Abstract
Objective
To understand the progression in parameters of functional electrical stimulation (FES) cycling dosage (including duration, velocity, stimulation amplitudes, power output), and the resulting changes in muscle mass early after acute spinal cord injury (SCI).
Methods
Three participants, 24–38 years old, with neurological injury level C4–T4, severity AIS (American Spinal Injury Association Impairment Scale) A–C, started FES cycling 16–20 days post injury while admitted at a level-1 trauma center in Canada, and continued for 8–13 weeks in a rehabilitation hospital. They performed three sessions/week of 15–45 min FES cycling, supine or sitting. FES parameters, cycling performance, and muscle cross-sectional area (CSA) in thighs and calves were measured every 2 weeks.
Results
Progression in power output, but not in session duration, was limited in two participants who experienced stimulation-associated referred pain or apprehension, requiring limitation of stimulation amplitudes for up to 65 days after the start of FES cycling. Participants started with 15 min cycling at 20 RPM with no resistance (0 W), and progressed to 30–45 min at 30 RPM producing 8.8–19.0 W average power/session after 2–3 months. Initially, muscle CSA decreased in all 3 participants (up to 16% after 6 weeks), and recovered later after a variable period of FES cycling (up to 16% at 13.3 weeks).
Conclusion
Progression of FES cycling in the first 3 months after injury required a highly individualized approach, guided by participant response, rather than standardized increments in stimulation intensity or duration. Changes in muscle CSA did not always correspond with the dose of FES cycling.
Introduction
Early exercise training after spinal cord injury (SCI) may potentially prevent early neuro-musculoskeletal changes such as muscle atrophyCitation1 and spasticity,Citation2 and potentially harness plasticity to improve functional recovery.Citation3–5 This may be achieved through the use of functional electrical stimulation (FES) cycling, which has been successfully used in chronic SCI, with demonstrated benefits on cardiorespiratory status, muscle strength, body composition, bone health, and functional performance.Citation6–12 However, this may be challenging in acute SCI (<6 months after injury) as recovery from the initial trauma, medical issues, spinal shock, and emotional adjustment might pose barriers to, or interfere with the application of FES cycling. Despite its potential impact, there is a paucity of research on the effects of FES cycling in the acute SCI population,Citation13–17 and the stimulation parameters and their progression have not been covered in detail in previous studies. The goal of this report is to document the progression in dosage parameters (cycling duration, velocity, stimulation amplitudes, power output), and the corresponding changes in muscle mass, in three people who participated in our feasibility study on early FES cycling after SCI. This case series aims to help us understand the timing and progression of FES cycling early after SCI, in order to prepare for a clinical trial.
Methods
Three participants started FES cycling 16–20 days post injury while in the acute care hospital, and continued for 8–13 weeks while in the rehabilitation hospital. Written consent was obtained prior to enrolment and the study was approved by the University Health Research Ethics Board.
FES cycling
Participants cycled supine in bed or sitting in a wheelchair with an RT300 Supine or SLSA FES bike (Restorative Therapies, Baltimore, MD, USA), while in the acute care or rehabilitation hospital respectively. Three to four muscle groups were stimulated through surface electrodes (5 × 9 cm and 7.5 × 10 cm, Axelgaard PALS electrodes, Fallbrook, CA, USA). Quadriceps and hamstrings were always stimulated, combined with glutaeus maximus and/or gastrocnemius. During the initial set-up, maximum stimulation amplitudes were set for each channel (using 300 µs pulse width, and 35 Hz frequency, which we considered our default settings) at a level that produced a small range of motion without any resistance, or at the maximum tolerable level if discomfort was experienced.
The RT300 control algorithm is based on maintaining a constant user-defined target speed, by adjusting stimulation levels (up to 100% of set maximum) and resistance. If FES-produced contractions were not strong enough to cycle with zero resistance, then motor support was activated to continue cycling for the predetermined session duration, with muscle stimulation continuing at 100%.
Participants performed three sessions per week, starting with 15 min FES cycling at 20 revolutions per minute (RPM) without resistance. Speed, duration, and stimulation amplitudes were increased over time as tolerated, while considering degree of muscle contraction, power output, and fatigue. Exercise tolerance was assessed by monitoring pain, discomfort, and patient confidence during cycling, as well as cycling biomechanics and changes in heart rate, blood pressure, and oxygen saturation. Furthermore, skin integrity was monitored before and after cycling. The goal was to cycle at the maximal power output at 30–35 RPM. The FES cycling parameters we used were guided by the standard and intensive care unit (ICU) templates provided by Restorative Therapies for RT300 bilateral leg therapy (with minor deviation from their default pulse width and frequency, respectively 250 µs and 40 Hz).
