ABSTRACT
Between 1940 and the late 1950s, the United Kingdom had a limited biological program to provide a retaliatory capability should UK forces be attacked using biological warfare (BW). Aspects of BW defense have been investigated from 1940 up to the present day. Techniques, processes, and equipment used within biological research programs are recognized to have dual-use applications; aerobiology is one such dual-use area. Research during these offensive and defensive eras of the UK BW research program has contributed to aerobiological science, leading to a number of positive changes in some areas, including laboratory safety; understanding of infection by the aerosol route; and survival, detection, and identification of airborne pathogens. This article will discuss the historical contributions made to aerobiology science, the global contemporary legislation that governs dual-use research, and a modern case study based upon this type of investigation. The article will contribute to our understanding of the dual-use aspects of a BW program.
Within the United Kingdom, the availability of information on biological warfare (BW) activity by foreign governments increased in the 1920s and 1930s.Footnote1 Following claims of German interest in attacking the London Underground and Paris Metro, British independent scientists and civil servants regularly met to determine the risks from BW. The Committee of Imperial Defense (CID) considered this subject for the first time during the mid-1930s, and formed a special Sub-Committee on Bacteriological Warfare.Footnote2 Further concerns around Germany’s possible use of BW led to a decision to start a practical program of investigation.Footnote3
In collaboration with the Medical Research Council (MRC), the Biology Department Porton (BDP) was quietly formed, in 1940, to provide experimental evidence on the feasibility of BW.Footnote4 The BDP carried out laboratory and field work over the next five years, including the development of a retaliatory weapon based upon cattle cake containing Bacillus anthracis spores, a series of field trials utilizing biological munitions, and early investigation of penicillin treatment of anthrax.Footnote5
Experimental aerobiology
The need to investigate aerosols led to research on techniques and equipment to enable experimental progress. During World War II, the BDP focused its investigations within the scientific field of aerobiology, as did its successors, the Microbiological Research Department (MRD) and the Microbiological Research Establishment (MRE).Footnote6 “Aerobiology” can be defined as the study of airborne biological particles (microorganisms, pollen, spores, and seeds) and their movements and effects on human, animal, and plant health, and on the environment. Investigation of intoxication using botulinum toxin delivered by the inhalation route were first carried out by David Willis Wilson Henderson (Physiology Section, Chemical Defence Experimental Station—CDES) and John Henry Gaddum (CDES) in 1940 using a simple apparatus involving a collison atomizer (originally used for inhalation therapy). No further information on the experimental set-up is available. The collison atomizer was originally introduced, by William Evan Collison, as an “inhaler” to aerosolize drugs for inhalation therapy.Footnote7
The “Henderson apparatus”
In April 1942, BDP published a report outlining a new apparatus it had developed to produce a fine particulate cloud from a liquid suspension of bacteria and enable the assessment of toxicity and/or aerosol survival.Footnote8 This was the first description of the “Henderson apparatus,” a piece of equipment used and developed during the many aerobiological investigations of the last seventy-seven years. In September 1943, Henderson published details of an updated version of his apparatus.Footnote9 shows an early Henderson apparatus displayed across laboratory benches. Following World War II, the release of information from wartime studies led to the publication of a scientific paper on the equipment in the open literature.Footnote10
Figure 1. An early Henderson apparatus extended across laboratory benching to show the individual components. Source: DEFE 55/132, D.W. Henderson, “A Note on an Improved Type of Laboratory Apparatus for Producing Clouds of ‘Toxic Dusts’,” BDP 30, September 16, 1943. © Crown copyright, Dstl.
