Abstract
The E3 ubiquitin ligase is an important regulator of cell signaling and proteostasis and is tightly controlled in many diseases, including cancer. Our study aimed to investigate the biological role of the E3 ubiquitin ligase CBLC in breast cancer and elucidate the specific mechanistic network underlying CBLC-mediated target substrate degradation, cell proliferation and metastasis. Here, we showed that CBLC expression was higher in breast cancer tissues and cells than that in normal tissues and cells. Higher expression of CBLC predicted a better prognosis for breast cancer patients. CBLC inhibited the proliferation, migration and invasion of breast cancer cells. Co-IP and immunofluorescence co-localization assays demonstrated that CBLC interacted with CTTN in the cytoplasm. CBLC promoted the degradation of CTTN through the ubiquitin-proteasome pathway without affecting its mRNA level. The inhibitory effect of CBLC on breast cancer cell proliferation, migration and invasion could partly be reversed by CTTN. Taken together, our study clarified the biological role of CBLC as a tumor suppressor and discovered its functional substrate, providing a molecular basis for CBLC/CTTN as a potential therapeutic target in breast cancer.
Introduction
Metastatic breast cancer is a serious threat to women’s health due to its high recurrence rate and high mortality. The 5-year overall survival rate of metastatic breast cancer remains less than 30% [Citation1]. Moreover, the extent of response and potential resistance to clinical treatments varies among patients with metastatic breast cancer [Citation2]. Discovering novel effective therapeutic targets for metastatic breast cancer is still urgently required.
Casitas B-Lineage Lymphoma Proto-Oncogene (CBL) family proteins are RING finger E3 ubiquitin ligases which are required for target substrates for degradation by the proteasome [Citation3]. The CBL family consists of CBL, CBLB, and CBLC, which share a highly conserved structure in the N-terminus, including a linker region, a tyrosine-kinase binding (TKB) domain, and a RING finger domain, the latter two of which determine the E3 activity [Citation4]. In the C-terminal segments, however, CBLC lacks a proline-rich region, followed by a stretch of amino acid residues with several serine and tyrosine phosphorylation sites and a ubiquitin association domain in comparison with CBL and CBLB.
The expression pattern of CBLC in various tissues is quite different from that of CBL and CBLB. While CBL and CBLB are highly expressed in hematopoietic tissues and the testes [Citation5], CBLC is inclined to be expressed in the gastrointestinal tract, liver, kidney, pancreas, and prostate [Citation6,Citation7]. Presently, there are few reports about the expression level of CBLC in breast cancer. CBLC could interact with receptor tyrosine kinase RET, resulting in the ubiquitin degradation and decreased protein stability of RET in breast cancer. Kaplan-Meier analysis of breast cancer patients with high RET expression showed that patients with high CBLC expression had a higher survival rate, suggesting that higher expression of CBLC was associated with a better prognosis of breast cancer [Citation8,Citation9]. However, the physiological roles of CBLC in breast cancer remain unknown. Here, we evaluated the functions and underlying mechanisms of CBLC in breast cancer. Our results suggested that targeting CBLC or its direct substrate CTTN could serve as a potential strategy for the treatment of metastatic breast cancer.
Materials and methods
Cell lines and cell culture
Breast cancer cell lines (MCF10A, BT474, MDA-MB-231, MCF7, SK-BR-3 and UACC-812) and 293 T cells were obtained from the China Center for Type Culture Collection (CCTCC, China) and cultured in Dulbecco’s modified Eagle’s medium (DMEM) supplemented with 10% (v/v) fetal bovine serum (FBS) and 1% antibiotics (Gibco) in a humidified incubator containing 5% CO2 at 37 °C.
Plasmid and siRNA transfection
The Flag-CBLC, HA-CTTN, EGFP-CBLC, DSRED-CTTN and HA-Ub plasmids were purchased from Focus Biology (Nanchang, China) and purified with a Mini Plasmid Kit (DP103, Tiangen, China). CBLC knockdown in breast cancer cells was achieved by transfection of small interfering RNA (siRNA) targeting CBLC (siCBLC) (GeneBio, China). Plasmid and siRNA transfections were carried out using jetPRIME (114-15, Polyplus- transfection, FR) reagent. The siRNA sequences are listed in Table S1.
