Abstract
Purpose. The purpose of this article was to determine how characteristics of bimanual coordination tasks affect the quality of performance and to determine the impact of these characteristics on muscular activation of the upper limbs, with consideration of age-related differences. Methods. The research was carried out on two groups consisting of 25 people aged 20–30 and 60–67 years. The subjects performed seven tasks that varied in coordination mode, tracking mode and outline-tracing. The main measures of task performance were calculated on the basis of the difference between the position of the target and tracing cursors. Cohen’s d value was calculated to show differences in measures between groups. Results. There were higher values of error and variability measures for elderly people compared to young. Complex tasks showed the largest difficulty, which suggests that, when performed, such tasks have the greatest potential to improve coordination skills. Tasks during which both limbs contribute to the movement of one cursor proved the most appropriate. Conclusion. The tracking mode is of great importance for the quality of performance in motor coordination tasks, while the performance of tasks with imposed speed is much more strongly age-sensitive than performance with a freely chosen speed.
1 Introduction
The ageing of humans is associated with progressive structural and functional changes. The changes concern inter alia the morphology of human skeletal muscles. The deterioration of muscle function is due to changes in muscle mass, and fibre composition and size [Citation1]. Changes in the nervous and musculoskeletal systems resulting in deterioration of muscle function affect the maximum strength capacity [Citation2]. Slower peripheral nerve conduction and age-related changes in the structure and function of skeletal muscles may be the causes of motor activity disorders, as well as cognitive impairments, such as processing speed and executive function [Citation3]. This results in reduced motor coordination [Citation4], increased inaccuracy of motion and slower motion [Citation5]. By extension, the elderly can be less accurate when performing various motor tasks, as the response time, speed of movement and motor coordination are all reduced with age [Citation6,Citation7].
The tasks of bimanual coordination constitute a particular challenge [Citation8]. In existing studies on bimanual coordination, numerous motor tasks are used. Isometric force tasks with visual feedback are among them [Citation9,Citation10]. Studies demonstrate variation in the effectiveness of bimanual coordination tasks depending on the type of task performed [Citation11–13]. There are different classifying features describing bimanual coordination tasks. These relate to the symmetry of the task (symmetric and asymmetric). Symmetric coordination patterns require each limb to perform the same activity. Simultaneous change over time resulting from spatial movement or the force exerted occurs in such a manner that one limb moves in a mirror reflection of the other, which is related to the simultaneous contraction of the same muscle groups in both limbs. Tasks with full symmetry are called in-phase tasks. Coordination in anti-phase involves alternating contractions of the same muscle groups. In the case of multi-phase tasks, one limb follows the other limb with a time lag [Citation14,Citation15]. Bimanual coordination tasks can also be varied according to the nature of the movement or exertion of force, indicating discrete and continuous tasks. Continuous bimanual coordination tasks are connected to continuous movement and/or exertion of force, without a break between repetitions [Citation13,Citation16]. Discrete bimanual coordination tasks include single or consecutive movements with a clear interruption between each movement [Citation13].
The performance of tasks requiring the maintenance of a force of varying character, at a specified level, requires muscle activity of the upper limbs. Mechanical strain and job demands are important for the musculoskeletal load [Citation17]. The involvement of upper limb muscles can be determined with the use of surface electromyography (EMG) [Citation18,Citation19]. Due to muscle redundancy, one basic problem for task performance is to understand how muscles are coordinated to perform common tasks adequately [Citation20]. Different individuals may have different levels of sensitivity or reactivity to load, which may be affected by their psychological disposition and pathology [Citation21]. Forearm muscles are activated differently during functional tasks in people with computer-related wrist/hand symptoms [Citation22]. The situation is similar for elderly workers. Another aspect of muscle activity is the psychological dimension. In addition to physical load, psychosocial stressors may contribute to the increase in muscle activity during exposure to a performed task [Citation23,Citation24]. Shahidi et al. [Citation25] showed that activity significantly increased from conditions of low to high stress for both the dominant and non-dominant upper trapezius, which suggests that the upper trapezius muscles are selectively activated by psychosocial stress. It may then be expected that the activation pattern of upper limb muscles during the performance of coordination tasks differs between young and elderly people, also due to psychosocial factors related to task difficulty.
The differences in coordination capacity between young and elderly people have been the subject of previous studies. Krehbiel et al. [Citation26] present a summary of these studies and offer a meta-analysis of the error indicators for the performance of bimanual coordination tasks with respect to variability, accuracy and time. Their analysis demonstrated a significant reduction in accuracy and variability, and an increase in movement time among the elderly people as compared to the young. The analysis, however, did not take into account the impact of the characteristics of the performed bimanual task or the value of the performance measures for coordination tasks.
The purpose of the study presented in this article was to examine whether the characteristics of bimanual coordination tasks have an impact on performance and to what extent this performance is age-related.
2 Methodology
2.1 Study subjects
The research was carried out on two groups consisting of 26 people aged 20–30 and 60–67 years. In both study groups, people who were right-handed and had not experienced any neurological, cardiac or musculoskeletal disorders in the last 5 years were selected. The characteristics of the study subjects are presented in Table . The research procedure has been approved by the Ethics Committee. Prior to the commencement of the study, the subjects were informed of the purpose and course of the study, and they all signed consent forms.
Table 1. Characteristics of the study subjects.
