectrolyte PLL layer, bilayer PLL/PEI, or PLL/PSS was comparable to the control for every polyelectrolyte layer or bilayer after 8-day culture (). Experiments were performed in six repeatings.
Figure 1. The percent number of Jurkat cells alive, cultured in the presence of polyelectrolytes adsorbed on modified polypropylene support: poly-L-lysine (PLL) layer, bilayer of poly-L-lysine and poly-ethylenimine (PLL/PEI), or poly-L-lysine and poly-styrenosulfonate (PLL/PSS).
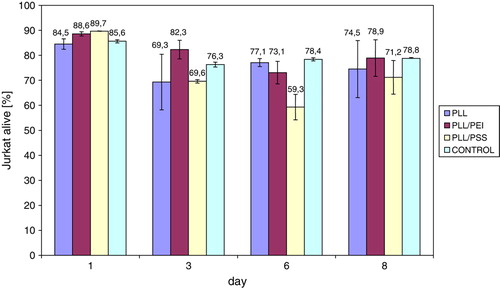
The viable cell's share after 5 days of culture in the presence of support with adsorbed polyelectrolyte PAH layer, bilayers PAH/PEI, or PAH/PSS is comparable to a control (). Experiments were performed in six repeatings.
Nanoencapsulation of HL-60 with Polyelectrolytes
The mean yield of nanocapsules obtained after polyelectrolytes deposition on HL-60 cells was about two times lower for PLL/PSS coating as compared to PLL/PEI coating for one bilayer as well as for three bilayers ().
Figure 3. The mean yield of nanocalsules of 5 milions HL-60 cells. PLL/PEI 1bl – cells covered with poly-L-lysine and polyethylenimine bilayer; PLL/PEI 3bl – cells covered three times with poly-L-lysine and polyethylenimine bilayer (cumulatively 6 layers); PLL/PSS 1bl–cells covered with poly-L-lysine and poly-styrenosulfonate bilayer; PLL/PSS 3 bl-cells covered three times with poly-L-lysine and poly-styrenosulfonate (cumulatively 6 layers); bl-bilayer.
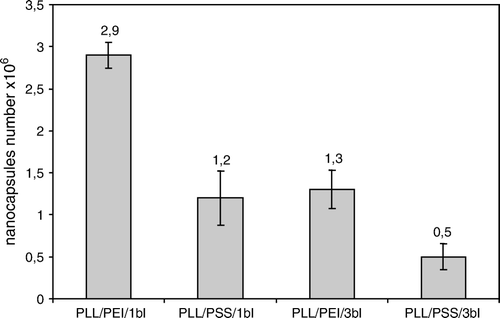
The properties of cells coated by different numbers and conformations of PE layers were assessed by mitochondrial activity evaluation. The cells encapsulated within PLL/PEI or PLL/PSS bilayers single or threefold were examined. The obtained values for different PE conformations and numbers of polyelectrolyte layers were comparable with control on 1st, 5th, and 8th day of culture ().
Figure 4. The mean formazan production by nanoencapsulated and non-encapsulated (control) HL-60 cells. PLL/PEI 1bl – cells covered with poly-L-lysine and poly-ethylenimine; PLL/PEI 3bl – cells covered three times with poly-L-lysine and poly-ethylenimine bilayer (cumulatively 6 layers); PLL/PSS 1bl – cells covered with poly-L-lysine and poly-styrenosulfonate; PLL/PSS 3 bl-cells covered three times with poly-L-lysine and poly-styrenosulfonate (cumulatively 6 layers).