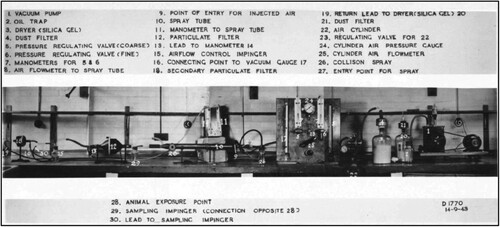
The original Henderson apparatus had several advantages and disadvantages compared with other equipment available for the assessment of toxicity or aerosol survival, such as modified Reyniers-type chambers. These were used for whole-body-exposure techniques, and developed during investigations as part of the US BW program at Camp Detrick, Frederick, Maryland.Footnote11
The advantages of the Henderson apparatus included its simple design and operation, the consistency of results obtained with experimental animals, and the ease with which any desired aerosol concentration could be established and assessed. The apparatus was also safe to use within the limits required by the experimental staff. However, the researcher was limited to being able to produce clouds of single organisms; the aerosol production method could damage sensitive organisms; and only a limited number of exposures could be carried out at once. The apparatus was used from 1942 until the late 1960s with few major changes, although some were implemented to improve its operation including instrumentation, stability, and ease of use. By 1963, the US Army Biological Laboratories at Fort Detrick had highlighted the Henderson apparatus as a safe and simple means of conducting significant aerobiological research.Footnote12
Research workers carried out some modifications during the 1960s to facilitate experimental infection with a range of different species and increased number of replicates. Two different versions of the Henderson apparatus were offered commercially in the United States, by S. Blickman, Inc., in Weehawken, New Jersey. One model recirculated air; the other used laboratory service air and vacuum supplies with no recirculation. Both models were engineered to be compatible for use within microbiological containment cabinets.Footnote13
The Henderson apparatus underwent a major alteration in the 1960s to add humidity control of the air that flows from the “spray” (or mixing) tube so that the apparatus could generate and condition aerosols with respect to humidity.Footnote14 This was required to enable the study of aerosolized microorganisms at different relative humidities using other devices such as the rotating drum.Footnote15 A rotating (or Goldberg) drum allows the survival of aerosolized microorganisms to be followed over extended time periods. The rotation of the drum keeps the aerosolized microorganisms suspended in the aerosol state. The changes that were required to achieve operation of the Henderson apparatus between 20 and 95 percent relative humidity at ambient temperatures from 0 to 37°C led to an increase in air flow through the spray tube. The water content of air at 0°C and 20 percent relative humidity is 0.97 grams per cubic meter; the single-jet collison spray had a nominal output of 0.77 grams per minute of water; a flow rate of 66 liters per minute is minimal. The higher flow rate, even at room temperature, allowed the use of a three-jet collison spray, which in turn increased the aerosol concentration and reduced the vulnerability of the apparatus to jet blockage (three jets instead of a single jet).Footnote16 Wheels were added to provide mobility, to ensure greater flexibility of use. shows a general view of the mobile Henderson apparatus.
Sampling aerosols
Sampling of aerosols during work with the original Henderson apparatus made use of a glass impinger.Footnote17 In an impinger, the air (or the gas stream to be sampled) is drawn using a vacuum through a collecting fluid (e.g., water, buffer solution, etc), and particles leave the airstream to be collected by high-speed impaction into the fluid. Most impingers are constructed from glass with a single collecting chamber and a vacuum attached through a side arm. Air to be sampled is drawn through a curving intake tube followed by an impinging jet. When the drop in pressure across the jet becomes a minimum of half an atmosphere,Footnote18 the flow through the jet attains the speed of sound (i.e., becomes sonic) and therefore rate limited (i.e., once the air flow through the jet is sonic, a greater flow rate cannot be achieved—the jet acts as a critical orifice). Following calibration, the impinger does not have to be monitored by a flowmeter, provided the pressure drop across the jet is maintained at half an atmosphere or greater. Impingers are compact, relatively inexpensive, and may be repeatedly sterilized for reuse. Sample fluid can be serially diluted and inoculated onto different microbiological growth media, allowing optimum growth conditions for the organisms of interest. Extreme ranges of aerosol concentration are dealt with via the serial dilution of sample fluid. Particle-retention efficiency is very high: effectively all particles down to 0.5 micrometers (µm) are trapped by the collection fluid, giving a measure of the number of viable microorganisms in the aerosol, rather than the number of infective particles.Footnote19
In the original design, the distance between the jet and the base of the sampler was 4 mm, which led to the air displacing the impingement liquid and allowing the particles to hit the glass base of the impinger. The design was acceptable for spores (e.g., B. anthracis), but the high-speed impact into the glass base damaged some vegetative organisms, such as Serratia marcescens.Footnote20
Alternative designs were produced, such as the Porton raised all-glass impinger, where the jet was raised to 30 mm above the base of the sampler (AGI-30).Footnote21 The modification increased the sampling efficiency for viable cells by reducing the impingement velocity of the particles, thus reducing the damage caused by impact with the base of the sampler and allowing the surviving cells to successfully grow on the microbiological growth medium. The AGI-30 was adopted as a reference sampler for the collection of viable airborne microorganisms and is still widely used.Footnote22 shows a comparison of contemporary all-glass impingers (AGI-4 and AGI-30) manufactured by Ace Glass, Inc. (Vineland, NJ).