2.2 Test stand
The task of the participants was to move cursors on a screen. The movement of the tracking cursor on the monitor screen was triggered by exerting a force on a fixed-control joystick using the upper limbs. The position of the end of the resultant moment of force of the steering joysticks was presented ‘online’ on the monitor screen as a tracking cursor. The outline of the shape was visible on the screen, and differed depending on the task. The objective was to move the tracking cursor as close as possible to the line of the shape visible on the screen. In some tasks, there was also a second cursor that fixed the speed of the tracking cursor’s movement (target cursor). In these tasks, the subject also had to move the tracking cursor as close as possible to the target cursor.
In addition to the screen, the test stand consisted of a base frame mounted on the table surface and fixed to the top. It consisted of three profiles with guides; two two-axle sets of torque meters with a steering grip (joystick), mounted in a sliding manner onto the arms of the frame of the base; two adjustable, upholstered supports stabilizing the forearm of the study subject; and a four-channel tensometric amplifier designed to communicate with a computer. The view of the test stand is shown in Figure .
2.3 Procedure
The examination started with an interview with the study participant, where the purpose of the study was presented and the tasks were described. Following the signing of the relevant documents, the study subjects familiarized themselves with the test stand and the tasks they would perform during the test. The EMG electrodes were affixed and the reference measurements for the purpose of normalization of the EMG signal were carried out. The initial measurement of the maximum moments of force took place separately for each hand in four directions (front, rear, left, right). The initial measurement was used to determine the control force ranges for the performance of subsequent tasks, which amounted to 10% of the measured maximum moments of force.
2.4 Bimanual coordination tasks
All tasks performed were bimanual visual and motor coordination tasks and consisted of control by force of cursors moving on defined tracks by means of two fixed joysticks (one for each hand). The position of the cursor on the screen was proportional to the bending moment exerted by the study subject on the joystick in a given direction.
All bimanual coordination tasks were continuous and differences concerned only the coordination mode, the complexity of the shapes displayed on the screen and the cursor tracking mode (imposed speed) or shape tracking mode (freely chosen speed). Therefore, the performed tasks were varied due to the symmetry and complexity of the task, and the tracking mode. Features of the tasks in relation to the coordination mode, tracking mode and outlined shape are presented in Table .
Table 2. Features of bimanual coordination tasks with regard to coordination and shape tracking mode, and the shapes displayed on the screen.
During the task with imposed speed in anti-phase (IAP), the cursor moved on an ellipse at an imposed speed. There were two ellipses visible on the screen and two cursors on the left and right sides of the screen. The task of the study subject was to follow the target cursor moving at a constant speed, steering the left cursor using the left joystick and the right cursor using the right joystick. The measurement ended when the cursors completed a full lap around the ellipse. The cursors moved in the same direction, performing the task in an anti-phase.
The task with imposed speed, complex with two cursors (ICT) consisted of steering two cursors (one for each limb) on rectangular tracks with an imposed speed in the opposite direction for both hands. There were two rectangles visible on the screen, one for each limb. The task of the study subject was to follow the target cursors moving at a constant speed. The measurement ended when the cursors completed a full lap around the rectangles. The cursors moved in the opposite direction, and the speed in the horizontal direction was twice the speed in the vertical direction. This means that a complex task was performed.
The task with freely chosen speed in-phase (FP) was a symmetric task in-phase, which traced the shape of an ellipse. Controlling the cursors took place on ellipsoid tracks for 60 s at a freely chosen speed in the opposite direction for both hands. The measurement started from moving both cursors to arrows located at the bottom of the ellipse. These arrows indicated the direction in which the cursors had to be moved on the ellipsoid track. The speed of movement on the tracks was arbitrary, but it was a requirement that the movement was symmetric.
In the task with imposed speed in-phase (IP), the cursors were steered on tracks resembling a crown. The speed of movement was imposed by moving at the constant speed of the target cursor. The task of the study subject was to follow the target cursor with a tracking cursor controlled by a joystick. The direction of movement of the two cursors on the track was opposite (movement in-phase), resulting in symmetric movement in relation to the centre of the screen. The force exerted on both joysticks was symmetric in relation to each other. The task was carried out in two steps. In the first step, the movement took place first on an external outdoor circuit and then on an internal circuit. In the second step, the cursor began its movement from the inner shape.
In the first task with imposed speed, complex with one cursor (ICOa), a single cursor was moved on a track in the shape of a cloud. The cursor moved on the trajectories designated by a shape displayed permanently on the screen, chasing the target cursor. Cursor control was carried out by means of both joysticks, while the horizontal movement (left–right) was controlled by the left joystick and the vertical movement (up–down) by the right joystick. The speed of movement was imposed by the constant speed of the target cursor.
In the second task with imposed speed, complex with one cursor (ICOb), a single cursor was moved on a track in the shape of a four-leaf clover. Cursor control was carried out by means of both joysticks, while the horizontal movement (left–right) was controlled by the left joystick and the vertical movement (up–down) by the right joystick. The speed of movement was imposed by the fixed speed of the target cursor.
The task with freely chosen speed, complex with two cursors (FCT) consisted of steering two cursors on lines in a sinusoidal shape at a freely chosen speed for a maximum of 60 s. A cursor was moved on the large sinusoid with the right hand by means of a joystick, and with the left hand on the small sinusoid. The measurement began when both cursors were placed on the arrows at the beginning of the line. The speed of movement on the lines was arbitrary. Participants were told to bring the left and right cursors as close as possible to each other. The distance between these two cursors, however, was not controlled.