Data collection
All FES parameters were stored for each session, including maximum stimulation levels, pulse width, frequency, and average stimulation. The average stimulation is the average charge delivered to all channels per session. Cycling performance per session was measured by average power produced, average resistance, session duration (active time in minutes) and time off motor support.
Muscle cross-sectional area (CSA) of the thigh and shank were calculated based on circumference measurements with correction for subcutaneous fat-layer thickness (based on skinfold thickness, method adapted from Jones).Citation18 Circumferences and skinfolds were measured (mean of three repetitions) with a tape measure and caliper at mid-thigh and at the largest diameter of the calf. Measurements were taken every two weeks and normalized to baseline (relative changes).
Results
Participant P1 (age 25, T4 AIS B) started FES cycling 17 days post injury, was transferred to the rehabilitation hospital 25 days post injury, and performed a total of 13.4 weeks of FES cycling (36 sessions, ). P1 experienced referred pain in the area of the injured spinal cord and in the groin during FES cycling, which was caused by the electrical stimulation of the quadriceps and gluteals, respectively. This stimulation-associated pain limited stimulation amplitudes at baseline (), and up to 65 days, requiring motor support up to 7.1 weeks (). After one week of FES cycling, P1’s cycling was interrupted due to transfer to the rehabilitation hospital. At 4.7 weeks, FES cycling was restarted with 15-minute low-intensity sessions. From 6 to 10 weeks, the dosage was increased to 45-minute sessions, with up to 22 µC average stimulation, producing up to 13 W (Fig. 1(B)). Due to a sudden increase in spasticity experienced during daily function (of unknown reason), stimulation intensity was reduced for one week, with average power dropping to 4.2 W. Stimulation amplitudes were ramped up again, resulting in 13.9 W average power at 13 weeks (“best” session, ). P1 showed small changes in muscle CSA in the first six weeks of low-intensity FES cycling ((A)), but thigh muscle CSA started increasing after six weeks, as stimulation intensity and session duration were increased. Overall, P1 lost muscle mass in calf muscles, which were not stimulated. At 13.3 weeks, muscle CSA was substantially increased in right thigh, and decreased in right and left calves.
Figure 1 FES cycling progress and muscle cross-sectional area changes. DPI, days post injury. CSA, cross-sectional area. Changes from baseline are expressed as a percentage. The result section in the text describes the average power production. For purpose of comparison with other studies, resistance data are also provided in the figure. P1 showed small changes in muscle CSA in the first 6 weeks of low-intensity FES cycling. Overall, P1 gained muscle mass in the stimulated thigh muscles (quadriceps and hamstrings), and lost muscle mass in the non-stimulated calf muscles. At 13.3 weeks, muscle CSA was increased in right and left thighs (16% and 2%, respectively), and decreased in right and left calves (6% and 13%). P2 showed an initial loss in muscle CSA at 2.1 weeks (about 4–5% in left/right calves, and 5–11% in thighs), but regained about 6–7% in calf muscle CSA, and 9–12% in thigh muscle CSA after 6.1 and 8.7 weeks of FES cycling, respectively. P3 showed loss in muscle CSA up to 6 weeks (about 11–13% in calves, and 15% in thighs), and regained some muscle CSA at 8 weeks in calves and left thigh. The sudden apparent increase in left thigh CSA at 4 weeks was due to an increase in thigh circumference with unknown reason. The sudden apparent increase in right thigh CSA at 8 weeks was due to swelling from a diagnosed heterotopic ossification in the right proximal medial thigh. FES cycling was discontinued at 8 weeks, resulting in the decreases in CSA at the 12-week assessment.
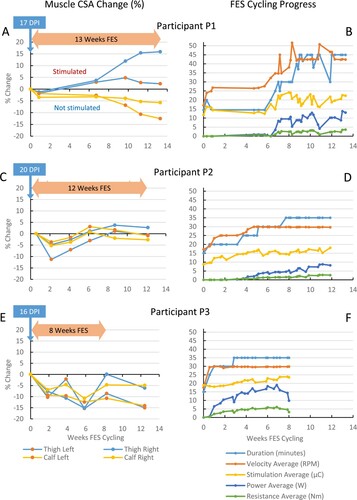
Table 1 Participant information, start and duration of FES cycling.
Table 2 FES stimulation parameters for first and best session.
Table 3 FES cycling performance for first and best session, and progress to OffMotor and voluntary cycling.