An additional aerosol sampler, the Porton pre-impinger, was designed to collect viable aerosols by simulating the deposition in the upper respiratory tract. The particle retention of the pre-impinger was similar to that of the nasal passages. When the combination of a pre-impinger and an impinger are used together, they provide information on the material retained in the upper respiratory tract (via pre-impinger data) and that which passes into the lungs (via the impinger data).Footnote23 This provides useful information on the distribution of particles from a polydisperse aerosol cloud. The pre-impinger is connected in front of an impinger. It is a spherical glass bulb, half filled with an aerosol-collecting liquid with an air-intake hole in the front of the glass sphere. shows a pre-impinger attached to an AGI-4. The pre-impinger was found to have very high impingement efficiency in relation to the velocity of the incoming air jet. The liquid level was not critical to the efficiency. This was due to the double impingement on the surface of the liquid, first by the jet of air and second by the tight vortex at the meniscus of the liquid. Pre-impingers were used for aerosol trials by the United Kingdom and the United States.Footnote24
Field trials
Field trials using pathogenic agents were carried out during and after World War II. The research was concerned with understanding the survival of “infectious clouds” in the environment and their capacity to cause effects downwind. It was believed that releasing pathogens in the open air was crucial to understanding the vulnerability and risks of a BW attack. The United Kingdom carried out field trials on Gruinard Island, situated 1 km from the mainland in Gruinard Bay, Ross and Cromarty, on the northwest coast of Scotland. The trials determined the feasibility of producing lethal effects, via an aerosol formed by the explosion of a modified aircraft bombFootnote25 or small bombletFootnote26 containing a suspension of B. anthracis spores.
After World War II, the United Kingdom planned and carried out a series of BW trials at sea.Footnote27 After the first sea trial, Operation Harness, different types of trials were suggested including: trials on land with unit bombs charged with simulants; larger trials with simulants; and trials at sea with toxic agents.Footnote28 The results of the series of sea trials showed that aerosols of pathogens did not survive as well in the field as they did during experiments carried out in enclosed holding conditions in the laboratory.Footnote29
These field trials concluded that aerosols generated by both explosive and spray releases were feasible and posed a potential risk to a population if attacked. Operation Negation, which took place in late 1954/early 1955, was the last sea trial carried out using open-air release of pathogens. This was due to a changing political climate, coupled with the cost of such an operation, as well as the decision in the late 1950s to abandon the UK search for a retaliatory BW capability.Footnote30 One of the main points learned from the work was the need to understand the critical environmental parameters that could induce deleterious effects on the pathogens; these studies would require a controlled laboratory environment. Further BW defense field trials in the 1960s and later were conducted using BW simulants. A BW simulant is a non-pathogenic microorganism (or non-toxic substitute, e.g., albumin or ovalbumin) that mimics the properties and behavior of the BW agent that it is used to resemble without the pathogenic (or toxic) properties.
Laboratory research
Laboratory research investigated the effect of humidity, using a rotating-drum method devised and reported by Leonard J. Goldberg at the Naval Biological Laboratory in the United States.Footnote31 These experiments allowed the creation of humidity-survival profiles for pathogens of concern. The data from the experiments were dual use; they would allow the risk from a BW aerosol attack to be better understood. However, the data could be used to identify the optimum relative humidity for use of a particular BW agent. These investigations were extended in time, as there were instances where some pathogenic species showed a dissociation between viability and infectivity as bacterial populations aged in the aerosol state. With this knowledge in mind for a single parameter change, work focused on other attributes that reduced the viability and also capacity of these species to initiate infection post-aerosolization and transport within the air column. This information would be useful to understanding the risks of BW use. During this investigation, an early assessment of spray-fluid composition on the survival of these “clouds” took place. The simple addition of sugars to the spray fluid increased survival, whereas re-suspending the organisms in water reduced survival,Footnote32 as did the preliminary assessments of freeze-dried preparations.Footnote33
At the same time, other researchers investigated the effect of particle size on the respiratory infectivity of bacterial species. The relationship between dose response and pathogenesis to different particle size (and as such organism loading within those particles) was studied in infection models of B. anthracis,Footnote34 Brucella suis,Footnote35 and Pasturella pestis (Yersinia pestis).Footnote36 Each one of the experiments showed that the dose required to cause an effect increased as the particle size increased and that, with larger particles, the initial disease involved the upper respiratory tract. Understanding the pathogenesis of the diseases, following the initiation of infection in the upper respiratory tract, was important, since the characteristics of the infection and any signs/symptoms might be different. By reducing the hazard, the potential was still apparent, as these pathogens were able to initiate infection throughout the respiratory system. This work was significant, as previous research and offensive programmes had concentrated solely on the production of small 1 µm droplets in order to deliver microorganisms to the deep lung.