Immumohistochemical staining
Tissue microchips (HBre-Duc060CS-03) containing 30 breast cancer tissues and 30 paracancer tissues were purchased from Shanghai OUTDO Biotech Co., LTD. Immunohistochemical staining was performed on these tissue microchips. A Pannoramic MIDI scanner (3 D HISTECH) was used to obtain all staining information on tissue sections. Pannoramic Viewer was used to capturing representative images and DensitoQuant software was applied to determine the staining score. The area of strong positive (dark brown), moderate positive(brown-yellow), weakly positive(light yellow) and negative positive(blue) was analyzed, H-score was calculated according to the following formula. H score=∑(PI × 1)=(weakly positive PI × 1)+(moderately positive PI × 2)+(strongly positive PI × 3), where P represents the percentage of the number of positive cells and I stands for color intensity. The scatter plot shows the H-score of each section.
Real-time fluorescence quantitative PCR (RT-qPCR)
Total RNA was isolated from breast cancer cell lines using TRIzol (Invitrogen, USA) reagent, and cDNA synthesis was performed using the PrimeScriptTM RT reagent Kit (Takara, Japan). RT-qPCR was performed using a SYBR Green PCR system (Takara, Japan). The 2−ΔΔCT method was used to calculate the relative fold change values. The primers are listed in Table S2.
Western blot
Western blotting (WB) was carried out routinely. The primary antibodies used included anti-DYKDDDDK tag (Flag) (98533S, CST, USA), anti-HA (3724S, CST, USA), CBLC (NBP1-02810, Novus, USA), CTTN (3503, CST, USA), GAPDH (AC001, ABclonal, USA) and β-Actin (4970, CST, USA). The second antibodies used included goat anti-mouse (14709, CST, USA) and goat anti-rabbit (707492, CST, USA) protein bands were visualized with a chemiluminescence system.
Cell counting kit-8 (CCK8) assay
Cells (5 × 105) were seeded in 96-well. CCK8 reagent (C0037, Beyotime, China) was added and incubated for 2 h. The optical density (OD) value was measured at 0, 24, 48 and 72 h.
Wound healing assay
Cells (5 × 105) were seeded into 6-well plates until the confluence of cells reached 90%–95%. Then, 200 µl pipette tips were used to scratch straight lines in two wells. Three fields were randomly selected, and images of the scratches were acquired at the same position after 0, 48 and 72 h. Image J software was used to analyze the widths of the scratches.
Transwell migration and invasion assays
Cells (5 × 105) were added to the upper compartments of transwell migration chambers (3422, Corning, USA) or invasion chambers (354480, Corning, USA), and a complete medium containing 20% FBS was added to the lower compartments. After being cultured in an incubator (37 °C, 5% CO2) for 24 h, cells in the lower compartment were observed and counted in six fields under a microscope.
Identification of CBLC interacting proteins
The Flag-CBLC plasmid was transfected into 293 T cells. Cell lysates were applied to the anti-Flag or anti-HA immunoaffinity resin to enable adsorption of the protein complex. After affinity binding, the precipitate was washed with cold PBS buffer. Proteins in the eluate were separated by electrophoresis, transferred to membranes, and analyzed by WB. The whole gel with the isolated proteins was stained using a silver staining kit (P0017S, Beyotime, China) and submitted for liquid chromatography-tandem mass spectrometry (LC-MS/MS) protein identification. The proteins with research value among the interacting proteins were screened, and pathway enrichment information was obtained by using the DAVID database.
Co-immunoprecipitation (co-IP)
The Flag-CBLC and HA-CTTN plasmids were co-transfected into 293 T cells. Total cell extracts were pre-cleared with 30 µl of Protein G agarose beads (37478S, CST, USA) at 4 °C for 45 min. The supernatant was incubated with the anti-Flag or anti-HA primary antibody and rotated at 4 °C overnight, followed by the addition of 30 µl Protein G agarose beads for 2 h. The beads were washed, resuspended and boiled for 10 min. The proteins were separated by SDS-PAGE (10% SDS) and transferred to a PVDF membrane for immunoblot detection. The primary antibodies used included anti-DYKDDDDK tag (Flag) (98533S, CST, USA), anti-HA (3724S, CST, USA), anti-CBLC (NBP1-02810, Novus, USA), anti-GAPDH (AC001, ABclonal, USA). The second antibodies used included goat anti-mouse (14709, CST, USA) and goat anti-rabbit (707492, CST, USA). Protein bands were visualized with a chemiluminescence system.