Participant P2 (age 38, C4 AIS C) started FES cycling 20 days post injury, was transferred to the rehabilitation hospital 39 days post injury, and performed a total of 11.9 weeks of FES cycling (32 sessions, ). P2 initially had limited trunk control and was not comfortable with forceful leg and trunk movements caused by FES, limiting stimulation amplitudes to 22–35 mA (). P2 showed apprehension several times later when asked if he was OK with an increase in stimulation amplitudes. Unlike the other participants, P2 had less fused muscle contractions at 35 Hz, therefore stimulation frequency was increased to 40 Hz. P2 required motor support during the first five weeks (). Duration and speed were increased in four steps (20–25–30–35ʹ min and 25–30 RPM) with 5–12 sessions per step. The average stimulation level was gradually increased during each step ((D)), with amplitudes per channel shown in . P2 also received calf muscle stimulation. P2 reached 8.8 W average power during the best session at 11.1 weeks, and was able to produce some power with voluntary muscle contractions (). P2 showed an initial loss in muscle CSA at 2.1 weeks, but later regained calf and thigh muscle CSA, peaking at 6.1 and 8.7 weeks, respectively ((C)).
Participant P3 (age 24, T4 AIS A) started FES cycling 16 days post injury, was transferred to the rehabilitation hospital 22 days post injury, and performed a total of eight weeks of FES cycling (20 sessions, ). Although P3 had no discomfort with higher stimulation amplitudes, initial limits of 60–70 mA across all channels were set, producing moderate contraction levels (). P3 was able to cycle without motor support at 1.6 weeks. Dosage was increased in three steps up to 35 min at 30 RPM at week 2.9 ((F)). Stimulation levels were gradually and almost continually increased, resulting in a steady increase in power production. P3 produced 5.7 W at one week, and reached 19 W average power during the best session at 6.9 weeks (, stimulation amplitudes in ). P3 showed a loss in muscle CSA up to six weeks, and regained some muscle CSA at eight weeks in both calves and left thigh ((E)). The sudden apparent increase in left thigh CSA at four and eight weeks were respectively due to unknown reason and diagnosed heterotopic ossification in the unstimulated right proximal medial thigh. FES cycling was discontinued at eight weeks, resulting in decreases in CSA at the 12-week assessment.
Discussion
Publications on FES cycling typically report the ranges of stimulation and biking parameters used, without giving much detail about the progression over time in these parameters.Citation6–10 Furthermore, it is not always clear how the maximum stimulation amplitudes were determined. We determined maximum stimulation intensities per channel based on the response to stimulation in terms of pain and contraction level, judged by visual observation and palpation. Allowing stimulation amplitudes to go up to the maximum stimulator output of 140 mA could result in forceful contractions, potentially causing muscle injury. In P1 and P2, we found that stimulation-associated pain and apprehension required limitation of stimulation amplitudes for certain channels to relatively low levels (as low as 25 mA) and for up to two months after the start of FES cycling, limiting progression in FES intensity. Therefore, P3 with no pain limitation had a faster increase in power production compared to P1 and P2. None of the above-mentioned studies mentioned pain caused by FES as a limitation to stimulation and hence training intensity.
Rather than using predefined steps for progression based on power output (e.g. 6 W increase after three sessionsCitation13,Citation14), we found it more suitable to control stimulation amplitudes, which allows channel by channel adjustments, in order to balance muscle groups and left/right side. Stimulation amplitudes were increased in small increments, sometimes from session to session, especially during the first four weeks, as long as the participant did not experience any problems, such as pain, discomfort, increased spasticity, blood pressure issues during and after the FES cycling session. Later in the program, less frequent (every 3–5 sessions) increases in stimulation amplitudes were made.
Our data suggested that muscle CSA decreased initially in all three participants, and recovered later after a variable period of FES cycling. P2 showed a relative gain in muscle CSA after the first four weeks, during which FES cycling intensity was very low (up to 1 W average power). P3 on the other hand showed a relative gain only after the first eight weeks, during which average power had increased up to 19 W. This observation was opposite of what was expected. FES cycling was interrupted in P1, who “restarted” at 4.7 weeks, and progressed quickly to producing 12.9 W average power. Despite the delayed start, P1 showed the highest gain in muscle CSA with right thigh reaching a 16% increase above baseline at 13.3 weeks. It is unclear why the left thigh did not follow the same trend. Furthermore, in P1, muscle CSA decreased in the calf muscles which were not stimulated.
The small sample size of this case series is a limitation of the study, precluding generalization of the data and guidance of clinical practice. However, our study supports the feasibility of FES cycling early after SCI, and provides some insight about the application and progression in FES parameters that may inform other studies and future clinical trials.
Conclusion
The stimulation intensity for FES cycling early after SCI may be limited by pain and other factors inherent to the acuity of the injury, which play an important role in the progression strategy. The progression of the first three months of FES cycling after injury requires a highly individual approach, rather than using standardized increments in terms of stimulation intensity and duration. Overall, muscle CSA appeared to decrease in non-stimulated muscles, and increase in stimulated muscles after an initial period of muscle loss, but the changes did not always correspond with the dose of FES cycling. Delaying progression by four weeks did not appear to result in a smaller gain in muscle CSA.