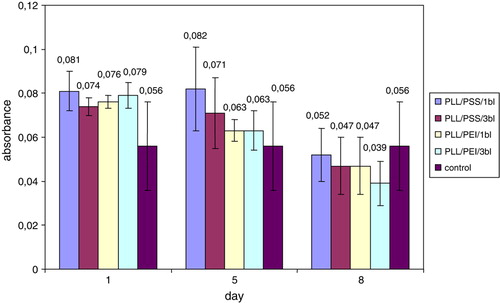
The mitochondrial activity was evaluated for cells nanoencapsulated with bilayers PLL/PEI triple or sixfold. The obtained values of absorbance for: non-encapsulated cells (negative control), cells covered with bilayers consisting of poly-L-lysine layer and poly-ethylenimine layer triple or sixfold were, respectively: 0.054±0.05, 0.058±0.011, 0.065±0,007 (n = 6). There was no difference in MTT test observed between 3-bilayer or 6-bilayer coating and a control after 8 days of culture. Nevertheless, the mean yield of PLL/PEI 6-bilayer was more than two times lower than the 3-bilayer coating. It was obtained (1.20±0.18)×106 or (0.52±0.03)×106 nanocapsules.
The mitochondrial activity of cells coated with triple bilayers PLL/PEI or PAH/PEI was compared. There was no difference observed between two types of bilayers and a control after 8 days of culture. The obtained values of absorbance for: non-encapsulated cells (negative control), cells covered with triple bilayer PLL/PEI or PAH/PEI were, respectively: 0.054±0.019, 0.041±0.008, 0.046±0.01 (n = 6).
Nanoencapsulation of Pancreatic Rat Islets with Polyelectrolytes
The rat pancreatic islets of Langerhans were coated with single PAH layer, threefold PAH/PSS, or PLL/PEI bilayer.
The mitochondrial activity was assessed for islets encapsulated within a single PAH layer or threefold PAH/PSS bilayer after 48-hour culture. The obtained values for different polyelectrolyte layers were about two times lower as compared to a control ().
Figure 5. The mean formazan production by non-encapsulated (control) and nanoencapsulated rat islets of Langerhans during 48-hour culture. PAH – islets covered with poly-allylamine layer; PAH/PSS – islets covered with bilayer of poly-allylamine hydrochloride and poly-styrenosulfonate.
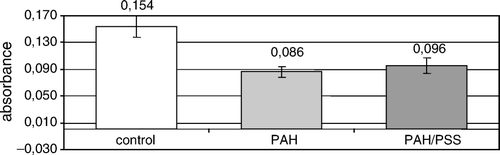
The mitochondrial activity was assessed in MTT test for islets encapsulated within threefold PLL/PEI bilayer after 8-day culture. The obtained absorbance values for nanocoated islets were about two times lower as compared to a control (). The nanocoated islet SEM image is presented in . The coated islets integrity was preserved during the whole period of culture ().
Figure 6. The mean formazan production by non-encapsulated (control) and nanoencapsulated rat islets of Langerhans during 8-day culture. PLL/PEI – islets covered three times with poly-L-lysine and poly-ethylenimine bilayer (cumulatively 6 layers).
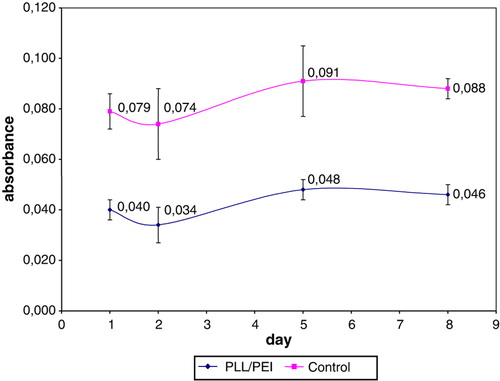
AFM Measurements
The encapsulated HL-60 cell's AFM image is presented in . AFM measurements of average layer thickness was performed using microbeads of 0,2 µm diameter. One-layer coated microbeads are presented in and . Analyzing the profiles of images the layer thickness may be assessed. The average thickness of the layers was 17±4.3 nm.
Figure 9. HL-60 cell coated with triple bilayer of poly-L-lysine and poly-ethylenimine, deposited on filtration membrane. AFM image of surface (Nanoscope V, Veeco Instruments Inc., USA).