Laboratory experiments were proving useful in dissecting phenomena that were crucial to understanding what had been observed in the previous large-scale sea and field trials, but there was a need to elucidate the relationship between laboratory results and what happened outside in the environment. The discovery of the microthread technique, which used spider-web threads to trap particles containing bacterial cells, made a significant contribution to aerosol assessment in the late 1960s.Footnote37 Captured simulant species were subjected to outside air and conditions and were used to qualify laboratory results. The work began dissecting other phenomenon, including the effect of wind direction on survival, suggesting the existence of a “germicidal pollutant.” The new phenomenon was given the name “open-air factor” (OAF), and was a discovery of magnitude in the aerobiological field, as it reduces the survival of some microorganisms.Footnote38 The ability to elucidate OAF in the laboratory was thwarted, since the effect was not observed indoors, which led to design alterations of an existing aerosol test sphere (167,000 liters in volume; ) to allow the study of outdoor air indoors.Footnote39 The experiments suggested that ozonized olefins could be partially responsible for the phenomenon of OAF.Footnote40 Current studies within the field of atmospheric chemistry are further investigating the complex secondary organic aerosols and their effect on aerosols and climate.Footnote41
A reorganization and change of location
BDP and MRE, under the direction of Paul Fildes and David Henderson, had driven the field of aerobiology for many years. Aerobiology was a young science at the inception of research at BDP, requiring a multidisciplinary scientific team of engineers, physicists, and biologists designing and carrying out experiments, as well as designing and building the equipment required to accomplish the work. For nearly thirty years, there was debate within the UK government over which department should oversee MRD and its successors. During the mid to late 1960s, several discussions took place around funding and where MRE should be placed within the government, owing to Ministry of Defence (MOD) cutbacks. In 1967, a memorandum relating to a Defence Expenditure Study on Research and Development considered the UK research program on chemical and biological warfare.Footnote42 The document reviewed chemical- and biological-warfare research and raised points that should be taken into account on future arrangements for MRE. It was stated that, if the MRE closed, it would take five to ten years to re-establish the capability, with the correct expertise and infrastructure, at the 1967 levels. If MRE became a civil microbiological research establishment, a shorter time, three to five years, would be required.
In the end, the government decided that the Public Health Laboratory Service (PHLS) would take responsibility for the MRE. In April 1979, the MRE building became the PHLS Centre for Applied Microbiology and Research (CAMR). As the strategic direction of the establishment changed, BW defense-aerobiology research ceased. A small team of microbiologists was transferred to the Chemical Defence Establishment (CDE, the sister establishment to MRE) to provide a focus for BW defense expertise.Footnote43 The transferred staff formed the Defence Microbiology Division (DMD). Over the next ten to fifteen years, DMD (and its successors) developed from initially providing knowledge, advice, and expertise within the MOD to carrying out experimental investigations to provide the evidence base to support wider advice, as well as to develop medical countermeasures and diagnostics.Footnote44
The aerobiological expertise that grew within the MRE and its predecessor organizations helped increase safety within their laboratories and those of the wider civilian world. Examples include investigations into the design of microbiological safety cabinets,Footnote45 their use in preventing laboratory-acquired infections,Footnote46 and general safety in high-risk laboratories.Footnote47
Contemporary aerobiology
Aerobiological investigations continue to support the UK biological-defense program at the Defence Science and Technology Laboratory (Dstl) at Porton Down. Aerobiology is closely linked to all aspects of the defense program: from the hazard assessment of a BW agent, design of aerosol sampling, respiratory and other protective equipment and procedures, and efficacy testing of candidate medical countermeasures, through to technical advice, assistance, and support for arms control.