The set of bimanual coordination tasks described was carried out three times, for about 45 min. In the tasks in which the speed of movement of the cursor was imposed, the speed increased by 30% in the second performance compared to the first, and by 50% in the third compared to the first. The speed in the first performance was 6°/s.
2.5 EMG signal measurement
Surface EMG was used to assess the load of the upper limbs during each of the tasks performed. Eight muscles were tested: four muscles of the upper right limb and four muscles of the upper left limb. These muscles were: flexor carpi ulnaris (FC); biceps brachii caput breve (BB); deltoideus pars acromialis (DE); trapezius pars descendens (TR). These are all forearm and shoulder muscles. Forearm muscles were expected to respond to handgrip and the forces necessary to move the cursor. Shoulder muscles are related to mental load, which means that those muscles can express difficulty in tasks.
The EMG signal measurements were carried out using surface double-differential sensors DE-3.1 (Delsys Inc., USA), containing three active electrodes. The EMG signal was recorded with the Bagnoli-16 apparatus (Delsys Inc., USA). The control of the measuring process was carried out using a computer. The EMG signal was recorded using EMG Works version 4.0. The EMG signal sampling frequency was 2 kHz. The transfer band of the Bagnoli-16 apparatus is between 20 and 450 Hz. The signal was amplified with a strengthening factor of 1000.
Prior to the placement of the sensors on the skin over the examined muscle, both the skin and the sensors were cleaned and disinfected with alcohol. The skin was properly prepared (including shaving, if necessary, slight abrasion and cleaning with an alcohol solution) to obtain an inter-electrode resistance below 2 kΩ. Electrodes were placed on the skin over the muscle belly, longitudinally to muscle fibres. The placement of measurement sensors on the skin was carried out in accordance with the guidelines given in the SENIAM (Surface ElectroMyoGraphy for the Non-Invasive Assessment of Muscles) programme [Citation27].
2.6 Performance measures of the coordination tasks and the load indicators of the upper limbs
The performance measures of the coordination task are variables calculated on the basis of the difference between the position of the reference point and the position of the point corresponding to the control by means of a joystick (tracing cursor). The effect of performance was studied with respect to measures that express the ability to precisely maintain spatial and temporal demands. Two spatial variables were under analysis: mean error and variability. The mean error is the integral of values as the difference between the course of the target curve and the course of the mapping curve, related to the duration of the analysed task. Variability is the value of the standard deviation of the differences between the target curve and the mapping curve. Those two measures were independent of time. Meanwhile, the execution time is the duration of the performance of the task. As such, the mean error and variability are spatial variables, and the execution time is a temporal variable.
The normalized root mean square (rms) value of a signal recorded from each muscle is assumed as the upper limb load measure, thus generating four load indicators. The rms parameter is set out from a 2000-sample window, which corresponds to a 1-s fragment of the signal. This value is the normalized rmsmax value calculated for the test with the maximum activation of the muscle, carried out prior to the proper test, and averaged over all values for the given task.
2.7 Analysis of the study results
The main objective of the presented study was to analyse the differences between older and younger people in particular tasks, in relation to task performance measures and load measures of the upper limbs. The varied quantitative measures of coordination and load indicators of the upper limbs were carried out using Cohen’s d indicator, which is an appropriate effect size for the comparison between two means [Citation28]. Cohen’s d value was calculated as the difference between the means for the elderly and the young, divided by the pooled standard deviations. The calculated indicator gives quantitative information on the differentiation among individual tasks and among groups. For Cohen’s d values lower than 0.2 the effect size is small, between 0.2 and 0.5 the effect size is medium, above 0.8 the effect size is large and above 1.3 the effect size is very large [Citation28].
The data lack a normal distribution and, as such, non-parametric tests were applied. The differences between the performance measures of coordination tasks and the upper limb load indicator for the older and younger groups were tested using the Mann–Whitney U test. The analysis of differences in the performance measures and the upper limb indicators obtained in the analysed tasks was carried out using the analysis of variance (ANOVA) Friedman test and χ2 statistics.
Because there were no statistically significant differences between left and right upper limbs, data for performance and EMG recordings obtained for the left and right sides were pooled. This avoided bias due to lateralization. As the non-dominant hand could increase the error score, pooling the data from both dominant and non-dominant hands balanced the distribution.
An analysis that indicated the existence of differences between the three parts of the task was also presented. In this analysis, two groups were distinguished based on the tracking mode: tasks with imposed movement speed and tasks with freely chosen speed.
Statistica version 10.0 was used for the statistical analyses.
3 Results
The data distribution of performance measures is presented in Figure . The figure shows differences in the data distribution between young and elderly participants. For the young group, values are in the range up to 0.3 with single outliers. The elderly group measured values are up to 0.5, also with few outliers. In the case of the mean error for the elderly group there seems to be more outliers than for variability and for both measures for the young group. However, even in this case, the number of outliers seems not to affect the results.
Figure 2. Data distribution of the performance measures: (a) mean error in the young group; (b) mean error in the elderly group; (c) variability in the young group; (d) variability in the elderly group.