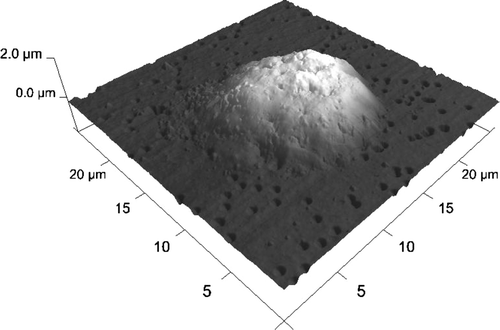
DISCUSSION
There was no cytotoxic effect observed for evaluated polyelectrolyte layers PLL, PAH, and bilayers PLL/PEI, PLL/PSS, PAH/PEI, PAH/PSS in the aspect of biocompatibility. There were no significant differences in viability of Jurkat cells, cultured in presence of polyelectrolytes adsorbed on biocompatible support, as compared to a control.
Coating of HL-60 cells with polyelectrolyte bilayers PLL/PEI, PLL/PSS, or PAH/PEI allowed for surviving and functioning of cells for all polyelectrolyte bilayers types as well as for a different number of layers, although the best yield of nanocapsules was obtained for the bilayer PLL/PEI. However, LbL technique is a mild process without covalent interaction involvement, allowing for maintenance of the integrity of coated cells, and polyelectrolyte bilayers PAH/PSS or PLL/PEI exerted no cytotoxic effect on cells; both applied conformations of bilayers exerted influence on rat pancreatic islets’ functioning. The islets coated with these polyelectrolytes exhibited mitochondrial activity level about two times lower as compared to non-encapsulated islets during the whole period of culture. Nevertheless, the mitochondrial activity values obtained for encapsulated PLL/PEI islets were comparable during the whole period of culture.
The disparity in reaction of HL-60 cells and pancreatic islets on PE coating might be caused by different charge value beneficials for each type of cell.
There is some discrepancy in literature reports concerning the applicability of certain polyelectrolytes for encapsulation of cells. The toxicity of PLL as well as many other polycations toward a variety of cell types has been documented Citation[18]; however, PLL is widely employed in encapsulation with polyelectrolyte shells as well as in conventional microcapsules. Some authors observed that PAH/PSS films maintain human islets’ viability of function Citation[19]. The others observed in comparable experiments islets’ viability decline using confocal microscopy Citation[15].
In our experiments, the functional parameters of coated cells differed depending on the type of cells; nevertheless, none of the applied PE coatings caused the cell's apoptosis and necrosis.
The ability to tune these layers, allowing proper nutrients and product transport between the cell and external environment, is currently under our investigation.
Polyelectrolyte coating opens a means for manipulation through cell charge and electrical attraction. It seems to be a promising way of allowing capsule void volume minimization for therapeutic treatments as well as for biotechnological applications.
CONCLUSIONS
Nanocoating of cells seems to be a promising way of allowing capsule void volume minimization for biomedical purposes, especially for islet transplantation. In the study, applied polyelectrolyte coatings may have application for encapsulation of cells for further transplantation purposes. The LbL process proceeded without covalent interaction, allowing for maintenance of cell integrity and functioning. The PLL/PEI conformation, allowing one to obtain the best yield of nanocapsules, may be recommended for coating shells, although all applied PE conformations in nanocoating of cells allowed for survival and functioning of encapsulated HL-60 cells as well as rat pancreatic islets of Langerhans. The number of applied PE layers does not influence the functioning of coated cells.
Acknowledgement
Declaration of interest: The authors report no conflicts of interest. The authors alone are responsible for the content and writing of the paper.