Immunofluorescence assay
A total of 2 × 104 293 T or MDA-MB-231 cells were plated in laser confocal Petri dishes. When the confluence reached 70%, cells were transfected with EGFP-CBLC and DSRED-CTTN. Cells were fixed in 4% paraformaldehyde buffer, permeabilized with 0.1% Triton X-100 and incubated with DAPI for 30 min. Confocal microscopy (LSM 880, Carl Zeiss, Germany) was used to acquire the co-localization.
Protein stability experiment
The HA-CTTN plasmid was transfected into MDA-MB-231-Vector or MDA-MB-231-CBLC breast cancer cells, and 62.5 µg/mL cycloheximide (CHX) (HY-100461, Med Chem Express, USA) was added to the culture medium. Proteins from each well were extracted separately at 0, 2, 6,12, 20 and 30 h after CHX treatment and analyzed by WB.
Ubiquitination experiment
Cells were transfected with HA-CTTN, HA-Ub or siCBLC for 24 h. The proteasome inhibitor MG-132 (HY-13259, MedChemExpress, USA) was added at a concentration of 50 µM. HA-CTTN was purified from the lysate by IP and the ubiquitination status of CTTN was detected by immunoblotting.
Statistical analysis
SPSS 17.0 was applied for data analysis. All the data were expressed as mean ± standard deviation. The t-test and Pearson Chi-Squared test were used to compare the significant differences between the indicated groups. Statistical significance was assigned at p < 0.05.
Results
CBLC is highly expressed in breast cancer tissues and cells and is associated with prognosis
We obtained the mRNA expression levels of CBL, CBLB and CBLC by mining the breast cancer gene expression profile data in the TCGA database. We found that only CBLC was highly expressed in paired and unpaired breast cancer tissues, while the expression of CBL and CBLB was lower in breast cancer tissues (). Further extensive cancer analysis showed that CBLC was highly expressed in most malignancies such as bladder urothelial carcinoma (BLCA), cervical and endocervical cancers (CESC), esophageal carcinoma (ESCA) and lung squamous cell carcinoma (LUSC) (). Immunohistochemical experiments confirmed that the expression level of CBLC in breast cancer tissues was significantly higher than that in adjacent tissues, which was consistent with the mRNA expression trend of CBLC (). We used RT-qPCR and WB to detect the mRNA and protein expression levels of CBLC in various breast cancer cells and normal breast cells. The mRNA and protein expression levels of CBLC in breast cancer cells were higher than those in normal breast cells (). Interestingly, the expression level of CBLC in MDA-MB-231 cells with high invasion and high metastasis was lower than that in MCF7 cells with less invasiveness (). Furthermore, an obviously positive association of CBLC expression with the histological type of breast cancer patients was observed (p < 0.001, ). Finally, we mined the correlation between CBLC and the prognosis of breast cancer patients from the TCGA database and found that higher expression of CBLC suggested longer survival time and better prognosis for breast cancer patients (). These results suggest that CBLC may be involved in the progression and development of breast cancer.
Figure 1. CBLC expression in breast cancer tissues and cells. (A,B) mRNA expression levels of CBL, CBLB and CBLC in paired (A) and unpaired (B) breast cancer samples from TCGA database. (C) mRNA expression level of CBLC in pan-cancers. (D) IHC images showing the expression and location of CBLC in 15 breast cancer tissues and 15 para-carcinoma tissues. CBLC expressions were converted to H-score. (E,F) mRNA (E) and protein (F) expression levels of CBLC in five breast cancer cells and normal breast epithelial cells. *p < 0.05 (normalized to MCF10A cells). (G) Kaplan-Meier Curve shows the overall survival of breast cancer patients with different CBLC expression levels.
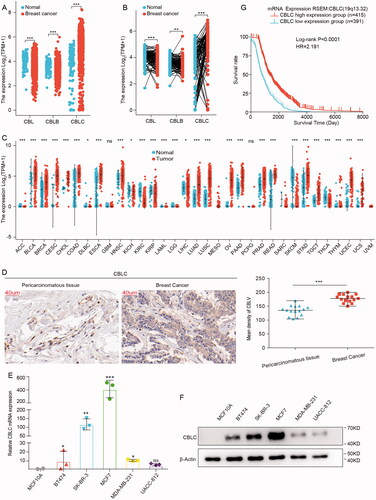
Table 1. The correlation between CBLC expression and the clinical features of breast cancer patients from the TCGA database.