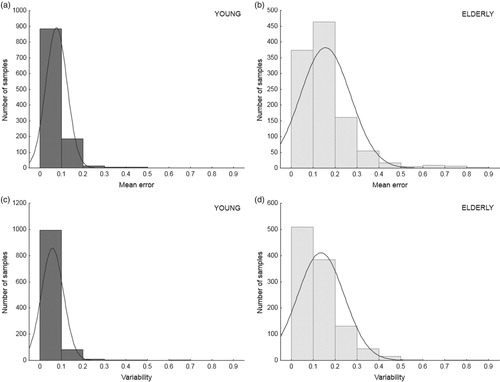
The performance measures of coordination for each task, taking into account data pooled over three sets of tasks performed (under different speeds) for the elderly and younger people, are shown in Figure . The results indicate a significant difference between the values of the performance measures obtained for the elderly group and those obtained for the group of young people. Attention should also be paid to the lack of proportionality, namely that for some tasks the differences between age groups are greater than for others.
Figure 3. Mean and 95% confidence interval of (a) mean error, (b) variability and (c) execution time in seven tasks differentiated by coordination mode, tracking mode and outlined shape. Note: FCT = freely chosen speed, complex with two cursors; FP = freely chosen speed in-phase; IAP = imposed speed in anti-phase; ICOa = first imposed speed, complex with one cursor; ICOb = second imposed speed, complex with one cursor; ICT = imposed speed, complex with two cursors; IP = imposed speed in-phase.
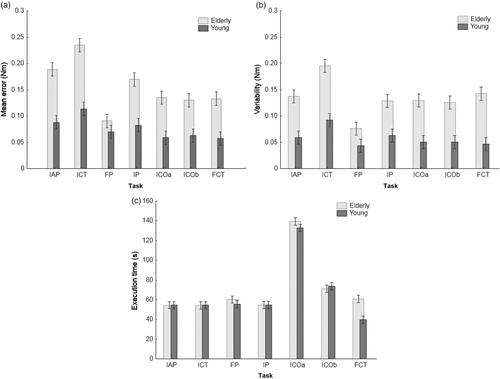
The differences between the values of the performance measures of coordination between the younger and elderly groups were examined using the Mann–Whitney U test. The results of the analysis indicate, in principle, the variation of all measures in all parts of the task with p < 0.001. This is similar for the FC, BI and DE muscle load indicators. An exception is the TR muscle, for which there are no statistically significant differences between the groups of elderly and younger people.
Figure shows the load indicator values broken down by age group and the task of bimanual coordination for the four analysed muscles. The highest level was obtained for the TR muscle for both age groups. High values of muscle load were also observed for the DE muscle but only for the young group. In the BB muscle, on the contrary, the level of muscle load was very low, close to 10%.
Figure 4. Mean and 95% confidence interval of normalized root mean square upper limb load indicators for (a) flexor carpi ulnaris, (b) biceps brachii caput breve, (c) deltoideus pars acromialis and (d) trapezius pars descendens in seven tasks differentiated by coordination mode, tracking mode and outlined shape. Note: FCT = freely chosen speed, complex with two cursors; FP = freely chosen speed in-phase; IAP = imposed speed in anti-phase; ICOa = first imposed speed, complex with one cursor; ICOb = second imposed speed, complex with one cursor; ICT = imposed speed, complex with two cursors; IP = imposed speed in-phase.
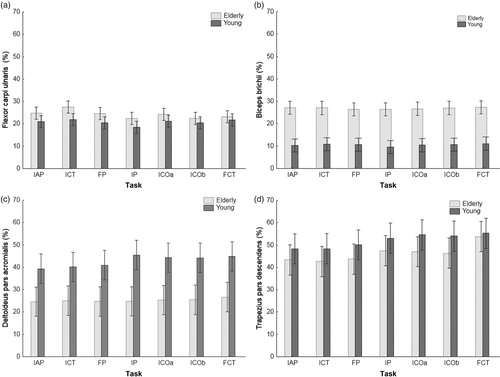
Table presents the effect size with regard to the differentiation of the performance measures and the upper limb load indicators between the examined groups. The lowest values of Cohen’s d indicator were obtained for the FP task, i.e., the task of tracking a shape in-phase at a freely chosen speed. The second lowest values were achieved for the FCT task, i.e., tracking at a freely chosen speed in a complex task. The highest values for Cohen’s d (effect size) statistics were obtained for the ICOb task, and then the ICOa task. The results clearly indicate the differences between the tasks for mean error and variability measures. The FP and FCT tasks were performed at a freely chosen speed. In other cases, the speed was imposed.
Table 3. Cohen’s d values presenting the effect size for differences between the elderly and young groups for each task and analysed variable.
The impact of the tracking mode on the value of the performance measures of coordination tasks in each of the three parts of the task was also analysed. In this case, the analysis was carried out in two groups: imposed and freely chosen speed. The values of the indicators for the division of tasks into groups and the three parts of the task are shown in Figure .
Figure 5. Mean and 95% confidence interval of performance measures of coordination tasks broken down by groups of elderly people and younger people, and for a group of tasks with imposed and freely chosen speed of movement of a cursor: (a) mean error, (b) variability and (c) execution time.