References
- Calafiore R., Basta G., Luca G., Calvitti M., Calabrese G., Racanicchi L., Macchiarulo G., Mancuso F., Guido L., Brunetti P. Grafts of microencapsulated pancreatic islet cells for the therapy of diabetes mellitus in non-immunosuppressed animals. Biotechnol. Appl. Biochem. 2004; 39: 159–164
- Calafiore R. Alginate microcapsules for pancreatic islet cell graft immunoprotection: struggle and progress towards the final cure for type 1 diabetes mellitus. Expert Opinion on Biological Therapy. 2003; 3(2)201–205
- Calafiore R., Basta G., Luca G., Boselli C., Bufalari A., Giustozzi G.M., Moggi L., Brunetti P. Alginate/polyaminoacidic coherent microcapsules for pancreatic islets graft immunoisolation in diabetic recipients. Ann. N.Y. Acad. Sci. 1997; 831: 313–322
- Schneider S., von Mach M., Kraus O., Kann P., Feilen P.J. Intraportal transplantation of allogenic pancreatic islets encapsulated in barium alginate beads in diabetic rats. Artif Organs 2003; 27(11)1053–1056
- Sefton M.V., May M.H., Lahooti S., Babensee J.E. Making microencapsulation work: conformal coating, immobilization gels and in vivo performance. J. Contr. Release. 2000; 65(1–2)173–86
- Hill R.S. Immunoisolation of adult porcine islets for the treatment of diabetes mellitus. The use of photo-polimerizable polyethylene glycol in the conformal coating of mass-isolated porcine islets. Ann. N. Y. Acad. Sci. 1997; 831: 332–343
- Calafiore R., Basta G., Luca G., Boseli C., Bufalari A., Cassarani M.P., Giustozzi G.M., Brunetti P. Transplantation of pancreatic islets contained in minimal volume microcapsules in diabetic high mammalians. Ann. N. Y. Acad. Sci. 1999; 875: 219–232
- Sukhorukov G., Donath E., Lichtehfeld H., Knippel E.M., Badde A., Mohwald H. Layer-by-layer self assembly of polyelectrolytes on colloidal particles. Colloids Surf. A 1998; 137: 253–266
- Donath E., Moya S., Neu B., Sukhorukov G.B., Georgieva R., Voigt A., Baumler H., Kiesewetter H., Mohwald H. Hollow polymer shells from biological templates: Fabrication and potential applications. Chem. Eur. J. 2002; 8(23)5481–5485
- Diaspro A., Silvano D., Cavalleri O., Gliozzi A. Single living cell encapsulation in nano-organized polyelectrolyte shells. Langmuir 2002; 18(13)5047–5050
- Svaldo-Lanero T., Krol T., Magrassi R., Diaspro A., Rolandi R., Gliozzi A., Cavalleri O. Morphology, mechanical properties and viability of encapsulated cells. Ultramicroscopy 2007; 107: 913–921
- Veerabadran N.G., Goli P.L., Stewart-Clark S.S., Lvov Y.M., Mills D.K. Nanoencapsulation of stem cells within polyelectrolyte multilayer shells. Macromol. Biosci. 2007; 7: 877–882
- Ai H., Fang M., Jones S., Lvov Y.M. Electrostatic layer-by-layer nanoassembly on biological microtemplates: platelets. Biomacromolecules 2002; 3(3)560–564
- Hillberg A., Tabrizian M. Biorecognition through layer-by-layer polyelectrolyte assembly: In-situ hybridization on living cells. Biomacromolecules 2006; 7(10)2742–2750
- Wilson J.T., Wanxing C., Chaikof E.L. Layer-by-layer assembly of a conformal nanothin PEG coating for intraportal islet transplantation. Nano Lett. 2008; 8(7)1940–1948
- Granicka L., Werynski A., Kawiak J. Polypropylene surface modified membranes for immunoisolation. Sep Purif Technol. 2005; 41: 221–230
- Lacy P.E., Kostianovsky M. Method for the isolation of intact islets of Langerhans from the rat pancreas. Diabetes. 1967; 16: 35
- Fischer D., Li Y.X. In vitro cytotoxity testing of polycations: influence of polymer structure on cell viability and hemolysis. Biomaterials 2003; 24(7)1121–1131
- Krol S., del Guerra S., Grupillo M., Diaspro A., Gliozzi A., Marchetti P. Multilayer nanoencapsulation. New approach for immune protection of human pancreatic islets. Nano Lett. 2006; 6(9)1933–1939