CBLC inhibits the proliferation, migration and invasion of breast cancer cell
We examined the effect of CBLC on the proliferation, migration and invasion of breast cancer cells. As the endogenous CBLC expression was lower in MDA-MB-231 and higher in MCF7 (), we selected MDA-MB-231 cells for the construction of CBLC overexpressing cells by the infection with lentiviruses carrying CBLC (), and MCF-7 cells were transfected with siCBLC for CBLC silencing (). The CCK-8 assay showed that CBLC overexpression significantly inhibited cell proliferation (p < 0.01, ). The wound healing assay indicated that the healed area in the CBLC overexpression group was significantly larger than that in the control group (p < 0.01, ). Transwell assays demonstrated that overexpression of CBLC significantly suppressed cell migration and invasion (p < 0.01, ). In addition, CBLC knockdown in MCF7 cells showed the opposite effects on cell proliferation, migration and invasion compared with CBLC overexpression (). Taken together, these results show that CBLC functions as a tumor suppressor in breast cancer cells.
Figure 2. The effects of CBLC in breast cancer cell migration, proliferation and invasion. (A,B) mRNA and protein expression of CBLC in CBLC overexpressing cells and control cells. (C,D) Knockdown efficiencies of three siRNAs targeting CBLC at mRNA and protein levels. **p < 0.01 (compared with siNC). (E) Effect of CBLC overexpression on MDA-MB-231 cell proliferation. **p < 0.01 (compared with control cells on the same day). (F) Growth kinetics of MCF7 cells transfected with siNC or siCBLC3. *p < 0.05 (compared with siNC group on the same day). (G)Migration abilities of CBLC overexpressing cells and control cells (50×). **p < 0.01. (H) Migration and invasion abilities of MCF7cells transfected with siNC or siCBLC3 (100×). **p < 0.01. (I) Migration and invasion abilities of MDA-MB-231-vector cells and MDA-MB-231-CBLC cells (100×). **p < 0.01. (J) Migration and invasion abilities of MCF7 cells transfected with siNC or siCBLC3 (100×). T. **p < 0.01.
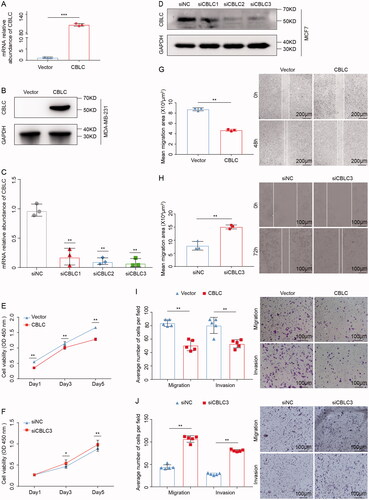
CBLC interacts with CTTN
We identified the direct substrate of CBLC by IP combined with LC-MS/MS. Silver staining showed that a series of proteins were specifically pulled down by the anti-CBLC antibody compared with control IgG (). After eliminating the interference proteins in the control group, a total of 935 proteins were identified as potential CBLC-specific interacting proteins. GO analysis indicated that these proteins were enriched in the biological processes, such as cell redox homeostasis, the cellular component of extracellular exosomes and the molecular function of poly (A) RNA binding (). KEGG pathway analysis revealed an enrichment of CBLC interacting proteins in the biosynthesis of antibiotics、protein processing in the endoplasmic reticulum and Glycolysis/Gluconeogenesis (). Among all the CBLC interacting proteins, CTTN contained seven unique peptides by LC-MS/MS identification, and it has been reported that CTTN could promote the formation of invasive pseudopodia, which is essential for the invasion and metastasis of tumor cells [Citation10–12]. Therefore, we choose CTTN for subsequent verification. Both forward (CBLC pull down CTTN) and reverse (CTTN pull down CBLC) co-IP confirmed the interaction between CBLC and CTTN (). Fluorescence co-localization experiments showed that CBLC was primarily distributed in the cytoplasm, especially in the perinuclear cytoplasm, and that CTTN co-localized with CBLC in the cytoplasmic region in 293 T and MDA-MB-231 cells (). Overall, these results demonstrated that CTTN interacted with CBLC and might be important for the CBLC-mediated malignant phenotypes of breast cancer cells.