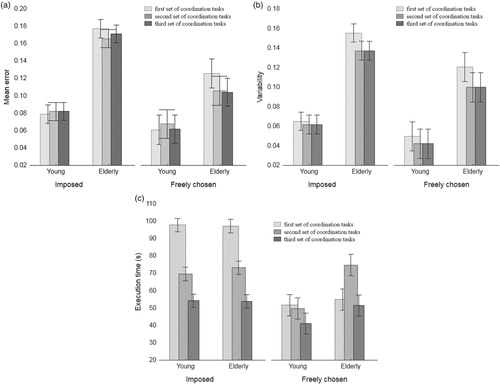
The higher values of the performance measures of coordination for the tasks with an imposed speed of movement of the cursor are clearly visible. There are also significant increases in the values of mean error and variability for elderly people compared to younger people. Higher values for the first set of tasks are also demonstrated for elderly people. Although not in all cases, the differences between the successive parts of the test are statistically significant (Table ). It is interesting that in the case of tasks with freely chosen speed of movement, the time decreased in a statistically significant manner in subsequent parts of the task. In the case of the group of elderly people, there is greater differentiation in the measurement of variability than in the measurement of mean error. The opposite holds true for younger people. In the case of tasks with freely chosen speed, much greater differentiation occurs for the elderly group in the case of both mean error and variability.
Table 4. Results of the analysis of variance Friedman test differentiating performance measures of coordination tasks between successive parts of tests.
4 Discussion
In the presented study, motor coordination was examined as the coordination error of mapping a given shape, presented on the monitor screen, by means of a control device, exerting a specific external force in order to maintain the target force. The model takes into account that visual information is particularly important for the control of isometric force. The tasks used in the study require continuous exertion of force by bimanual coordination in the directions determined by the outline shape displayed on the screen. The selection of tasks was dictated by the results of other studies indicating that the synchronization of continuous movements is linked to the activation of the corpus callosum [Citation16], which decreases with age [Citation29,Citation30], and may impact the quality of the performance of continuous bimanual coordination tasks.
The results of the presented study indicate higher values of coordination error for elderly people than for younger people in the case of all bimanual coordination tasks. This applies to both the mean error and variability. The execution time is also higher. This confirms that elderly people are less accurate when it comes to mapping a force than younger people, i.e., a high-quality performance of bimanual coordination tasks constitutes a greater challenge for elderly people compared to younger people, especially for more complex coordination tasks [Citation31,Citation32]. This is why older people have trouble not only with tasks related to work, but also with daily tasks that require complex bilateral coordination [Citation33].
In terms of performance measures of coordination tasks, significant variations were noted depending on the type of the task. A feature called the coordination mode is very important for the comparison of performance measures. The article studied various coordination modes in typical daily tasks and indicated that the basic modes of coordination, i.e., in-phase and anti-phase, are observed more frequently than complex coordination modes [Citation34]. The results of the presented study confirmed that complex tasks were a particular challenge, especially for the elderly. During complex coordination tasks, cooperation between limbs occurs at various amplitudes, directions, frequencies and/or force, which requires higher levels of motor skills and flexibility [Citation14,Citation15].
Activities during which each of the limbs steered ‘its’ cursor (ICT and FCT tasks) were examined along with activities during which a single cursor was moved, controlled by the action of two limbs (FCT and ICOb tasks). The results indicate that among the more demanding complex tasks (higher values of performance measures) there are tasks in which the movement of the cursor is controlled by simultaneous exertion of force by both upper limbs.
The greatest mean error and variability values are noted for the ICT task, namely the complex task with an imposed speed of movement, when two cursors are moving, one for each hand. At the same time, the mean error and variability for the FCT task, also a complex task but with freely chosen speed, are considerably smaller, which confirms that the tracking mode is important for the performance of tasks.
Asymmetric coordination tasks require more processing than symmetric tasks, as confirmed by this study. Two tasks were in-phase. One task was performed at a freely chosen speed (FP task) and the second at an imposed speed (IP task). The values of the performance measures were significantly lower for the FP task than for the IP task, in the case of both mean error and variability. In the case of the IP task, there are also much higher values of Cohen’s d indicator, which means that this coordination scheme is significantly more varied as regards the age factor. Therefore, it should be concluded that an imposed tracking mode increases the difficulty of the task for the elderly people.
Previous studies indicate that the in-phase coordination mode is characterized by higher stability than the anti-phase [Citation13,Citation35], irrespective of the task performed and its features. In the present study, the IAP and IP tasks differ by coordination mode and the outline shape followed by the cursor. In both cases, the task is performed at an imposed speed. The values of the performance measures, however, do not differ from one group of study subjects to another. The Cohen’s d value is also similar for both indicators. It can, therefore, be concluded that the tracking mode has a greater impact on coordination error than the performance of the tasks in-phase or anti-phase.
Task performance is also related to the speed of movement. Comparing the performance of in-phase and anti-phase tasks at different movement speeds established that elderly people obtained results similar to young people during in-phase coordination at all speeds, but only at low speeds during anti-phase coordination [Citation36]. This means that the difference in the performance of tasks resulting from age becomes more visible during the performance of tasks in anti-phase and increases as speed increases. In the presented tasks, there is no increase in the values of mean error and variability in subsequent parts of the study, both for tasks at an imposed speed and at freely chosen speed. This, however, could have been caused by the effect of learning.
Inaccuracies in the performance of activities requiring motor coordination indicate a link between the brain and the structure and functions of the muscle. Clinical and experimental data indicate that perceptual motor adaptation, including the response time, movement speed and coordination, is reduced with age [Citation6,Citation7]. The ageing process causes changes in the morphology of the human skeletal muscles.