Dstl has developed its aerobiology and microbiological containment capabilities to enable work at biosafety Level 4 labs that work with highly pathogenic viruses.Footnote48 Investigations carried out between 2005 and 2010 studied the survival of filoviruses in liquids, on surfaces, and in aerosols.Footnote49 It was shown that both ebolavirus and marburgvirus can survive for long periods (forty-six days) in both guinea-pig sera and cell-culture media, and can be recovered from plastic and glass surfaces stored at low temperatures (+4°C) for over three weeks.Footnote50
Dstl has investigated the survival of ebolavirus and marburgvirus in aerosols using two different methods: a dynamic aerosol in a rotating drum and a captured aerosol using the spider-web-microthread technique. Results were comparable, and showed that ebolavirus and marburgvirus were moderately stable, with biological decay rates of 3.5–4 percent per minute (% min–1).Footnote51 The survival data, under different conditions, provided a basis to inform risk assessments and manage exposure to filoviruses.
The United Kingdom actively encourages publication of work in the scientific literature. This policy is promulgated in the UK Confidence Building Measure Returns.Footnote52 The information created at Dstl was critical and used to inform high-level decision making for the inactivation of ebolavirus during the West Africa outbreak of 2014–16. Additional investigation, carried out to support the ebola outbreak response, studied the rapid inactivation of ebolavirus to enable high-throughput testing of clinical samples. The data that were generated provided the evidence base for the selection of the process for use in the field. This was published in 2015.Footnote53
Legislation and nonproliferation
The UK investigations carried out in the field of aerobiology during both the offensive (1940 to late 1950s) and defensive (1940 to present) BW program are an ideal example of dual-use technology and information; the output could be used for both civilian and military purposes. The pivotal international legislation linked to BW is the Biological and Toxin Weapons Convention (BWC) that entered into force in March 1975.Footnote54 This was the first multilateral disarmament treaty that banned an entire class of weapons. The United Kingdom is a depositary country along with the United States and Russia.Footnote55 The Australia Group is an informal group of countries which, via the harmonization of their export controls, supports the BWC by striving to ensure that exports do not assist in the development of chemical or biological weapons.Footnote56
Continuing stewardship in the arms- and export-control arenas is essential to countering chemical and biological proliferation risks that will continue to appear with the global spread of new and existing science and technology. The United Kingdom has a long history of commitment to strengthening international treaties and supporting the monitoring and verification of international compliance, whilst ensuring that the United Kingdom meets its obligations in the export of sensitive goods. The UK National Counter Proliferation Strategy provides a framework for UK counterproliferation activity. It has the overall aim of preventing the spread (or further development) of chemical, biological, radiological, and nuclear capability or advanced military technologies that might threaten UK interests or regional stability.Footnote57
Notes
1 National Archives, Kew, WO 188/764, Bacterial Warfare: Russia, 1924–26, Enclosure 1: Russia Bacteriological, September 28, 1926; J.R. Walker, “The 1925 Geneva Protocol: Export Controls, Britain, Poland and Why the Protocol Came to Include ‘Bacteriological’ Warfare,” Harvard Sussex Program Occasional Paper Issue 05, June 2016; Martin Hugh-Jones, “Wickham Steed and German Biological Warfare Research,” Intelligence and National Security, Vol. 7 (1992), pp. 379–402.
2 National Archives, Kew, WO 188/648, Committee of Imperial Defence Sub-committee on Bacteriological Warfare, Note by the Joint Secretaries, November 4, 1936.
3 National Archives, Kew, CAB 104/234, letter Hankey to Elliot, August 9, 1940.
4 National Archives, Kew, WO 188/653, BW(40) 35, War Cabinet, Bacteriological Warfare Committee, “Further Experiments on Methods of Spread of Bacteria,” Note by the Chairman, September 23, 1940.
5 National Archives, Kew, DEFE 55/107, P. Fildes, G.P. Gladstone, D.W. Henderson, and D.D. Woods, “The Use of Anthrax against Cattle in Warfare,” BDP 5, April 28, 1941; G.B. Carter and G.S. Pearson, “British Biological Warfare and Biological Defence, 1925–45,” in Erhard Geissler and John Ellis van Courtland Moon, eds., Biological and Toxin Weapons: Research, Development and Use from the Middle Ages to 1945 (New York: Oxford University Press, 1999), pp. 178–81; National Archives, Kew, DEFE 55/149, P. Fildes, “Penicillin Treatment of N Spores in Mice,” BDP 47, October 5, 1944.