Figure 3. Identification of the interaction between CBLC and CTTN. (A) Silver staining of the protein complex pulled down by Flag-CBLC in 293T cells. (B) Biological process/Cellular component/Molecular function enrichment analysis of CBLC interacting proteins. (C) KEGG pathway enrichment analysis of CBLC interacting proteins. (D,E) Forward (D) and reverse (E) co-IP confirmed the interaction between CBLC and CTTN. (F) CBLC with a green fluorescent protein tag and CTTN with a red fluorescent protein tag were colocalized, as visualized by laser confocal microscopy. The top images show the colocalization of CBLC and CTTN in 293T cells, and the bottom images show the colocalization of CBLC and CTTN in MDA-MB-231 cells (1000×).
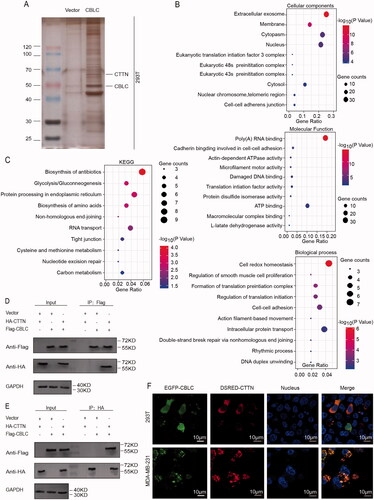
CBLC promotes the ubiquitin-mediated degradation of CTTN
To explore whether CBLC could influence the stability, ubiquitination and degradation of CTTN through the UPS, we first investigated the effect of CBLC overexpression on the mRNA and protein levels of CTTN. RT-qPCR assay indicated that overexpression/knockdown of CBLC had no significant effect on the CTTN mRNA level (). WB analysis showed that overexpression of CBLC significantly reduced the expression level of CTTN, while knockdown of CBLC has the opposite effect (). In the protein stability assay, the expression of CTTN decreased gradually from 0 to 30 h after CHX treatment. CTTN degradation was more obvious in the CBLC overexpression group than in the control group at each time point (). Furthermore, overexpression of CBLC significantly enhanced CTTN ubiquitination in MCF-7 cells, whereas knockdown of CBLC inhibited the ubiquitin modification of CTTN (). These results suggest that CBLC promotes the degradation of CTTN through the UPS, without affecting the transcription of CTTN.
Figure 4. Effect of CBLC on CTTN expression, stability and ubiquitination. (A,B) Effect of CBLC overexpression/knockdown on endogenous CTTN mRNA expression in MDA-MB-231/MCF7 cells. (C,D) Effect of CBLC overexpression/knockdown on CTTN protein expression in MDA-MB-231/MCF7 cells. (E) The stability of CTTN after treatment with cycloheximide (CHX) at different time points in CBLC overexpressing cells and control cells. (F) WB detection of CTTN expression and ubiquitination in MCF7 cells transfected with Flag-CBLC or vector pretreated with MG132. Input: protein expression in the extracted supernatant, Eluate: protein expression after purification by IP. (G) WB detection of CTTN expression and ubiquitination in MDA-MB-231 cells transfected with siNC or siCBLC pretreated with MG132. Input: protein expression in the extracted supernatant, Eluate: protein expression after purification by IP.
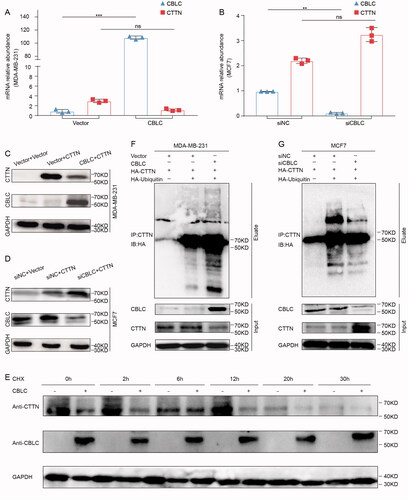
CTTN is the functional target of CBLC
To confirm the biological roles of CTTN in CBLC-mediated proliferation, invasion and migration of breast cancer cells, the MCF-7 cells were transfected with control siRNA or siCTTN for CTTN silencing (). CTTN was also transiently expressed in MDA-MB-231 cells stably expressing CBLC. The CCK-8 results showed that overexpression of CTTN partially reversed the tumor growth inhibition mediated by CBLC (p < 0.01, ). Wound healing assays and transwell assays confirmed that the CBLC-mediated inhibitory effects on cell migration and invasion were partially rescued by CTTN (p < 0.01, ). In addition, MDA-MB-231 cells were transfected with control siRNA, siCBLC or siCBLC combined with siCTTN, respectively. Knockdown of CTTN in MDA-MB-231 cells significantly reversed the effects of CBLC silencing on cell proliferation, migration and invasion (p < 0.01). Taken together, these results suggested that CBLC regulates the proliferation and metastasis of breast cancer cells partly through CTTN degradation.