The presented results show differences in muscle activity between the young and the elderly groups. The level of load of the elderly group was higher in the case of the biceps brachii muscle and the FC muscle, and lower in the case of the DE and TR muscles. The muscle mass, fibre composition and size all change, resulting in deterioration of their function [Citation2,Citation37], which in turn has an impact on the performance of tasks related to the neurophysiological control of the muscles. Phenomena linked to muscle structure and their load were measured using surface EMG. EMG measures neural output from the spinal cord and, thus, the number of activated motor units and their discharge rates. EMG recordings are often implemented in protocols that involve specific force profiles [Citation38] or movement trajectories [Citation39,Citation40]. Studies that compared motor unit firing characteristics between young people and elderly people revealed a lower firing rate in the elderly compared with the young [Citation41]. These differences can influence both motor performance and muscle activity. This can explain the large differences between young and elderly people in the muscle contraction level in BI and DE muscles.
A musculoskeletal load related to the task being performed can be either physical or mental, although these are always connected and cannot be completely separated when a subject performs a particular task [Citation42]. Mental demands related to the precision of task performance may also increase activity in both the neck–shoulder as well as forearm muscles. Shahidi et al. [Citation25] and Nimbarte et al. [Citation43] showed that mental load increases the activity of the trapezius muscle and is suggested to cause prolonged low-level activity of the upper trapezius muscles. Also, Visser et al. [Citation44] reported a significant increase in both neck and forearm muscle activities in the trapezius, extensor digitorum and flexor digitorum superficialis as the effect of increasing speed and mental demand in a mouse-aiming task. Taking into account the association between visual and/or attentional demands, the increased activity of the trapezius muscle [Citation45–47] and that the more demanding and complex the task, the higher the musculoskeletal load [Citation48], it should be assumed that the performed tasks were more mentally burdensome for the elderly group than for the younger group. The musculoskeletal load of the upper limbs during the performance of specific tasks resulting from the muscular contraction of the muscles involved in the exertion of force by means of a joystick and from the mental burden associated with performing activities requiring the involvement of attention varied according to the difficulty of the performed tasks. This may mean that, in terms of mental load, the tasks varied sufficiently for it to be reflected in muscle strain.
The usefulness of visual feedback on manual efficiency and musculoskeletal load caused by the performance of handling activities may vary substantially depending on the nature of the task, but also the worker’s age. Inaccuracies in the performance of activities requiring motor coordination indicate a link between the brain and the structure and functions of the muscle. At the same time, research results indicate that ageing affects perceptual motor adaptation, including the response time, movement speed and motor coordination [Citation6,Citation7]. It can, therefore, be assumed that a number of applications can be found for coordination tasks. Meanwhile, tasks testing bimanual skills can be used both for rehabilitation purposes and as indicators of neurodegenerative diseases, e.g., Parkinson’s disease [Citation49]. High-intensity, repetitive task-oriented treatment has also been found to be one of the most effective treatment methods for motor rehabilitation after stroke [Citation50]. A relatively large reduction in the value of the performance measures between the performed tasks in sets one and three of the study, especially for the elderly group, indicate the potential of these tasks to play a role in training that increases the bimanual coordination capacity in people with reduced coordination capacities. The presented motor coordination tasks seem to be appropriate for such purposes.
5 Limitations
The limitations in the present study are mainly related to the range of tests carried out, i.e., the number of study subjects and the number of examined tasks. In the study, only external indicators were analysed, which allow for the determination of the total impact of bimanual coordination tasks in the form of a functional response, but no analysis based on brain-mapping type registration was taken into account. Registration of such brain reactions would allow for the direct examination of the response of certain areas of the brain to the performance of each task, e.g., by testing the primary somatosensory cortex and cerebellum activity during performance [Citation51].
Further, another issue that influences performance and was not taken into consideration was the value of the force on the control sensors. In the case of the presented study, the value of the level of the force was 10% of the maximum force capacity. The impact of force on the increase in inaccuracy of the exerted force is an issue worthy of further exploration. Also, the number of repetitions of sets of tasks could influence performance, especially with respect to differences between performances in subsequent repetitions. The number of repetitions was restricted to three to avoid fatigue.
6 Conclusion
Among the tasks of bimanual coordination performed continuously and described by the coordination mode as in-phase, anti-phase and complex, the complex tasks showed the largest difficulty, which suggests that such tasks had the largest potential to improve coordination skills. These are the tasks during which the exercise of force by both limbs contributes to a movement of separate cursors. The tracking mode (freely chosen or imposed speeds) is crucial for the quality of the performance of motor coordination tasks. Finally, performance of tasks with imposed speed is much more age-sensitive than performance with freely chosen speed.
Disclosure statement
No potential conflict of interest was reported by the authors.
Correction Statement
This article has been republished with minor changes. These changes do not impact the academic content of the article.