6 MRD, 1946–57; MRE, 1957–79.
7 W.E. Collison, Inhalation Therapy (London: Heinemann, 1935), <https://ia800302.us.archive.org/2/items/inhalationtherap031595mbp/inhalationtherap031595mbp.pdf>.
8 National Archives, Kew, DEFE 55/112, D.W. Henderson and D.D. Woods, “A Laboratory Apparatus for Setting up ‘Clouds’ with a Report on Experiments with B. anthracis,” BDP 10, April 2, 1942.
9 National Archives, Kew, DEFE 55/132, D.W. Henderson, “A Note on an Improved Type of Laboratory Apparatus for Producing Clouds of ‘Toxic Dusts’,” BDP 30, September 16, 1943.
10 D.W. Henderson, “An Apparatus for the Study of Airborne Infection,” Journal of Hygiene, Vol. 50 (1952), pp. 53–68.
11 T. Rosebury, Experimental Air-Borne Infection (Baltimore: Williams & Wilkins, 1947), pp. 11–18. Note that Camp Detrick, established 1943, was renamed Fort Detrick in 1956. See N.M. Covert, Cutting Edge: A History of Fort Detrick, Maryland, 1943–1993 (Frederick, MD: Public Affairs Office, Headquarters US Army Garrison, Fort Detrick ).
12 O.T. Miller and G.G. Gremillion, “Respiratory Exposure of Animals to Microorganisms with the Henderson Appartus,” Technical Manuscript 39, Safety Division, US Army Biological Laboratories, Fort Detrick, Frederick, MD, April 1963, AD402787, <https://apps.dtic.mil/dtic/tr/fulltext/u2/402787.pdf>.
13 Miller and Gremillion, “Respiratory Exposure of Animals to Microorganisms with the Henderson Appartus”; J.V. Jemski and G.B. Phillips, “Aerosol Challenge of Animals,” Technical Manuscript 144, US Army Biological Laboratories, Fort Detrick, Frederick, MD, September 1964, AD450639, <https://apps.dtic.mil/dtic/tr/fulltext/u2/450639.pdf>.
14 H.A. Druett, “A Mobile Form of the Henderson Apparatus,” Journal of Hygiene, Vol. 67 (1969), pp. 437–49, <www.cambridge.org/core/services/aop-cambridge-core/content/view/D069D3E85AD4409CD6CFF73FE052091E/S0022172400041851a.pdf/mobile_form_of_the_henderson_apparatus.pdf>.
15 L.J. Goldberg, H.N.S. Watkins, E.E. Boerke, and M.A. Chatigny, “The Use of a Rotating Drum for the Study of Aerosols over Extended Periods of Time,” American Journal of Hygiene, Vol. 68 (1958), pp. 85–93.
16 K.R. May, The Collison Nebulizer: Description, Performance and Application,” Aerosol Science, Vol. 4 (1973), pp. 235–43.
17 National Archives, Kew, DEFE 55/112, figure 3.
18 A commonly used unit of pressure is the atmosphere (atm). Standard atmospheric pressure is called 1 atm of pressure and is equal to 760 mm Hg and 101.3 kPa at sea level.
19 K.R. May and G.J. Harper, “The Efficiency of Various Liquid Impinger Samplers in Bacterial Aerosols,” British Journal of Industrial Medicine, Vol. 14 (1957), pp. 287–97.
20 Ibid.
21 Ibid.
22 P.S. Brachmann, R. Ehrlich, H.F. Eichenwald, V.J. Cabelli, T.W. Kethley, S.H. Madin, J.R. Maltman, G. Middlebrook, I.D. Morton, I.H. Silver, and E.K.Wolfe, “Standard Sampler for Assay of Airborne Micro-organisms,” Nature, June 12, 1964, p. 1295.
23 National Archives, Kew, DEFE 55/247, K.R. May and H.A. Druett, “Pre-impinger: A Selective Aerosol Sampler,” January 1, 1953 to December 31, 1953, Microbiological Research Department Report 4; K.R. May and H.A. Druett, “The Pre-impinger: A Selective Aerosol Sampler,” British Journal of Industrial Medicine, Vol. 10 (1953), pp. 142–51.