Figure 5. CTTN is the functional target of CBLC. (A) Knockdown efficiencies of three siRNAs targeting CTTN at and protein levels by WB. **p < 0.01 (compared with siNC). (B,E) CCK8 assay of MDA-MB-231-CBLC cells. (B) transfected with the pcDNA3.1/HA-CTTN plasmid, and that of. MCF7-siCBLC cells. (E) transfected with siNC/siCTTN2. (C,F) Wound healing assay of MDA-MB-231-CBLC (C) cells transfected with the pcDNA3.1/HA-CTTN plasmid, (F) and that of MCF7-siCBLC cells transfected with siNC/siCTTN2 100×). (D,G) The transwell assay of MDA-MB-231-CBLC cells (D) transfected with the pcDNA3.1/HA-CTTN plasmid and that of MCF7-siCBLC cells, (G) transfected with siNC/siCTTN2 (100×).
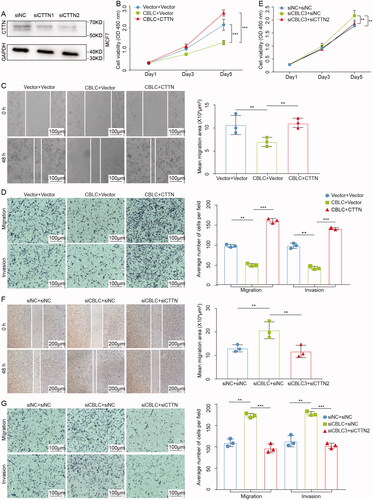
Discussion
The results of this study showed that the expression of CBLC was higher in breast cancer tissues than that in tumor-adjacent tissues (). CBLC overexpression inhibited the proliferation, migration and invasion of breast cancer cells (). Breast cancer patients with high expression of CBLC have a better prognosis, which is consistent with the in vitro biological effects of CBLC (). The seemingly contradictory phenomenon between the expression level of this gene and its biological role can be explained from available evidence, such as p16Ink4a and p63. Currently, p16Ink4a is considered a tumor suppressor protein. However, p16Ink4a can also be overexpressed in tumors, and few studies have been performed to elucidate this fact [Citation10]. p63 was another tumor suppressor that could function in a similar capacity to p53. Interestingly, p63 is often overexpressed and amplified in cancer, thus revealing a paradox [Citation11]. We speculated that although the high expression of CBLC inhibits the occurrence and development of breast cancer, the impact of oncogenic transcriptional factors that control the expression of CBLC might cover the biological role of CBLC itself.
Previous studies have shown that CBL family proteins have a certain expression tendency in different tumors. The expression of CBL and CBLB were up-regulated in glioma and colorectal cancer and were closely associated with tumor progression and metastasis [Citation12,Citation13]. CBL and CBLB could also alleviate immunosuppression and inhibit tumor metastasis in breast cancer and non-small cell lung cancer [Citation14–17]. The differences in the roles of CBL family proteins in different tumors may be explained by the different substrates they target and the different downstream signaling pathways they activate. CBLC could interact with RET, resulting in the ubiquitination and degradation of RET and inhibition of RET-mediated ERK activation [Citation8]. The RF and TKB domains in the N-terminus of CBLC determined its E3 ligase activity. Moreover, the phosphorylation of CBLC by recruiting Src on Tyr341 is important for CBLC activation and its E3 ligase functions [Citation18]. Specifically, the SH3 domain of Src mediates its interaction with Tyr341 from CBLC. Therefore, CBLC-related molecular mechanisms involve not only self-activation but also competition or coordination with interacting proteins.