References
- Tournadre A, Vial G, Capel F, et al. Sarcopenia. Joint Bone Spine. 2019;86(3):309–314. doi: 10.1016/j.jbspin.2018.08.001
- Cruz-Jentoft AJ, Baeyens JP, Bauer JM, et al. Sarcopenia: European consensus on definition and diagnosis: report of the European Working Group on Sarcopenia in Older People. Age Ageing. 2010;39(4):412–423. Epub 2010 Apr 13. doi: 10.1093/ageing/afq034
- Kim M, Won C. Sarcopenia is associated with cognitive impairment mainly due to slow gait speed: results from the Korean Frailty and Aging Cohort Study (KFACS). Int J Environ Res Public Health. 2019;16:1491. doi: 10.3390/ijerph16091491
- Granacher U, Bridenbaugh SA, Muehlbauer T, et al. Age-related effects on postural control under multi-task conditions. Gerontology. 2011;57(3):247–255. Epub 2010 Oct 27. doi: 10.1159/000322196
- Seidler RD, Bernard JA, Burutolu TB, et al. Motor control and aging: links to age-related brain structural, functional and biochemical effects. Neurosci Biobehav Rev. 2010;34:721–733. doi: 10.1016/j.neubiorev.2009.10.005
- Arnold P, Vantieghem S, Gorus E, et al. Age-related differences in muscle recruitment and reaction-time performance. Exp Gerontol. 2015;70:125–130. doi: 10.1016/j.exger.2015.08.005
- van Halewyck F, Lavrysen A, Levin O, et al. Both age and physical activity level impact on eye–hand coordination. Hum Movement Sci. 2014;36:80–96. doi: 10.1016/j.humov.2014.05.005
- Lin C-H, Chou L-W, Wei S-H, et al. Influence of aging on bimanual coordination control. Exp Gerontol. 2014;53:40–47. Epub 2014 Feb 15. doi: 10.1016/j.exger.2014.02.005
- Addamo PK, Farrow M, Bradshaw JL, et al. The effect of attending to motor overflow on its voluntary inhibition in young and older adults. Brain Cogn. 2010;74(3):358–364. Epub 2010 Oct 27. doi: 10.1016/j.bandc.2010.10.001
- Hu X, Newell KM. Aging, visual information, and adaptation to task asymmetry in bimanual force coordination. J Appl Physiol. 2011;111(6):1671–1680. Epub 2011 Sep 29. doi: 10.1152/japplphysiol.00760.2011
- Seidler RD, Alberts JL, Stelmach GE. Changes in multi-joint performance with age. Motor Control. 2002;6(1):19–31. doi: 10.1123/mcj.6.1.19
- Serrien DJ, Swinnen SP, Stelmach GE. Age-related deterioration of coordinated interlimb behavior. J Gerontol. 2000;55(5):295–303. doi: 10.1093/geronb/55.5.P295
- Bangert AS, Reuter-Lorenz PA, Walsh CM, et al. Bimanual coordination and aging: neurobehavioral implications. Neupsychol. 2010;48(4):1165–1170. doi: 10.1016/j.neuropsychologia.2009.11.013
- Mechsner F, Kerzel D, Knoblich G, et al. Perceptual basis of bimanual coordination. Nature. 2001;414:69–73. doi: 10.1038/35102060
- White O, Diedrichsen J. Responsibility assignment in redundant systems. Curr Biol. 2010;20(14):1290–1295. doi: 10.1016/j.cub.2010.05.069
- Kennerley SW, Diedrichsen J, Hazeltine E, et al. Callosotomy patients exhibit temporal uncoupling during continuous bimanual movements. Nat Neurosci. 2002;5(4):376–381. doi: 10.1038/nn822
- Dragioti E, Gerdle B, Larsson B. Longitudinal associations between anatomical regions of pain and work conditions: a study from The SwePain Cohort. Int J Environ Res Public Health. 2019;16:2167. doi: 10.3390/ijerph16122167
- Alonso JF, Mañanas MA, Rojas M, et al. Coordination of respiratory muscles assessed by means of nonlinear forecasting of demodulated myographic signals. J Electromyogr Kines. 2011;21(6):1064–1073. doi: 10.1016/j.jelekin.2011.07.004
- Hutten GJ, van Thuijl HF, van Bellegem AC, et al. A literature review of the methodology of EMG recordings of the diaphragm. J Electromyogr Kines. 2010;20(2):185–190. Epub 2009 May 6. doi: 10.1016/j.jelekin.2009.02.008
- Ting LH, McKay JL. Neuromechanics of muscle synergies for posture and movement. Curr Opin Neurobiol. 2007;17:622–628. doi: 10.1016/j.conb.2008.01.002
- Szeto GP, Straker LM, O’Sullivan PB. A comparison of symptomatic and asymptomatic office workers performing monotonous keyboard work – 2, neck and shoulder kinematics. Man Ther. 2005;10(4):281–291. doi: 10.1016/j.math.2005.01.005
- Szeto GPY, Lin JKM. A study of forearm muscle activity and wrist kinematics in symptomatic office workers performing mouse-clicking tasks with different precision and speed demands. J Electromyogr Kines. 2011;21(1):59–66. doi: 10.1016/j.jelekin.2010.06.006
- Wixted F, O'Riordan C, O'Sullivan L. Inhibiting the physiological stress effects of a sustained attention task on shoulder muscle activity. Int J Environ Res Public Health. 2018;15(1):115. doi: 10.3390/ijerph15010115
- Stephenson JL, Christou EA, Maluf KS. Discharge rate modulation of trapezius motor units differs for voluntary contractions and instructed muscle rest. Exp Brain Res. 2011;208(2):203–215. doi: 10.1007/s00221-010-2471-4
- Shahidi B, Haight A, Maluf K. Differential effects of mental concentration and acute psychosocial stress on cervical muscle activity and posture. J Electromyogr Kinesiol. 2013;23:1082–1089. doi: 10.1016/j.jelekin.2013.05.009
- Krehbiel LM, Kang N, Cauraugh JH. Age-related differences in bimanual movements: a systematic review and meta-analysis. Exp Gerontol. 2017;98:199–206. doi: 10.1016/j.exger.2017.09.001
- Hermens HJ, Freriks B, Disselhorst-Klug C, et al. Development of recommendations for SEMG sensors and sensor placement procedures. J Electromyogr Kinesiol. 2000;10(5):361–374. doi: 10.1016/S1050-6411(00)00027-4
- Lipsey M, Wilson D. Practical meta-analysis. Thousand Oaks (CA): Sage; 2001.