We have applied the lessons learned from our feasibility study and are currently running a pilot clinical trial investigating the effects of early and delayed FES cycling in the first 6 months after SCI on neurorecovery and neuropreservation.
Disclaimer statements
Contributors: None.
Funding: This work was supported by the University of Alberta Endowed Spinal Cord Research Chair funding.
Conflict of interest: Authors have no conflict of interest to declare.
Acknowledgements
The authors wish to thank the Spinal Cord Injury Treatment (Northern Alberta) Society (SCITCS) for providing the FES cycling equipment, and the management teams and frontline clinicians at the University of Alberta Hospital and Glenrose Rehabilitation Hospital for their support.
References
- Castro MJ, Apple DF, Hillegass EA, Dudley GA. Influence of complete spinal cord injury on skeletal muscle cross-sectional area within the first 6 months of injury. Eur J Appl Physiol Occup Physiol 1999 Sep;80(4):373–8.
- Ditunno JF, Little JW, Tessler A, Burns AS. Spinal shock revisited: a four-phase model. Spinal Cord 2004;42(7):383–95.
- Battistuzzo CR, Callister RJ, Callister R, Galea MP. A systematic review of exercise training to promote locomotor recovery in animal models of spinal cord injury. J Neurotrauma 2012;29(8):1600–13.
- Norrie BA, Nevett-Duchcherer JM, Gorassini MA. Reduced functional recovery by delaying motor training after spinal cord injury. J Neurophysiol 2005;94(1):255–64.
- Fouad K, Krajacic A, Tetzlaff W. Spinal cord injury and plasticity: opportunities and challenges. Brain Res Bull 2011;84(4–5):337–42.
- Martin R, Sadowsky C, Obst K, Meyer B, McDonald J. Functional electrical stimulation in spinal cord injury: from theory to practice. Top Spinal Cord Inj Rehabil 2012;18(1):28–33.
- Hicks AL, Martin Ginis KA, Pelletier CA, Ditor DS, Foulon B, Wolfe DL. The effects of exercise training on physical capacity, strength, body composition and functional performance among adults with spinal cord injury: a systematic review. Spinal Cord 2011 Nov;49(11):1103–27.
- Sadowsky CL, Hammond ER, Strohl AB, Commean PK, Eby SA, Damiano DL, et al. Lower extremity functional electrical stimulation cycling promotes physical and functional recovery in chronic spinal cord injury. J Spinal Cord Med 2013;36(6):623–31.
- Bloomfield SA, Mysiw WJ, Jackson RD. Bone mass and endocrine adaptations to training in spinal cord injured individuals. Bone 1996 Jul;19(1):61–8.
- Frotzler A, Coupaud S, Perret C, Kakebeeke TH, Hunt KJ, Donaldson NdN, et al. High-volume FES-cycling partially reverses bone loss in people with chronic spinal cord injury. Bone 2008 Jul;43(1):169–76.
- Janssen TWJ, Pringle DD. Effects of modified electrical stimulation-induced leg cycle ergometer training for individuals with spinal cord injury. J Rehabil Res Dev 2008;45(6):819–30.
- Faghri PD, Glaser RM, Figoni SF. Functional electrical stimulation leg cycle ergometer exercise: training effects on cardiorespiratory responses of spinal cord injured subjects at rest and during submaximal exercise. Arch Phys Med Rehabil 1992;73(11):1085–93.
- Baldi JC, Jackson RD, Moraille R, Mysiw WJ. Muscle atrophy is prevented in patients with acute spinal cord injury using functional electrical stimulation. Spinal Cord 1998;36(7):463–9.
- Demchak TJ, Linderman JK, Mysiw WJ, Jackson R, Suun J, Devor ST. Effects of functional electric stimulation cycle ergometry training on lower limb musculature in acute sci individuals. J Sport Sci Med 2005;4(3):263–71.
- Galea MP, Panisset MG, El-Ansary D, Dunlop SA, Marshall R, Clark JM, et al. SCIPA switch-on: a randomized controlled trial investigating the efficacy and safety of functional electrical stimulation-assisted cycling and passive cycling initiated early after traumatic spinal cord injury. Neurorehabil Neural Repair 2017;31(6):540–51.
- Eser P, De Bruin ED, Telley I, Lechner HE, Knecht H, Stüssi E. Effect of electrical stimulation-induced cycling on bone mineral density in spinal cord-injured patients. Eur J Clin Invest 2003;33(5):412–9.
- Lai CH, Chang WHS, Chan WP, Peng CW, Shen LK, Chen JJJ, et al. Effects of functional electrical stimulation cycling exercise on bone mineral density loss in the early stages of spinal cord injury. J Rehabil Med 2010;42(2):150–4.
- Jones P, Pearson J. Anthropometric determination of leg fat and muscle plus bone volumes in young male and female adults. J Physiol 1969 Oct;204(2):63P–6P.