The oncogene CTTN functions in regulating the interaction between cell adhesion components and the arrangement of the cytoskeleton [Citation19]. Aberrant expression of CTTN often leads to tumor cell invasion and metastasis [Citation20] and CTTN can also trigger anoikis resistance [Citation21–23]. The N-terminus of CTTN interacts with ARP2/3 and activate ARP2/3-mediated self-polymerization and pseudopodia formation, resulting in cancer cell metastasis [Citation24]. Knockdown of CTTN significantly reduces pseudopodia formation in non-small cell lung cancer [Citation25,Citation26]. As the expression of CBLC is decreased in MDA-MB-231 cells with high metastatic potential (), it is insufficient for CBLC to degrade oncogenes such as CTTN, leading to the accumulation of CBLC and pseudopodia formation.
CBL can be recruited by the EGFR and promote the endocytosis and degradation of EGFR [Citation27,Citation28]. CTTN overexpression inhibits CBL activation and blocks the interaction between CBL and EGFR [Citation29–31], thus impairing the ubiquitination of EGFR and contributing to EGFR-induced malignant phenotypes. We supposed that CTTN degradation mediated by CBLC might eliminate the restriction of CTTN on CBL activation, leading to the degradation of EGFR by CBL. However, this hypothesis still needs further verification.
PROTAC technology can efficiently and specifically degrade target proteins. Dozens of PROTAC substrate proteins have been clinically verified as drug targets [Citation32]. CTTN, which is highly expressed in breast cancer, is also one of the ideal targets for PROTAC. In the future study, we will construct PROTAC molecules targeting CTTN to facilitate CTTN degradation, which could offer more options for breast cancer treatment.
Above all, our study has reported CBLC as a novel tumor suppressor in breast cancer. CBLC could bind to and deliver CTTN for degradation by USP, inhibiting CTTN-mediated cell proliferation and metastasis. CBLC/CTTN signaling axis might be a potential therapeutic strategy for breast cancer.
Supplemental Material
Download MS Word (14.1 KB)Disclosure statement
The authors report no competing interests.
Data availability statement
The raw data supporting the conclusions of this article will be made available by the authors, without undue reservation.
Additional information
Funding
References
- Jin L, Han B, Siegel E, et al. Breast cancer lung metastasis: molecular biology and therapeutic implications. Cancer Biol Ther. 2018;19(10):858–868.
- Liang Y, Zhang H, Song X, et al. Metastatic heterogeneity of breast cancer: molecular mechanism and potential therapeutic targets. Semin Cancer Biol. 2020;60:14–27.
- Weissman AM, Shabek N, Ciechanover A. The predator becomes the prey: regulating the ubiquitin system by ubiquitylation and degradation. Nat Rev Mol Cell Biol. 2011;12(9):605–620.
- Li X, Gong L, Gu H. Regulation of immune system development and function by CBL-mediated ubiquitination. Immunol Rev. 2019;291(1):123–133.
- Keane MM, et al. Cloning and characterization of CBL-B: a SH3 binding protein with homology to the C-CBL proto-oncogene. Oncogene. 1995;10(12):2367–2377.
- Kim M, Tezuka T, Suziki Y, et al. Molecular cloning and characterization of a novel CBL-family gene, CBL-C. Gene. 1999;239(1):145–154.
- Kiguchi K, Bol D, Carbajal S, et al. Constitutive expression of erbB2 in epidermis of transgenic mice results in epidermal hyperproliferation and spontaneous skin tumor development. Oncogene. 2000;19(37):4243–4254.
- Kales SC, Nau MM, Merchant AS, et al. Enigma prevents CBL-C-mediated ubiquitination and degradation of RETMEN2A. PLOS One. 2014;9(1):e87116.
- Provenzano E, Ulaner GA, Chin SF. Molecular classification of breast cancer. PET Clin. 2018;13(3):325–338.
- Romagosa C, Simonetti S, López-Vicente L, et al. p16(Ink4a) overexpression in cancer: a tumor suppressor gene associated with senescence and high-grade tumors. Oncogene. 2011;30(18):2087–2097.
- Finlan LE, Hupp TR. p63: the phantom of the tumor suppressor. Cell Cycle. 2007;6(9):1062–1071.
- Luo P, Wang X, Zhou J, et al. C-CBL and CBL-B expression in skull base chordomas is associated with tumor progression and poor prognosis. Hum Pathol. 2018;74:129–134.
- Lyle C, Richards S, Yasuda K, et al. c-CBL targets PD-1 in immune cells for proteasomal degradation and modulates colorectal tumor growth. Sci Rep. 2019;9(1):20257.