- Sullivan EV, Pfefferbaum A, Adalsteinsson E, et al. Differential rates of regional brain change in callosal and ventricular size: a 4-year longitudinal MRI study of elderly men. Cereb Cortex . 2002;12(4):438–445. doi: 10.1093/cercor/12.4.438
- Driesen NR, Raz N. The influence of sex, age, and handedness on corpus callosum morphology: a meta-analysis. Psychobiology. 1995;23(3):240–247. doi: 10.3758/BF03332028
- Aoki T, Fukuoka Y. Finger tapping ability in healthy elderly and young adults. Med Sci Sport Exer. 2010;42(3):449–455. doi: 10.1249/MSS.0b013e3181b7f3e1
- Loehrer PA, Nettersheim FS, Jung F, et al. Ageing changes effective connectivity of motor networks during bimanual finger coordination. Neuroimage. 2016;143:325–342. doi: 10.1016/j.neuroimage.2016.09.014
- Maes C, Gooijers J, Orban de Xivry JJ, et al. Two hands, one brain, and aging. Neurosci Biobehav Rev. 2017;75:234–256. Epub 2017 Feb 8. doi: 10.1016/j.neubiorev.2017.01.052
- Howard IS, Ingram NJ, Körding PK, et al. Statistics of natural movements are reflected in motor errors. J Neurophysiol. 2009;102(3):1902–1910. doi: 10.1152/jn.00013.2009
- Temprado JJ, Zanone PG, Monno A, et al. Attentional load associated with performing and stabilizing preferred bimanual patterns. J Exp Psychol Human Percept Perform. 1999;25:1579–1594. doi: 10.1037/0096-1523.25.6.1579
- Lee TD, Wishart LR, Murdoch JE. Aging, attention, and bimanual coordination. Can J Aging. 2002;21(4):549–557. doi: 10.1017/S0714980800002087
- Rosenberg IH. Sarcopenia: origins and clinical relevance. Clin Geriatr Med. 2011;27(3):337–339. doi: 10.1016/j.cger.2011.03.003
- Roh J, Rymer WZ, Perreault EJ, et al. Alterations in upper limb muscle synergy structure in chronic stroke survivors. J Neurophys. 2013;109(3):768–781. doi: 10.1152/jn.00670.2012
- Rasool G, Afsharipour B, Suresh N, et al. Spatial analysis of multichannel surface EMG in hemiplegic stroke. IEEE Trans Neural Syst Rehabil Eng. 2017;25(10):1802–1811. doi: 10.1109/TNSRE.2017.2682298
- Sarcher A, Raison M, Leboeuf F, et al. Pathological and physiological muscle co-activation during active elbow extension in children with unilateral cerebral palsy. Clin Neurophysiol. 2017;128(1):4–13. doi: 10.1016/j.clinph.2016.10.086
- Piasecki M, Ireland A, Stashuk D, et al. Age-related neuromuscular changes affecting human vastus lateralis. J Physiol. 2016;594(16):4525–4536. doi: 10.1113/JP271087
- Lean Y, Shan F. Brief review on physiological and biochemical evaluations of human mental workload. Hum Factors Ergon Manuf Serv Ind. 2012;22:177–187. doi: 10.1002/hfm.20269
- Nimbarte AD, Al Hassan MJ, Guffey SE, et al. Influence of psychosocial stress and personality type on the biomechanical loading of neck and shoulder muscles. Int J Ind Ergon. 2012;42:397–405. doi: 10.1016/j.ergon.2012.05.001
- Visser B, De Looze M, De Graaff M, et al. Effects of precision demands and mental pressure on muscle activation and hand forces in computer mouse tasks. Ergonomics. 2004;47(2):202–217. doi: 10.1080/00140130310001617967
- Richter HO, Zetterberg C, Forsman M. Trapezius muscle activity increases during near work activity regardless of accommodation/vergence demand level. Eur J Appl Physio. 2015;115:1501–1512. doi: 10.1007/s00421-015-3125-9
- Zetterberg C, Forsman M, Richter HO. Effects of visually demanding near work on trapezius muscle activity. J Electromyogr Kines. 2013;23:1190–1198. doi: 10.1016/j.jelekin.2013.06.003
- Mehta RK, Agnew MJ. Influence of mental workload on muscle endurance, fatigue, and recovery during intermittent static work. Eur J Appl Physio. 2012;112:2891–2902. doi: 10.1007/s00421-011-2264-x
- Vidulich MA, Tsang PS. The confluence of situation awareness and mental workload for adaptable human–machine systems. J Cogn Eng Decis Mak. 2014;9(1):95–97.
- Vercruysse S, Spildooren J, Heremans E, et al. The neural correlates of upper limb motor blocks in Parkinson's disease and their relation to freezing of gait. Cereb Cortex . 2014;24:3154–3166. doi: 10.1093/cercor/bht170
- Song GB. The effects of task-oriented versus repetitive bilateral arm training on upper limb function and activities of daily living in stroke patients. J Phys Therapy Sci. 2015;27:1353–1355. doi: 10.1589/jpts.27.1353
- Riecker A, Kassubek J, Groschel K, et al. The cerebral control of speech tempo, opposite relationship between speaking rate and BOLD signal changes at striatal and cerebellar structures. Neuro Image. 2006;29:46–53.