24 Anon., “BW Field Evaluation of the EDO Spray System Phase A, Trials 1–4,” August 1, 1959, DPG-TR-273, <https://apps.dtic.mil/dtic/tr/fulltext/u2/596100.pdf>.
25 National Archives, Kew, DEFE 55/118, D.W. Henderson and D.D. Woods, “The Dispersion of Anthrax Spores from the 30lb (LC) Aircraft Bomb,” BDP Report No. 16, August 17, 1942.
26 National Archives, Kew, DEFE 55/126, P. Fildes, “Bomb Aircraft L.C., 4-lb. HE/CHEM. TYPE F MARK 1 for dispersal of N spores,” BDP Report No. 24, August 4, 1943.
27 P.M. Hammond and G.B. Carter, From Biological Warfare to Healthcare: Porton Down, 1940–2000 (Basingstoke, UK: Palgrave, 2002), pp. 19–39.
28 National Archives, Kew, WO195/10483, “Operation Harness. Opinions and Recommendations of the Conference of Technical Representatives of the United States, Canada and the United Kingdom. Biological Research Advisory Board, Advisory Council on Scientific Research and Technical Development, Ministry of Supply,” BRB 63, received July 12, 1949.
29 National Archives, Kew, DEFE 55/405, D.W. Henderson, “Biological Warfare Research 1945–1956: A Summary,” MRE/989/7, April 10, 1957.
30 United Kingdom, Confidence Building Measure Return for 2011 (Covering Data for 2010) for the Convention on the Prohibition of the Development, Production and Stockpiling of Bacteriological (Biological) and Toxin Weapons and Their Destruction, April 10, 1972, 2011, 45–47, <www.unog.ch/80256EDD006B8954/%28httpAssets%29/009540B24174AC38C12578930057FF00/$file/BWC_CBM_2011_United+Kingdom.pdf>.
31 Goldberg et al., “The Use of a Rotating Drum for the Study of Aerosols over Extended Periods of Time.”
32 C.S. Cox, “The Survival of Escherichia coli Sprayed into Air and into Nitrogen from Distilled Water and from Solutions of Protecting Agents, as a Function of Relative Humidity,” Journal of General Microbiology, Vol. 43 (1966), pp. 383–99.
33 National Archives, Kew, DEFE 55/405.
34 H.A. Druett, D.W. Henderson, L. Packman, and S. Peacock, “Studies on Respiratory Infection. I. The Influence of Particle Size on Respiratory Infection with Anthrax Spores,” Journal of Hygiene, Vol. 51 (1953), pp. 359–71.
35 H.A. Druett, D.W. Henderson, and S. Peacock, “Studies on Respiratory Infection. III. Experiments with Brucella suis,” Journal of Hygiene, Vol. 54 (1956), pp. 49–57.
36 H.A. Druett, J.M. Robinson, D.W. Henderson, L. Packman, and S. Peacock, “Studies on Respiratory Infection. II. The Influence of Aerosol Particle Size on Infection of the Guinea-Pig with Pasteurella pestis,” Journal of Hygiene, Vol. 54 (1956), pp. 37–48.
37 K.R. May, H.A. Druett, “A Microthread Technique for Studying the Viability of Microbes in a Simulated Airborne State,” Journal of General Microbiology, Vol. 51 (1968), pp. 353–66.
38 H.A. Druett and K.R. May, “Unstable Germicidal Pollutant in Rural Air,” Nature, Vol. 220 (1968), pp. 395–96.
39 A.M. Hood, “An Indoor System for the Study of Biological Aerosols in Open Air Conditions,” Journal of Hygiene, Vol. 69 (1971), pp. 607–17; National Archives, Kew, WO188/2529, “Climatic Testing Facilities and the Spherical Bursting Chambers at CDE, Porton (Includes PTP 496, 497 & 637,” February 1 to April 30, 1958.
40 A.M. Hood, “Historical Review: The Effect of Open-Air Factors on the Virulence and Viability of Airborne Francisella tularensis,” Epidemiology and Infection, Vol. 137 (2009), pp. 753–61.