- JING Z, LI LONG, WANG XIN, et al. High C-CBL expression in gliomas is associated with tumor progression and poor prognosis. Oncol Lett. 2016;11(4):2787–2791.
- Wang S, Xu L, Che X, et al. E3 ubiquitin ligases CBL-B and C-CBL downregulate PD-L1 in EGFR wild-type non-small cell lung cancer. FEBS Lett. 2018;592(4):621–630.
- Xu L, Zhang Y, Qu X, et al. E3 ubiquitin ligase CBL-B prevents tumor metastasis by maintaining the epithelial phenotype in multiple drug-resistant gastric and breast cancer cells. Neoplasia. 2017;19(4):374–382.
- Cascio S, Finn OJ. Complex of MUC1, CIN85 and CBL in colon cancer progression and metastasis. Cancers. 2015;7(1):342–352.
- Ryan PE, Sivadasan-Nair N, Nau MM, et al. The N terminus of CBL-C regulates ubiquitin ligase activity by modulating affinity for the ubiquitin-conjugating enzyme. J Biol Chem. 2010;285(31):23687–23698.
- Wei C-Y, Zhu M-X, Yang Y-W, et al. Downregulation of RNF128 activates WNT/β-catenin signaling to induce cellular EMT and stemness via CD44 and CTTN ubiquitination in melanoma. J Hematol Oncol. 2019;12(1):21.
- Zhao Y, Lei Y, He S-W, et al. Hypermethylation of UCHL1 promotes metastasis of nasopharyngeal carcinoma by suppressing degradation of cortactin (CTTN). Cells. 2020;9(3):559.
- Luo M-L, Shen X-M, Zhang Y, et al. Amplification and overexpression of CTTN (EMS1) contribute to the metastasis of esophageal squamous cell carcinoma by promoting cell migration and anoikis resistance. Cancer Res. 2006;66(24):11690–11699.
- Castagnino A, Castro-Castro A, Irondelle M, et al. Coronin 1C promotes triple-negative breast cancer invasiveness through regulation of MT1-MMP traffic and invadopodia function. Oncogene. 2018;37(50):6425–6441.
- Lang L, Hou Y, Chen Y, et al. ATM-Mediated phosphorylation of cortactin involved in actin polymerization promotes breast cancer cells migration and invasion. Cell Physiol Biochem. 2018;51(6):2972–2988.
- Meng D-F, Xie P, Peng L-X, et al. CDC42-interacting protein 4 promotes metastasis of nasopharyngeal carcinoma by mediating invadopodia formation and activating EGFR signaling. J Exp Clin Cancer Res. 2017;36(1):21.
- Gang Z, et al. Combining cortactin and CTTN detection with clinicopathologic features increases effectiveness of survival predictions for patients with resectable hepatocellular carcinoma. Clin Lab. 2013;59(11–12):1343–1352.
- Li Y, Zhang H, Gong H, et al. miR-182 suppresses invadopodia formation and metastasis in non-small cell lung cancer by targeting cortactin gene. J Exp Clin Cancer Res. 2018;37(1):141.
- Brand K, Kentsch H, Glashoff C, et al. RASopathy-associated CBL germline mutations cause aberrant ubiquitylation and trafficking of EGFR. Hum Mutat. 2014;35(11):1372–1381.
- Shrestha N, Shrestha H, Ryu T, et al. δ-Catenin increases the stability of EGFR by decreasing C-CBL interaction and enhances EGFR/Erk1/2 signaling in prostate cancer. Mol Cells. 2018;41(4):320–330.
- Zhang X, Liu K, Zhang T, et al. Cortactin promotes colorectal cancer cell proliferation by activating the EGFR-MAPK pathway. Oncotarget. 2017;8(1):1541–1554.
- Timpson P, Lynch DK, Schramek D, et al. Cortactin overexpression inhibits ligand-induced down-regulation of the epidermal growth factor receptor. Cancer Res. 2005;65(8):3273–3280.
- Hashimoto A, Hashimoto S, Ando R, et al. GEP100-Arf6-AMAP1-cortactin pathway frequently used in cancer invasion is activated by VEGFR2 to promote angiogenesis. PLOS One. 2011;6(8):e23359.
- Li X, Song Y. Proteolysis-targeting chimera (PROTAC) for targeted protein degradation and cancer therapy. J Hematol Oncol. 2020;13(1):50.