41 M. Shrivastava, C.D. Cappa, J. Fan, A.H. Goldstein, A.B. Guenther, J.L. Jimenez, C. Kuang, A. Laskin, S. Martin, L.N. Ng, T. Petaja, J.R. Pierce, P.J. Rasch, P. Roldin, J.H. Seinfeld, J. Shilling, J.N. Smith, J.A. Thornton, R. Volkamer, J. Wang, D.R. Worsnop, R.A. Zaveri, A. Zelenyuk, and Q. Zhang, “Recent Advances in Understanding Secondary Organic Aerosol: Implications for Global Climate Forcing,” Reviews of Geophysics, Vol. 55 (2017), pp. 509–59.
42 National Archives, Kew, DEFE 11/660. A.H. Cottrell, “CA (Studies) Research Programme on Chemical and Biological Warfare,” February 8, 1967, AHC/72/67.
43 Hansard, February 15, 1979, Col. 1327. <https://hansard.parliament.uk/commons/1979-02-15/debates/9859672d-39b2-423e-985e-f8b44d782c5d/PublicHealthLaboratoryServiceBillLords>.
44 G.B. Carter, Chemical and Biological Defence at Porton Down 1916–2000 (London: Stationery Office, 2000), pp. 96–107, 138–39.
45 H.M. Darlow, “The Design of Microbiological Safety Cabinets,” Chemistry and Industry, November 11, 1967, pp. 1914–16.
46 C.G.T. Evans, R. Harris-Smith, and J.E.D. Stratton, “The Use of Safety Cabinets for the Prevention of Laboratory Acquired Infection,” in Safety in D.A. Shapton and R.G. Board, eds., Microbiology (London: Academic Press, 1972), pp. 21–36.
47 H.M. Darlow, “Safety in the Animal House,” Laboratory Animals, Vol. 1 (1967), pp. 35–42.
48 S.J. Smither and M.S. Lever, “A Review of Filovirus Work and Facilities at the Defence Science and Technology Laboratory Porton Down,” Viruses, Vol. 4, No. 8 (2012), pp. 1305–17.
49 T.J. Piercy, S.J. Smither, J.A. Steward, L. Eastaugh, and M.S. Lever, “The Survival of Filoviruses in Liquids, on Solid Substrates and in a Dynamic Aerosol,” Journal of Applied Microbiology, Vol. 109 (2010), pp. 1531–39; S.J. Smither, T.J. Piercy, L. Eastaugh, J.A. Steward, and M.S. Lever, “An Alternative Method of Measuring Aerosol Survival Using Spiders’ Webs and Its Use for the Filoviruses,” Journal of Virological Methods, Vol. 177 (2011), pp. 123–27.
50 Piercy et al., “The Survival of Filoviruses in Liquids, on Solid Substrates and in a Dynamic Aerosol.”
51 Ibid.; Smither et al., “An Alternative Method of Measuring Aerosol Survival Using Spiders’ Webs and Its Use for the Filoviruses.”
52 UK Confidence Building Measure Return for 2019 (covering data for 2018) for the Convention on the Prohibition of the Development, Production and Stockpiling of Bacteriological (Biological) and Toxin Weapons and Their Destruction, April 10, 1972, submitted to the United Nations April 2019.
53 S.J. Smither, S.A. Weller, A. Phelps, L. Eastaugh, S. Ngugi, L.M. O’Brien, J.A. Steward, S.G. Lonsdale, and M.S. Lever, “Buffer AVL Alone Does Not Inactivate Ebola Virus in a Representative Clinical Sample Type,” Journal of Clinical Microbiology, Vol. 53 (2015), pp. 3148–54.
54 Convention on the Prohibition of the Development, Production and Stockpiling of Bacteriological (Biological) and Toxin Weapons and on Their Destruction, <www.unog.ch/80256EDD006B8954/(httpAssets)/C4048678A93B6934C1257188004848D0/$file/BWC-text-English.pdf>.
55 Instruments of ratification and accession to the convention are deposited with one or more of the three depositary governments of the Convention.
56 Australia Group, “Introduction,” <https://australiagroup.net/en/introduction.html>.
57 UK National Counter Proliferation Strategy to 2020, <https://assets.publishing.service.gov.uk/government/uploads/system/uploads/attachment_data/file/510716/National_Counter_Proliferation_Strategy_to_2020_-_updated_24_March.pdf>.